Chapter 15 Radiography and Radiology
Radiographic Detail
The aim is to obtain as highly detailed radiographic images as possible. The detail that can be obtained is influenced by a number of factors, including those listed in Box 15-1.
BOX 15-1 Factors That Influence Radiographic Images
Radiation Safety
Radiation safety (i.e., ensuring that personnel around the horse do not receive doses of radiation) is essential. Different codes of practice apply in various countries, but the basic principles are summarized in Box 15-2.
BOX 15-2 Basic Principles of Radiation Safety
Response of Bone to Stimuli: Wolff’s Law
Periosteal New Bone
Blunt trauma to bone can lead to subperiosteal hemorrhage, resulting in lifting of the periosteum away from the bone. This process may stimulate the production of periosteal new bone (Figure 15-1). Some bones, such as the second and fourth metacarpal and metatarsal bones, seem particularly prone to such reactions. There is individual variation among horses in susceptibility to such reactions. Usually a lag period of at least 14 days occurs between trauma and the radiological detection of periosteal new bone. Such bone usually is much less dense than the parent bone; therefore soft exposures (or low kilovoltages) are essential for detection of this bone, which initially has a rather irregular outline. As the bone gradually consolidates and then models, it becomes more opaque and more smoothly outlined. Curiously, although a well-established splint exostosis would be expected to become inactive, many have increased radiopharmaceutical uptake (IRU) compared with the parent bone if examined scintigraphically.
Endosteal New Bone
Endosteal new bone may develop as a result of trauma (e.g., a cortical or subcortical fracture or trauma at an enthesis) or inflammation, infection, or, less commonly, a tumor (Figure 15-2). Stress fractures of the dorsal cortex of the McIII are accompanied by the development of endosteal new bone, which may be more readily detected than the fracture itself.
Sclerosis
Sclerosis is the localized formation of new bone within bone and results in increased bone mass. It is most easily identified in trabecular bone (Figure 15-3) and occurs in response to several stimuli, including the following:
Osteophyte Formation
Some joints seem to have a greater propensity than others for the development of periarticular osteophyte formation. The reason for this tendency is unknown and may in part reflect the ease with which osteophytes can be detected radiologically. Even within what is currently considered a single disease process, osteoarthritis of the distal hock joints (bone spavin), some horses develop predominantly periarticular osteophytes (Figure 15-4), whereas others have narrowing of the joint space and subchondral sclerosis. A third group develops extensive radiolucent areas (Figure 15-5).
Enthesophyte Formation
Enthesophyte formation is new bone at the site of attachment of a tendon, ligament, or joint capsule to bone. Entheseous new bone reflects the bone’s response to stress applied through these structures, such as ligamentous tearing or capsular traction (Figure 15-6
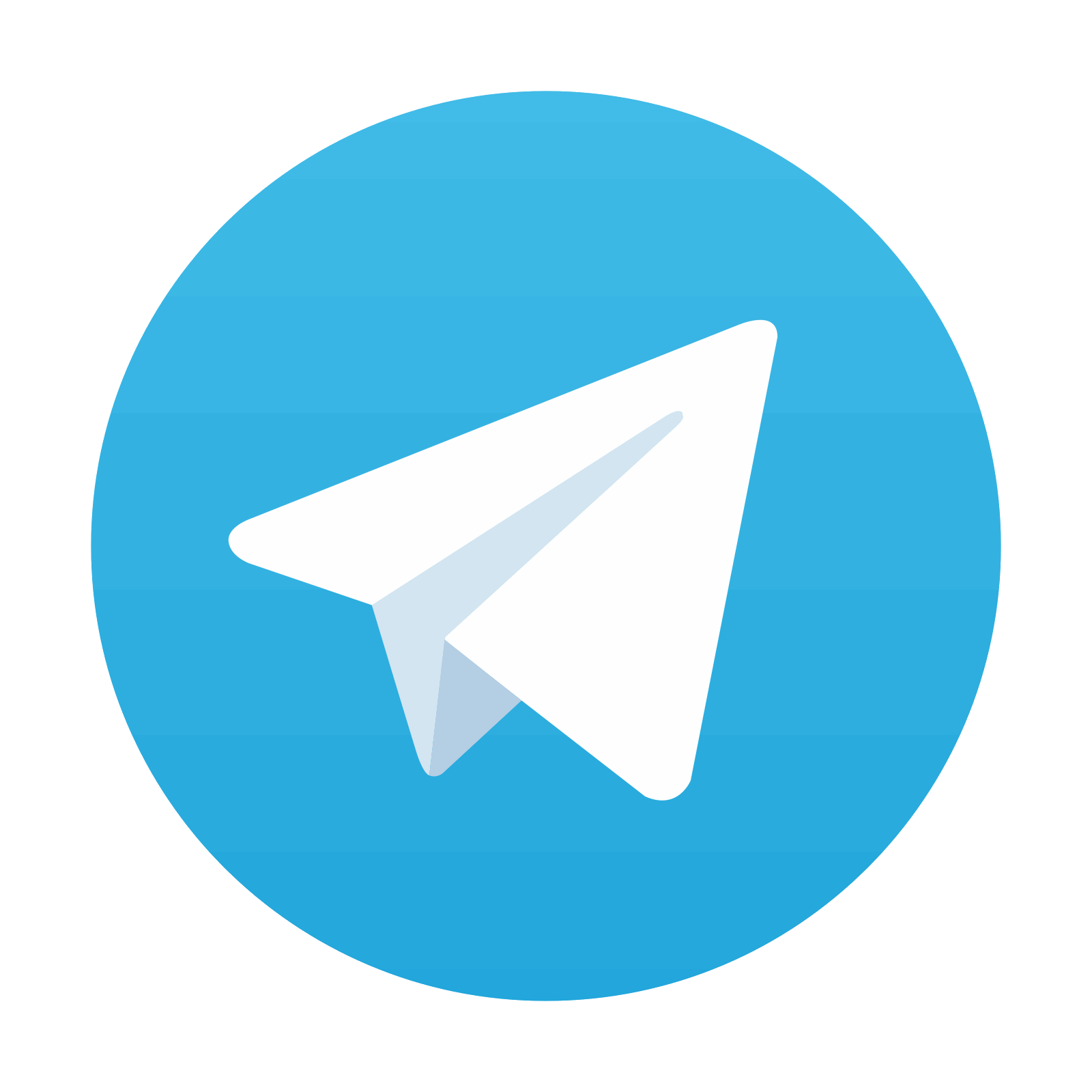
Stay updated, free articles. Join our Telegram channel

Full access? Get Clinical Tree
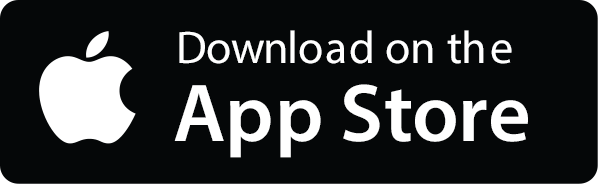
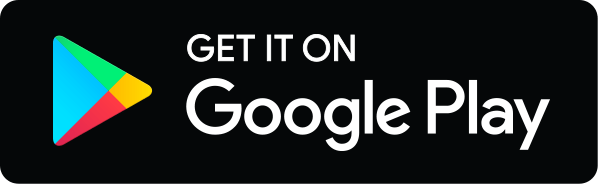