CHAPTER 50 Purine Uroliths
WHAT ARE PURINES?
Purines are heterocyclic aromatic nitrogen-containing bases that form the building blocks of nucleic acid. Purines are a six-membered pyrimidine ring fused to five-membered imidazole rings (Figure 50-1). Naturally occurring purines belong to three major classes of purine compounds: aminopurines (adenine and guanine), oxypurines (hypoxanthine, xanthine, uric acid, and allantoin), and methylpurines (caffeine, theophylline, and theobromine).1
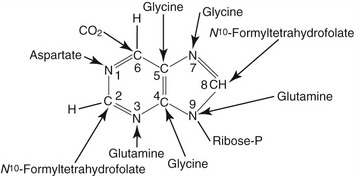
Figure 50-1 Structure of the purine and sources of atom in the purine rings during de novo synthesis are indicated.7,42 The atoms are numbered according to the international system.
Animal proteins and purine-rich diets are the major sources of exogenous purines for mammals. In addition to the exogenous sources, purines also are synthesized de novo in the liver from nonpurine precursors. During de novo synthesis of purines, molecules such as glycine, aspartate, glutamine, N10-formyltetrahydrofolate, carbon dioxide, glucose, and adenosine triphosphate (ATP) contribute to the formation of the purine ring (see Figure 50-1).2,3
Purine bases also can be salvaged and recycled endogenously from degrading nucleic acids. Preformed free purines that are derived from diet and nucleic acid degradation react with 5-phosphoribosyl-1-pyrophosphate (PRPP) in a reaction catalyzed by phosphoribosyltransferases (adenine phosphoribosyltransferase or hypoxanthine-guanine phosphoribosyltransferase) and form adenosine monophosphate (AMP), guanosine monophosphate (GMP), and inosine monophosphate (IMP). The detailed purine metabolic pathway is illustrated in Figures 50-2 and 50-3.2,4,5
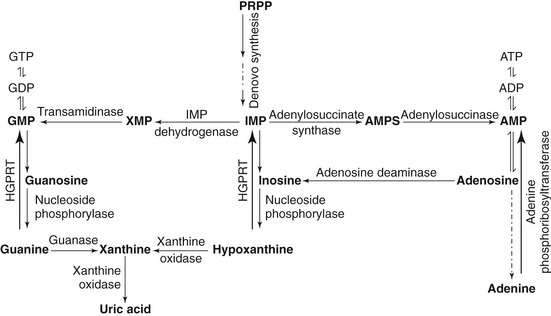
Figure 50-2 Schematic diagram of purine metabolic pathway.7,42,49 During de novo purine synthesis, bases are constructed on 5-phosphoribosyl-1-pyrophosphate (PRPP) in multiple steps. Hypoxanthine-guanine phosphoribosyltransferase (HGPRT) is a crucial enzyme involved in the salvage pathway.7,42,49 IMP, Inosine monophosphate; AMP, adenosine monophosphate; GMP, guanosine monophosphate.
WHAT IS URIC ACID?
Uric acid is an organic intermediate metabolite that arises in the purine metabolic pathway. Xanthine oxidase catalyzes the final step in uric acid synthesis. In most mammals uric acid is metabolized further into soluble allantoin. The degradation of uric acid is initiated by urate oxidase (uricase). The first intermediary metabolite of oxidative conversion of uric acid is 5-hydroxy isourate (HIU). HIU hydrolase catalyzes the hydrolysis of HIU to form 2-oxo-4-hydroxy-4-carboxy-5-ureidoimidazoline (OHCU).4 OHCU is decarboxylated by OHCU decarboxylase into dextrorotatory allantoin (S-(+)-allantoin)4 (see Figure 50-3). However, in human beings and primates the uric acid is the end product of purine catabolism.
In serum at physiological pH, uric acid is circulated in the form of monosodium urate. In mammals renal excretion is the major route of uric acid excretion although residual amounts of uric acid also are eliminated via the intestine. During renal clearance blood urate is filtered completely through the glomerulus, followed by reabsorption of the majority (≈90 per cent) of urates by the proximal tubules back into the circulation. Urate transporters in epithelia of the proximal tubules are essential for urate reabsorption (see the following).6 The reabsorbed uric acid is metabolized further in liver, with a fraction that is not catabolized going through the glomerular filtration-reabsorption cycle.7 This process leaves only 10 per cent of the plasma urate for excretion in urine.8
WHAT ARE THE PATHOPHYSIOLOGICAL METHODS OF URATE UROLITH FORMATION?
Supersaturation of uric acid in urine is essential for urate urolithiasis. Excess production, increased renal excretion, increased renal secretion, and decreased renal tubular reabsorption of uric acid promote hyperuricosuria. However, studies in human beings and dogs have demonstrated that urine supersaturation of uric acid alone is not sufficient to cause urate stones.1 In addition, other physiochemical characteristics of the urine, such as pH, urine volume, cation concentration, and macromolecules, also play a critical role in regulating urate stone formation.
Molecular mechanisms of urate urolithiasis in cats have not been identified clearly. Renal tubular reabsorptive defect and portal vascular anomalies are the two postulated mechanisms thought to be involved in the pathogenesis of urate urolithiasis in this species.7 However, no systematic studies have been carried out to prove the aforementioned hypotheses. Analyzing the comparative pathophysiological mechanisms involved in urate stone formation in human beings, dogs, and cats will provide the preliminary information to design systematic explorative studies in cats.
Again, uric acid is generated from purine catabolism, which takes place in the liver (see Figure 50-2). The final step in uric acid synthesis is catalyzed by xanthine oxidase. Although uric acid metabolism takes place primarily in the liver,9 in human beings xanthine oxidase also is expressed in the jejunum. In cats and dogs the tissue distribution of xanthine oxidase is not known. Interestingly, human beings lack urate oxidase activity in the liver, because the urate oxidase gene was lost in primate ancestors about 15 million years ago. Therefore uric acid is the end product of purine catabolism in human beings.10,11
Comparative analysis of plasma concentration of urate in cats and Dalmatian dogs suggests that the urate oxidase enzyme is present in cats.12,13 Evidence of urate oxidase in the peroxisomes of hepatic parenchymal cells in cats14 may account for the relatively low prevalence of urate uroliths in this species. It would be worthwhile to investigate the urate oxidase activity in cats with urate stones to determine if stone-forming cats possess a functional urate oxidase.
Dalmatian dogs excrete mainly uric acid in their urine and are highly likely to develop hyperuricosuria. In the Dalmatian dog it is believed that hyperuricosuria is inherited as an autosomal recessive condition and regulated by a single gene.15 Phylogenetic genome comparison and other studies indicate that urate oxidase is expressed in dogs.4 In addition, Safra et al revealed that the urate oxidase gene is not responsible for the hyperuricosuria phenotype in Dalmatian dogs, based on the cDNA sequence identity and negative LOD scores.15 However, uric acid levels are high in serum and urine of Dalmatian dogs compared with other breeds. It also has been shown that both stone-forming and non–stone-forming Dalmatian dogs have comparable levels of uric acid in plasma.16,17 Liver and kidney transplantation studies in Dalmatian dogs have enabled investigators to localize the cause of hyperuricosuria to the liver.18–23 Before this study, it was believed that Dalmatian dogs had a defect in the mechanism of urate reabsorption at the proximal tubules.24,25 Simkin postulates that normal dogs (non–stone-formers) express promoters that facilitate the uric acid transport across the hepatocyte and proximal tubular cells, although no studies have been carried out to test this hypothesis.26
However, in vitro studies revealed that functional urate oxidase is present in liver homogenates of Dalmatian dogs. In contrast, liver slices from Dalmatian dogs were unable to metabolize uric acid.27 The findings of these in vitro studies enabled the investigators to localize the cause of defective urate metabolism specifically to the membrane transporters in hepatocytes. Further investigations attempted to identify specific urate transporters in hepatocytes. Galectin-9, one of the putative urate transporters, was found to have no role in hyperuricosuria.28 Reports on other urate transporters and their role in hyperuricosuria in Dalmatian dogs are not available. However, Safra et al have shown that the locus CFA03 in the canine genome is linked to hyperuricosuria in Dalmatian dogs.29 Surprisingly, none of the candidate genes of this locus are known to be associated with purine metabolism or urate transport.29 However, follow-up studies on the other candidate genes of locus CFA03 have not been published to date.
In non-Dalmatian dogs, urate stone formation often is found in conjunction with a portal vascular anomaly.30,31 In portovascular shunts, liver functions are impaired significantly, resulting in inefficient hepatic conversion of ammonia to urea and uric acid to allantoin.30
The cellular mechanisms involved in hyperuricemia and hyperuricosuria have not been investigated in cats. In light of the findings from other species, it is likely that a defective purine metabolism is one of the causes. It is possible that consumption of a purine-rich diet and increased nucleic acid catabolism as a result of extensive tissue damage or tissue proliferation (for example, lymphoma or leukemia) will lead to hyperuricemia and hyperuricosuria by simply increasing the purine pool of the body.1 This leads to the hypothesis that the feline urate oxidase is unable to cope with a high load of uric acid generated from a high purine pool.
Reports indicate that a hepatic portal vascular anomaly also could contribute to hyperuricemia and hyperuricosuria in cats.32 Because purine metabolism primarily is taking place in the liver, purine metabolism also would be affected, resulting in limited uric acid production. In the event of a hepatic portovascular shunt, urate uroliths would be seen predominantly in young cats, rather than in older animals. However, there is no significant correlation between the age of cats and urate stone formation. Therefore extensive serum chemistry analysis (purine metabolites, ammonia levels, liver function tests) needs to be performed to address the role of hepatic portovascular shunt in urate urolithiasis in cats.
In serum at physiological pH, uric acid is circulated in the form of monosodium urate. In mammals renal excretion is the major route of uric acid excretion, although residual levels of uric acid also are eliminated via the intestine. During renal clearance, blood urate is filtered completely through the glomerulus, followed by the majority (≈90 per cent) of the urate fraction being reabsorbed back into the circulation at the proximal tubules. This mechanism leaves only 10 per cent of the plasma urate for excretion in the urine.8 The reabsorbed uric acid is metabolized further in the liver, with a fraction that is not catabolized going through the glomerular filtration-reabsorption cycle.7 Postglomerular renal handling of uric acid varies among species, mainly at the level of proximal tubule reabsorption and secretion.8,33,34
In cats, the net urate reabsorption has been observed.12 Dogs also exhibit net reabsorption of urate. In Dalmatian dogs the urate excreted in urine is higher than the filtered uric acid. This indicates that Dalmatian dogs have either a defect in renal urate reabsorption or abnormal tubular secretion of urate, or both. Studies that explored the renal physiology of this phenomenon confirmed that Dalmatian dogs have deficient proximal tubular urate reabsorption.35 However, later studies involving kidney and liver transplants implicated defective transport mechanisms in hepatocytes as the etiology of the hyperuricosuria in Dalmatian dogs. Specific cellular transporters involved in the pathogenesis of this condition in Dalmatian dogs have not been identified.
Urate transporters in epithelia of the proximal tubules are essential for urate reabsorption.6 Various membrane-associated urate transporters have been identified in human beings. Human beings reabsorb 90 per cent of the filtered urate through the sodium couple anion exchanger (Uric Acid Transporter-1, URAT1) located on the apical brush border of the proximal tubule epithelium.36 Studies have shown that a mutation in the URAT1 gene is manifested as hyperuricosuria and hypouricocemia.36
Another urate transporter, UAT1 (also known as galectin-9) is expressed on the apical and basolateral membrane of proximal tubular cells as well as in other tissues. UAT1 is postulated to be functioning as a bidirectional transporter of urate across the tubular epithelia.37 Urate also is transported into the proximal tubular epithelial cells via two other anion exchangers, known as OAT1 and OAT3. These transporters are located primarily at the basolateral membrane of the epithelia.38–40 In addition, multidrug-resistance–associated protein 4 (MRP4), an organic anion transporter, was reported to mediate the secretion of urate into the lumen from the tubular cells. MRP4 also is expressed in the liver.41
Recently another potential urate transporter, SLC2A9 (a fructose transporter), was reported in human beings by Vitart et al.42 The gene SLC2A9 encodes two protein isoforms with different lengths, each carrying around 10 different single nucleotide polymorphisms (SNPs). The causal role of these genetic variabilities on serum uric acid levels in human beings has yet to be identified.
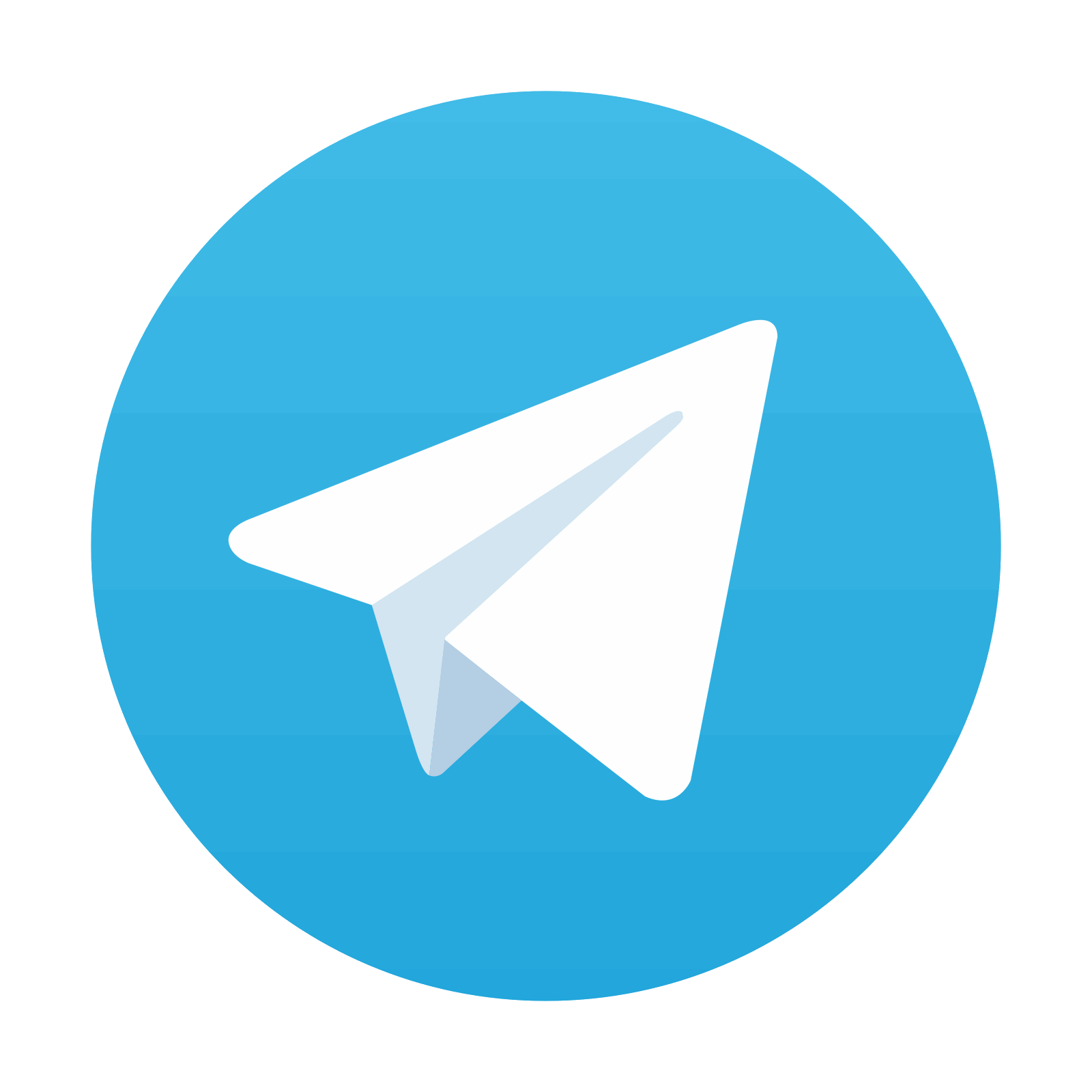
Stay updated, free articles. Join our Telegram channel

Full access? Get Clinical Tree
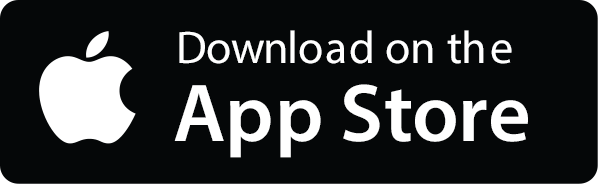
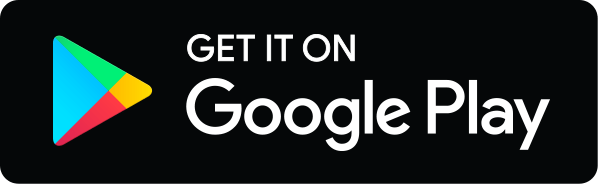