Chapter 15. Radiation Therapy
SECTION A External Beam Radiation Therapy (Teletherapy) and Brachytherapy
Jimmy C. Lattimer and David A. Bommarito
HISTORICAL PERSPECTIVE
Radiation in the form of an external beam has been used for many years in the management of solid cancers. Beginning in the 1930s, orthovoltage x-ray machines, which have an energy range of 150 to 500 kVp, were used for therapy. These units were largely replaced with higher energy Cobalt 60 and Cesium 137 machines following the development of the nuclear reactor in the late 1940s and 1950s. These sources have energies of 1.25 Mev and 0.662 Mev, respectively, based on the radioactive core contained within the machine. They became popular replacements to orthovoltage units because of their simplicity, stability, and, most importantly, ability to penetrate deeper tissues because of their higher energies. Today, Cobalt 60 and Cesium 137 units are falling out of favor because of concerns and liability related to replacement of the radioactive source, as well as modern-day issues of security and public safety. For these reasons, linear accelerator machines, which produce 4 to 15 megavolt x-ray beams, have largely replaced isotope machines.
LINEAR ACCELERATORS
Linear accelerators (LINAC) used in radiation therapy today are compact and highly reliable machines that, when integrated with computer control systems, can deliver radiation therapy (photon) beams at a constant dose rate and with a high degree of precision and repeatability. These factors are increasingly important in veterinary oncology with the advent of more complex approaches to radiation therapy planning for pets with cancer. In addition, many linear accelerators are configured to also deliver electron beams that are used to treat skin and superficial tumors. When considering patient referral for radiation therapy, practitioners should seek treatment sites with equipment best suited to provide the needs for each individual patient. While electron capabilities may not be important for the treatment of many deep-seated tumors, they provide an advantage when treating superficial or cutaneous lesions.
TELETHERAPY
Teletherapy refers to the use of an external beam for radiation treatment, as is done with linear accelerators and Cobalt 60 or Cesium 137 machines. Typical tumors treated this way include, but are not limited to, nasal carcinomas and sarcomas, brain tumors, oral neoplasms, isolated lymphoma, adenocarcinoma of the salivary gland and thyroid, mediastinal tumors, spinal column tumors, and malignancies of the pelvic canal. External beam radiation is also used to treat skin, subcutaneous tissue, and muscle after incomplete surgical resection of a tumor. Radiation therapy may be used to treat tumors in sites such as the distal limb, where surgical removal would require amputation or severely disfiguring surgery and for which there is a reasonable probability of controlling the neoplasm using radiation therapy alone or in combination with chemotherapy. Mast cell tumors and solitary cutaneous lymphoma are examples. External beam radiation therapy is also used for palliative treatment of tumors such as osteosarcoma of the limb to control pain when amputation is not a viable option.
External beam radiation therapy protocols are developed such that the total dose of radiation is given in a number of treatments referred to as fractions. This is done to maximize the killing effect on the tumor, while minimizing deleterious effects on the normal tissues. This is particularly important for normal tissues that do not have a large degree of cell turnover, such as muscle and bone. 1 The slow turnover of some normal tissues is protective against radiation damage if fractionation is used, because tissues are less sensitive to radiation damage if they are not actively dividing. This protective effect is negated, however, if radiation is delivered as a single large fraction, because large doses are damaging to both replicating and non-replicating tissues. Fractionation of the treatment over time allows for reassortment of tumor cells within the cell cycle, which improves the likelihood that each cell will be irradiated during the more sensitive phase of the cell cycle. Fractionation also allows for the return of hypoxic cells in the tumor population to a more oxic state as the radiation kills the more sensitive oxic cells between the hypoxic cells and the blood supply. This allows these cells to be irradiated in a more sensitive oxic state. Fractionation also allows time for normal cells killed by radiation to be repopulated from surviving cells. This also happpens in tumors but to a lesser degree. Normal cells are also more efficient at repairing radiation injury to the DNA when it is delivered in multiple small doses rather than in a single large dose. Tumor cells are generally not as good at this as normal cells in the dose range for most fractionation protocols. Taken together, these principles are referred to as the 4R’s of radiation therapy—reassortment, reoxygenation, repopulation, repair—and form the foundation for modern radiation therapy protocols. Modern, definitive (intent to cure) veterinary radiation therapy protocols typically call for the administration of the total radiation dose over 10 to 20 daily fractions in 2 to 4 weeks, although many variations exist to meet individual circumstances. Veterinary radiation therapy requires anesthesia of the patient, not because there is any pain associated with the treatment, but in order to ensure they are immobilized and radiation is delivered accurately to the tumor site. This requirement for anesthesia is the major obstacle to using protocols similar to those used in human radiation oncology, where the total dose of radiation is typically divided into 30 to 40 fractions. Therefore, the total dose of radiation delivered to veterinary patients is often somewhat lower and in larger fractions than that administered to people for tumors of similar types. Typical fraction sizes used in veterinary radiation therapy are in the 2.5 to 4 Gray (1 Gy = 100 rads) range when treating with curative intent. This represents a trade-off between using ideal radiobiological principles and the practicality related to the requirement for anesthesia, protracted hospitalization, cost, and owner acceptance.
STEREOTACTIC RADIOSURGERY/“GAMMA KNIFE” THERAPY
Stereotactic radiosurgery is an advanced form of radiation therapy used to treat small lesions (<2 cm) that are not surgically treatable (e.g., brain stem tumors). Between one and a few large doses are used, so extremely fine control is needed to avoid lethally damaging normal structures. Experience, computerized planning, and special positioning and beam limiting devices are required. Very few veterinary facilities have the expertise required to perform this technique.
THERAPY PLANNING
Within the last 10 years, computers have markedly changed many of the concepts related to radiation therapy planning. Previously, simple plans used one or two beams of radiation, and planning and dosimetry calculations were done by hand. Now it is common practice to use sophisticated computer plans that use data derived from computed tomography (CT) and the therapy machine’s dosimetry tables to provide a 3D map of the radiation dose delivery to the tumor and the surrounding tissues ( Figure 15-1 ). These programs allow prescription of radiation therapy plans that use more beams than were typically used in the past. By doing this, the radiation oncologist is able to conform the region of high radiation dose to the shape of the tumor and to shape the treatment field in ways that prevent a high radiation dose from being delivered to critical normal structures such as the optic nerve or spinal cord. This approach permits the delivery of higher radiation doses to tumors while minimizing acute local reactions such as moist desquamation or mucositis, and chronic effects such as bone necrosis or strictures, all of which are discussed in Section C . 2,3
![]() |
FIGURE 15-1 Computer-aided treatment plans used in many veterinary radiation treatment facilities today facilitate the use of CT images (A) to create treatment plans that maximize the dose to tumor tissues while sparing normal tissues. Here, plans for a dog with nasal carcinoma (B) and a dog with a maxillary mass (C) are shown. Tumor tissue, shown in red, receives the maximum dose, whereas eyes and normal surrounding tissues receive a smaller dose. |
PATIENT SET-UP AND POSITIONING
When planning a course of radiation therapy, the radiotherapist must first define the location of the tumor to be treated. Tumor margins and the location of associated vital structures are typically determined using contrast CT scans or MRI. When treatment plans are based on CT images, it is vitally important to reproduce the positioning from the CT scan to the treatment table as accurately as possible. Many different positioning systems have been used, with the simplest such systems using various rigid or semi-rigid foam pads of various shapes as well as sandbags and tape to position the animal. Using vacuum cradles or custom cast foam cradles can improve the accuracy of positioning, especially for the head. The addition of a mouth block incorporating a dental mold has been shown to further improve the positioning accuracy to ±0.1 cm under optimum conditions. 4 In general, accurate positioning of the head is easier than for other anatomic sites because of the more prominent bony landmarks and rigid nature of the skull. Consequently, larger volumes of normal tissue are often incorporated into the treatment volume in other parts of the body to ensure the inclusion of the entire tumor. 5 This inherently increases the likelihood of damage to normal surrounding tissues. Minimizing patient positioning errors is of paramount importance in not only maximizing tumor control, but in preventing post-irradiation complications.
SKIN-SPARING EFFECTS
Linear accelerators produce beams that are highly penetrating. Although the physics of high-energy x-rays is complex, once the effective energy of the beam exceeds approximately 500 keV, the maximum dose of radiation delivered by the beam (also called Dmax) to the tissue no longer occurs at the surface of the skin. This effect increases with energy to the point that for a 10-MeV beam, the Dmax is approximately 3 cm below the skin surface and the dose to the skin is approximately 30% of the dose at Dmax. This marked “skin sparing” effect is advantageous for treatment of deep tumors within the body. Once the dose maximum is reached, the intensity of the beam decreases relatively slowly beyond that point. Because of this, the dose to the skin on the side where the beam exits is substantially higher than that on the side of entry. This may be counterintuitive to clients, and they may question why skin effects would occur on the side opposite a treated lesion. Thus, pretreatment client consultation to explain potential side effects is vitally important.
Many beam-modifying devices can be used to conform the radiation dose to specific anatomical variations noted in small animal oncology patients. These include blocks, cutouts, filters, and wedges that are beyond the scope of this discussion but are shown in Figure 15-2 .
![]() |
FIGURE 15-2 Treatment aids used in megavoltage radiation therapy. Wedges (A) help to provide uniform depth dose when the field is incident on an angled field. Custom blocks (B) shield vital normal structures. Bolus (C) brings the Dmax closer to the surface of the skin. |
As mentioned previously, electron beams may be used instead of photons for superficial and cutaneous tumors. Electrons, because of their charge and mass, lose energy very quickly once they enter the body. Consequently, the rate of dose fall-off after Dmax is much greater for electrons than for x-ray (photon) beams. In practical terms, this means that a therapeutic dose is delivered to the superficial tissues, while underlying tissues are spared. Thus, electron beam therapy is ideal for treatment of many cutaneous and superficial masses, especially those that are overlying critical structures.
PALLIATIVE RADIATION
Some neoplasms, because of their biology and other factors, such as the presence or likelihood of distant metastasis, are unlikely to be cured by radiation therapy. Osteosarcoma is a prime example of such a tumor. 6 In these instances, treatment with “palliative intent” may be the best choice. Palliative intent treatments typically involve treatment with one to five large fractions of 6 to 10 Gray administered at weekly or longer intervals. Palliative intent treatments are given in order to slow tumor progression and, most importantly, decrease pain associated with the tumor. Radiation oncologists vary widely in their approach to palliative treatments, and there is no uniform recommendation with regard to the number or size of fractions for any given tumor. When recommending palliative treatment, it is important to be cognizant of the type of normal tissue complications that can arise, since large fractions are more likely to have severe late effects on normal tissue, such as bone necrosis, than are small fractions. The total dose of radiation that can be given is also less than would be the case for smaller fractions given over the same period. As a result, ultimate control of the tumor is less likely. Treatment with a larger number of smaller fractions will typically result in fewer complications and more durable tumor control and should be recommended when all other factors are equal. However, many mitigating factors including client preference and patient life expectancy may make palliative treatment the better choice in some cases.
BRACHYTHERAPY
Brachytherapy (from the Greek brachy , meaning short distance) consists of placing radioactive sources in or near the target tissue. The dose rate from these sources falls exponentially with distance, allowing for the delivery of very high doses to the tumor and much lower doses to the normal surrounding tissues. Brachytherapy has been limited in veterinary medicine by concerns about radioactive exposure to clinicians and hospital staff, the potential for lost or ingested radioactive material, and the lack of appropriate radiation isolation facilities. Despite these issues, various animal malignancies have been successfully treated using brachytherapy. Brachytherapy can be delivered through several different methods, each having distinct advantages and disadvantages. The choice of brachytherapy method depends on shape, size, and location of the target tissue. Examples of brachytherapy techniques used in veterinary medicine include the following:
1. Interstitial brachytherapy refers to the surgical placement of radioactive implants directly inside the target tissue. An example is interstitial iridium 192, which has been used with some success after surgical removal of canine mast cell tumors. 7
2. Intracavitary brachytherapy refers to the placement of radioactive implants inside a body cavity in close proximity to the target tissue. Intranasal iridium implants have been used to treat dogs after surgical debulking of nasal cavity malignancies. 8
Plesiotherapy involves direct application of a radioactive source onto the target tissue. Strontium 90 probes ( Figure 15-3 ) are the most common application of this type of therapy in veterinary medicine and have been used with great success to treat very superficial lesions. Strontium is a beta emitter, and almost the entire radiation dose is deposited superfi cially, with only about 5% going beyond 4 mm. Cats with superfi cial nasal planum squamous cell carcinoma treated with a single dose of strontium plesiotherapy had a 98% response rate, with 88% of these having complete resolution of their lesions. 9 Feline mast cell tumors have also been successfully treated with strontium. One report cited a 98% local control rate after single or multiple applications. 10
< div class='tao-gold-member'>
Only gold members can continue reading. Log In or Register a > to continue
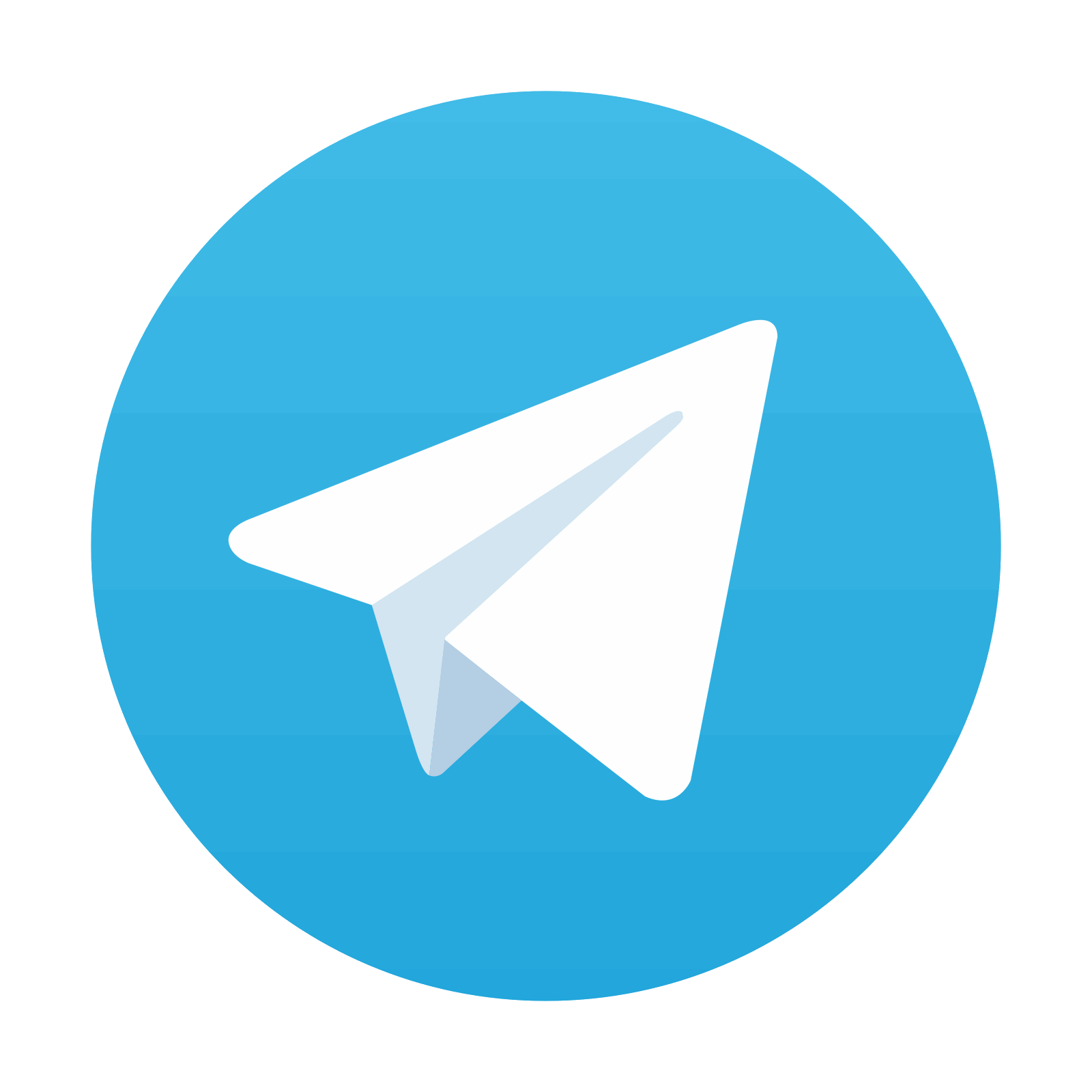
Stay updated, free articles. Join our Telegram channel

Full access? Get Clinical Tree
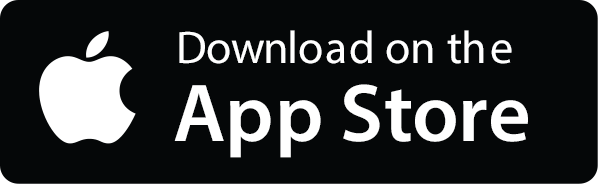
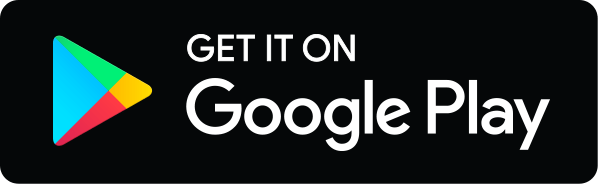