CHAPTER 11 Probiotics in Feline Medicine
The mammalian intestinal tract contains a complex, dynamic, and diverse society of pathogenic and nonpathogenic bacteria. Researchers have estimated that the human body contains 1014 cells, only 10 per cent of which are not bacteria and belong to the human body proper.1 There has been extensive research on the mechanisms by which pathogenic bacteria influence intestinal function and induce disease; however, recent attention has focused on the indigenous nonpathogenic microorganisms and the ways by which they may benefit the host. Initial colonization of the sterile newborn intestine occurs with maternal vaginal and fecal bacterial flora. The first colonizers, which have a high reductive potential, include species such as enterobacter, streptococcus, and staphylococcus. These bacteria metabolize oxygen, favoring the growth of anaerobic bacteria such as Lactobacillus species and Bifidobacterium species. Colonization with these bacteria is delayed significantly in cesarean deliveries,2 leading to delayed activation of the efferent limb of the mucosal immune response.3 The detrimental effects of not developing a normal bacterial flora are seen in germ-free mice, whose small intestines weigh less than those of their healthy counterparts. This is partly an effect of lymphoid constituent underdevelopment—in particular, of an absence of plasma cells in the lamina propria and Peyer’s patches and subsequent reduction in immunoglobulin (Ig) A expression. Exposure to bacteria results in a reversal of this phenomenon within 28 days of exposure.4
HISTORY OF PROBIOTIC HEALTH CLAIMS
Documentation of the health benefits of bacteria in food dates back to as early as the Persian version of the Old Testament (Genesis 18 : 8), which states that “Abraham owed his longevity to the consumption of sour milk.” Plinius, a Roman historian in 76 bc, recommended the use of fermented milk products for the treatment of gastroenteritis.5 One-hundred years ago, the Nobel Prize–winning Russian scientist Elie Metchnikoff suggested that the ingestion of Lactobacillus-containing yogurt decreased the number of toxin-producing bacteria in the intestine, contributing to the longevity of Bulgarian peasants.6 These observations led to the concept of a “probiotic” (from the Greek, meaning “for life”). The term probiotic was first used in 1965 to define “substances secreted by one microorganism that stimulate the growth of another” and was thus contrasted with the term antibiotic.7 The meaning of the word subsequently has evolved to apply to bacteria that “contribute to intestinal balance.” The current and more complete definition of a probiotic refers to “a preparation of or a product containing viable, defined microorganisms in sufficient numbers, which alter the microflora in a compartment of the host and by that exert beneficial health effects on the host.”8
Different strains of probiotic bacteria may exert different effects based on specific capabilities and enzymatic activities, even within one species.9,10 Different microorganisms express habitat preferences that may differ in various host species. Lactobacillus species are among the indigenous flora colonizing the chicken’s crop, the stomach of mice and rats, and the lower ileum in human beings. Bacteria colonizing such high-transit-rate sites must adhere firmly to the mucosal epithelium and adapt to the milieu of this adhesion site.11 The competition for adhesion receptors between probiotic and pathogenic microorganisms therefore depends on such habitat specifics. Four microhabitats in the gastrointestinal tract were outlined by Freter (1992) as follows12: (1) the surface of epithelial cells; (2) the crypts of the ileum, cecum, and colon; (3) the mucus gel that overlays the epithelium; and (4) the lumen of the intestine. The luminal content of bacteria depends greatly on bowel transit, resulting in a relatively low microbial density in the small bowel. It should be emphasized that a proven probiotic effect found in one strain or species can not be transferred to other strains or species because of differences in strain characteristics and habitat preferences.
MECHANISMS OF PROBIOTIC ACTION
PROBIOTICS BLOCK INTESTINAL PATHOGENIC BACTERIAL EFFECTS
Probiotics have been shown to mediate maintenance of the gastrointestinal microbial balance via two mechanisms: production of antibacterial substances and competitive inhibition of pathogen and toxin adherence to the intestinal epithelium.13–16
Probiotic-Derived Antibacterial Substances
Probiotics exert direct antibacterial effects on pathogens through production of antibacterial substances, including bacteriocins and acid.13,14 The primary mechanism of bacteriocin action is to form pores in the cytoplasmic membrane of sensitive bacteria, but they also can interfere with essential enzyme activities in sensitive species. The two-component lantibiotics, a class of bacteriocins produced by gram-positive bacteria such as Lactococcus lactis, are small antimicrobial peptides.13 These peptides have been found to be active at nanomolar concentrations to inhibit multidrug-resistant pathogens by targeting the lipid II component of the bacterial cell wall.17 Other non–lanthionine-containing bacteriocins are small antimicrobial peptides produced by Lactobacillus species. These peptides have a relatively narrow spectrum of activity and are mostly toxic to gram-positive bacteria, including Lactococcus, Streptococcus, Staphylococcus, Listeria, and Mycobacteria. Several strains of Bifidobacterium have been found to produce bacteriocin-like compounds toxic to both gram-positive and gram-negative bacteria.18 Probiotic bacteria, especially strains of Lactobacillus, produce acetic, lactic, and propionic acids that lower the local pH, leading to inhibited growth of a wide range of gram-negative pathogenic bacteria.19
Competitive Inhibition of Pathogen and Toxin Adherence to the Intestinal Epithelium
Several strains of Lactobacillus and Bifidobacterium are able to decrease adhesion of both pathogens and their toxins to the intestinal epithelium, and they can displace pathogenic bacteria even if the pathogens have attached to intestinal epithelial cells prior to probiotic treatment.15,16 However, specific probiotics or probiotic combinations should be selected based on their ability to inhibit or displace a specific pathogen. One of the mechanisms by which pathogenic bacteria bind to intestinal epithelial cells is the interaction between bacterial lectins and carbohydrate moieties of glycoconjugate receptor molecules on the cell surface. Studies have confirmed that probiotic inhibition of pathogen adherence to intestinal cells is mediated in part by competition for lectin-binding sites on glycoconjugate receptors on the cell surface.20,21 Blockade of bacterial enterotoxin binding also has been demonstrated as a mechanism with therapeutic potential.21a
PROBIOTICS REGULATE MUCOSAL IMMUNE RESPONSES
Both in vitro and in vivo studies show effects of probiotics on host immune functions: up-regulation of immune function may improve the ability to fight infections or inhibit tumor formation; down-regulation may prevent the onset of allergy or intestinal inflammation. One mechanism of probiotics regulating immunomodulatory functions is activation of toll-like receptors (TLRs).22,23
Enhancing Host Innate Immunity
Probiotics have the potential to stimulate innate immune responses against microorganisms and dietary antigens newly encountered by the host through several mechanisms. Intestinal dendritic cells can retain commensal bacteria by activating B lymphocytes selectively to produce IgA to reduce mucosal penetration by bacteria. The dendritic cells carrying commensals are restricted to the intestinal mucosal lymphoid tissues and thus avoid potential systemic immune responses.24 Treatment of mice with nonviable Lactobacillus GG (LGG) results in a significant elevation of fecal sIgA and enhanced secretion of interleukin (IL)–6, which augments IgA antibody responses at the mucosal surface.25 Importantly, LGG stimulates only moderate expression of costimulatory molecules, low production of tumor necrosis factor (TNF) and CCL20, and no production of IL-2, IL-12, IL-23, and IL-27 in dendritic cells compared with vigorous Th-1 type responses to pathogenic Streptococcus pyogenes. This phenomenon underscores the differential modulation of dendritic cells to pathogenic and nonpathogenic probiotic bacteria.26
Modulation of Pathogen-Induced Inflammatory Responses
Increasing Antiinflammatory Cytokine Production
Probiotics can induce dendritic cells to produce antiinflammatory cytokines, including IL-10, which suppress the Th1 response.27 However, the role of IL-10 production in probiotic prevention of Th1 responses is controversial and may be through both IL-10–dependent and IL-10–independent mechanisms.
Suppressing Proinflammatory Cytokine Production
Probiotics such as LGG have been shown to inhibit lipopolysaccharide (LPS)– and Helicobacter pylori–stimulated TNF production by murine macrophages.28 In addition, LGG-conditioned cell culture media decreases TNF production in macrophages, indicating that soluble molecules derived from LGG exert this immunoregulatory role.28 Inhibition of Escherichia coli or LPS-induced TNF production also occurs secondary to increased production of granulocyte colony-stimulating factor (G-CSF) production from macrophages. Probiotics such as LGG, L. rhamnosus GR-1, and their cell culture supernatants induce high levels of G-CSF production from macrophages, facilitating decreased production of TNF.29 The suppression of TNF production by G-CSF is mediated through STAT3 and subsequent inhibition of c-Jun N-terminal kinases (JNKs).29
Up-Regulation of Host Immune Responses to Defend Against Infection
Probiotics and commensal microflora may regulate a balance between proinflammatory and antiinflammatory mucosal responses, leading to intestinal homeostasis. Probiotics facilitate this important function by stimulating host immunological functions, including Th1 responses, through dendritic cell–directed T-cell activation. During colonization of mice with B. fragilis, dendritic cells take up and retain a bacterial polysaccharide that promotes maturation of dendritic cells, Th1-type cytokine production including IL-4, IL-12, and interferon (IFN)–γ, and subsequent CD4+ T-cell expansion.30
Regulation of Immune Responses by Probiotic DNA
There have been a number of intriguing studies documenting the beneficial immunomodulatory properties of probiotic DNA in people and murine models. DNA isolated from the probiotic VSL#3 mixture decreases LPS-activated IL-8 production and TNF and IFN release in vivo and in vitro. VSL#3 DNA also inhibits p38 MAP kinase and delays NF-κB activation.31 The unmethylated CpG dinucleotides, found commonly in bacterial and other nonmammalian genomes, activate innate immunity through TLR9. Administration of E. coli–derived DNA protects against injury in the dextran sulfate sodium (DSS) model of colitis in a TLR-dependent manner.22 The specificity of TLR9 response was confirmed following the loss of CpG protection in a TLR9-knockout model.22 Of equal importance, the study by Rachmilewitz et al showed a therapeutic effect of E.coli–derived DNA when given subcutaneously to mice with experimentally induced colitis. These pivotal studies prove that the protective effects of probiotics are mediated by their own DNA rather than by their metabolites or by their ability to colonize the colon. TLR9 signaling is essential in mediating the antiinflammatory effect of probiotics, and live microorganisms are not required to attenuate experimental colitis because nonviable probiotics are equally effective.
Differential Activation of TLRs by Probiotics in Immune Cells
Different probiotic bacteria stimulate distinct TLRs on host cells, an essential consideration in designing any therapeutic trials. Probiotic bacteria possess molecular recognition patterns similar to pathogenic bacteria; however, the probiotic organisms do not normally initiate pathogenic inflammatory responses. The probiotic E. coli Nissle 1917 expresses increased levels of both TLR2 and TLR4,23 whereas the probiotic VSL#3 mixture mediates its immunostimulatory response via TLR9 signaling.22 It appears that probiotics exert both up-regulatory and down-regulatory effects on immune responses, and TLR-regulated signaling pathways appear to be one of the mechanisms for these immunoregulatory actions. There clearly is a need to define the mechanism(s) for observed differences among the signals induced by probiotics and pathogens, which use similar receptors to induce divergent responses.
PROBIOTICS REGULATE INTESTINAL EPITHELIAL CELL FUNCTIONS
Substantial evidence indicates that probiotic bacteria stimulate intestinal epithelial cell responses, including restitution of damaged epithelial barrier,32 production of antibacterial substances and cell-protective proteins,33 and prevention of cytokine-induced intestinal epithelial cell apoptosis.34 Many of these responses result from probiotic stimulation of specific intracellular signaling pathways in the intestinal epithelial cells.
CURRENT APPLICATIONS AND USES OF PROBIOTICS IN HUMAN BEINGS
One important characteristic of probiotics is their ability to suppress the proliferation and virulence of pathogenic organisms; this is an increasingly well-documented role of probiotic bacteria in the gastrointestinal and genitourinary tracts. However, it is becoming increasingly clear that probiotic microbiota have direct effects on human physiology and immunity, including allergic disease (e.g., asthma, hay fever), autoimmune diseases (e.g., multiple sclerosis and type 1 diabetes), diseases of the oral cavity (e.g., periodontal disease and caries), and diseases of the nervous system (e.g., autism and depression).35 This section will review our current understanding of the multiple, systemic effects of probiotics in human beings, dogs, and cats.
PROBIOTICS AND ORAL HEALTH
Oral disorders in people, in particular dental caries, periodontal disease, oral Candida albicans infection, and halitosis, are associated with an alteration or imbalance in the oral microbial flora that potentially can be manipulated or restored with the introduction of probiotics.36 These probiotics must adhere to and colonize the specific surfaces of the oral cavity to confer their long-term health benefits. Although this is possible with individual strains of bacteria, the best effects appear to be the coordinated application of multiple strains that coaggregate and generate beneficial biofilms—a process that has been difficult to duplicate with many probiotics used individually. The first colonizers of the human oral cavity are Lactobacillus species, followed by Viridans group Streptococci. However, Streptococcus mutans, the primary agent of dental caries, is not present in the mouth until the eruption of the first teeth, because it requires solid surfaces for attachment and proliferation.37 These bacteria have highly developed mechanisms for carbohydrate metabolism that allow them to convert carbohydrates to exopolysaccharides, which promote bacterial attachment to dental surfaces and enhance accumulation of oral microbes in plaque to form biofilms.38 Thus the potential role of probiotics is to interfere in several steps of oral biofilm formation, modify the development of oral microecology, and reduce the proliferation of plaque-inducing microorganisms in saliva. Unfortunately, permanent oral colonization by established probiotics has not been reported when exogenous strains (Lactobacillus species or Bifidobacterium species) have been administered to human subjects.39,40 Therefore, although multiple studies show beneficial reductions of dental caries and reduction of oral Streptococci in both in vitro studies and in clinical trials, further work is needed to find optimal combinations of bacteria that can develop permanent colonization and show long-term benefit.41–43 There has been only one randomized, controlled trial performed to date evaluating probiotics for management and prevention of oral Candida infections and periodontal disease.44 In vitro studies demonstrate that Lactobacillus species and other probiotic bacteria inhibit the growth of oral pathogens involved in periodontal disease, such as Porphyromonas gingivalis, Prevotella intermedia, and Bacteroides spp., as well as the invasive yeast Candida albicans, an organism that commonly affects elderly, immunocompromised human beings or individuals with xerostomia.44–46 As a result, probiotic applications in oral biology continue to be an area of significant research and clinical interest.
PROBIOTICS IN GASTROINTESTINAL DISEASE
Of the potential benefits of probiotics, their role in prevention or treatment of gastrointestinal disease in human beings and animals has drawn the most interest. Human and experimental studies with probiotics have targeted specific health benefits associated with three functional areas of the gut microbiota: metabolic effects, protective effects, and trophic effects.47 Probiotics have metabolic effects on digestion, particularly of lactose and other disaccharides, and on the production of intestinal gas, a significant problem in patients with irritable bowel syndrome (IBS).
In one study the addition of probiotics such as Lactobacillus and Streptococcus (which are present as starter cultures in yogurt) to the diet of lactose-intolerant individuals resulted in improved lactose digestion and fewer clinical symptoms due to the presence of β-galactosidase, which hydrolyzes lactose, in these bacteria.48 The effect of probiotics in reducing bloating and gas production in patients with IBS has been well documented in randomized clinical trials. Colonic bacteria ferment ingesta to produce short-chain fatty acids, hydrogen, carbon dioxide, water, and other gases. Some microbial species produce large amounts of gas; other species consume gas, particularly hydrogen. The balance between gas-consuming and gas-producing microbes determines the amount, frequency, and odor of intestinal gas production. To date, it appears that Bifidobacterium species have the highest rates of therapeutic benefit in adults with IBS.49 However, in a study in colicky infants, a Lactobacillus strain resulted in the greatest reduction in intestinal bloating and gas.50 These different responses highlight the complexity of interactions between the microflora in each unique ecological habitat and emphasize the need to be cautious in predicting specific responses in individual subjects.
The protective effects of probiotics on the gastrointestinal tract include the prevention and treatment of acute diarrhea, the eradication of H. pylori infection, and prevention of systemic infections (septicemia) from bacterial translocation.47 Acute diarrhea due to antibiotics, nosocomial infections, community-acquired infectious enteritis, and traveler’s diarrhea are common disorders that have been managed with a variety of probiotic formulations. There have been a plethora of clinical trials studying the efficacy of probiotics in the treatment of acute diarrhea in adults (infectious diarrhea, traveler’s diarrhea); however, the evidence is most compelling for the administration of probiotics to decrease morbidity in people with antibiotic-associated diarrhea. In placebo-controlled studies, antibiotic-induced diarrhea occurred in 15 to 27 per cent of adults receiving placebo, but in only 3 to 7 per cent of adults receiving the probiotic.51 It is important to recognize that many different strains or combinations of strains were tested in multiple studies (e.g., L. rhamnosus GG, L. acidophilus, L. casei, Bacillus clausii), so specific recommendations are difficult. Nevertheless, meta-analyses of controlled trials and the Cochrane Systematic review concluded that some probiotics can be used to prevent antibiotic-associated diarrhea in children and adults.52–55
Prevention and management of Clostridium difficile infection in human beings secondary to prolonged antibiotic administration is a significant concern due to the morbidity associated with this infection and the difficulty in treatment. There have been few randomized controlled studies evaluating the efficacy of probiotics for management of C. difficile infection, and most have utilized the yeast organism Saccharomyces boulardii in this capacity. However, in one nonrandomized clinical study of a group of seven dialysis patients in a nephrology ward who developed C. difficile infection unresponsive to standard antibiotic therapy, S. boulardii administration was associated with complete resolution of the diarrhea in 75 per cent (5) of patients.56 Probiotic therapy with S. boulardii also was associated with a significant reduction in recurrent episodes of C. difficile infection (17 per cent of patients), versus 50 per cent of patients receiving placebo.57 The efficacy data of probiotics for prevention of community-acquired diarrhea and traveler’s diarrhea are more unclear; however, most studies suggest that probiotic therapy is beneficial. In a recent meta-analysis, probiotic therapy was more effective in preventing traveler’s diarrhea (7 per cent of people developed diarrhea) than a placebo group in which 31 per cent of people developed diarrhea.58
Another area of intense interest is the role of probiotics in preventing or inhibiting the colonization of the gastric mucosa by H. pylori. Some strains of Lactobacillus are known to inhibit the growth of Helicobacter in vitro; however, when these bacteria were added to yogurt in a clinical trial, they were not effective in eradicating the infection.59 A recent meta-analysis of 14 randomized trials evaluating the efficacy of probiotic therapy in combination with standard triple or quadruple antibiotic therapy showed that probiotic supplementation resulted in a higher incidence of Helicobacter infection eradication and a decreased incidence of antibiotic-associated diarrhea.60
Probiotics currently are being evaluated for their ability to reduce bacterial translocation and to prevent development of systemic infection (sepsis). This is a common, and potentially fatal, complication of a variety of pathological conditions resulting in mucosal barrier disruption (e.g., postoperative patients, and people with severe pancreatitis, multisystem organ failure, or necrotizing enterocolitis). Current recommendations for the implementation of probiotics in this group of patients are considered preliminary because of the paucity of large, randomized trials in this area. However, in a group of liver transplant patients who were given either antibiotic therapy for bowel decontamination, live probiotic (a Lactobacillus), or dead probiotic (same organism), the results were striking: infections occurred in 48 per cent of the antibiotic group, 13 per cent of the live probiotic group, and 34 per cent of the dead probiotic group.61 Additional randomized trials are needed to make confident predictions and recommendations for the administration of probiotics in postsurgical patients; however, the general consensus is that probiotics appear to be beneficial.47 Conversely, use of probiotic therapy in critically ill patients has not been generally recommended because additional evidence is still needed to support safety and effectiveness in that setting.
The third important area of probiotic influence in the gastrointestinal tract is its trophic effects on mucosal immunity and epithelial cell growth. There are three specific focus areas under this umbrella: IBD, food allergy, and colon cancer. Although the etiology of IBD in human beings (e.g., Crohn’s disease and ulcerative colitis) is unknown, substantial experimental and clinical evidence suggests that the mucosal immune system displays an aberrant response towards luminal antigens, most probably commensal bacteria, in genetically susceptible people.62 It is well known that certain bacteria activate proinflammatory mucosal responses, while others down-regulate intestinal inflammation. Thus it has been hypothesized that creating a favorable local microecology may restore homeostasis of the local immune response and thus resolve the intestinal inflammation.47 Multiple randomized clinical trials have been performed combining standard drug therapy with different strains of probiotic (Lactobacillus, E. coli, Bifidobacterium) and one combination probiotic product (VSL#3: three Bifidobacterium spp., four Lactobacillus spp., and S. salivarius).63–65 In these trials, addition of probiotics to the therapeutic regimen resulted in longer relapse-free remission times in patients with ulcerative colitis in some studies, but not in others; however in Crohn’s disease, the results were uniformly equivocal. The best evidence for use of probiotics in human beings with IBD is in chronic relapsing pouchitis, in which administration of VSL#3 was associated with a relapse in only three of 20 patients in the 4-month test period, compared to all of the patients relapsing in the placebo group.66 These results underscore the importance of cautiously interpreting studies utilizing probiotics in patients with IBD, because the dysbiosis (dysregulated bacterial ecosystem) varies in each form of IBD, necessitating careful selection of specific bacteria for different intestinal disorders.
In summary, in people evidence for the benefit of using probiotics to treat certain gastrointestinal disorders is increasing as more randomized trials are completed. At this time, there is level 1 evidence (i.e., data from either high-quality, randomized controlled trials with statistically significant results and few design limitations or from systematic reviews of trials) for effectiveness of probiotics in treating lactose intolerance/maldigestion, treating acute infectious or nosocomial diarrhea in children, preventing or treating antibiotic-associated diarrhea, preventing and maintaining remission of pouchitis in adult human beings, and maintaining remission of ulcerative colitis in adults.47 In addition, there is level 2 evidence (evidence obtained from randomized trials that have limitations in methodology or results that have wide confidence intervals) for using of probiotics to treat traveler’s diarrhea, prevent sepsis secondary to severe acute pancreatitis, and prevent infections in postoperative patients.47 As improved tools for investigation of gut colonization and microbial communities are developed, a more complete picture of the role of commensal microbes and the interactions of these microflora in inducing or controlling inflammation will be gained, facilitating better selection of probiotics and improved outcomes in the patients with gastrointestinal disease.
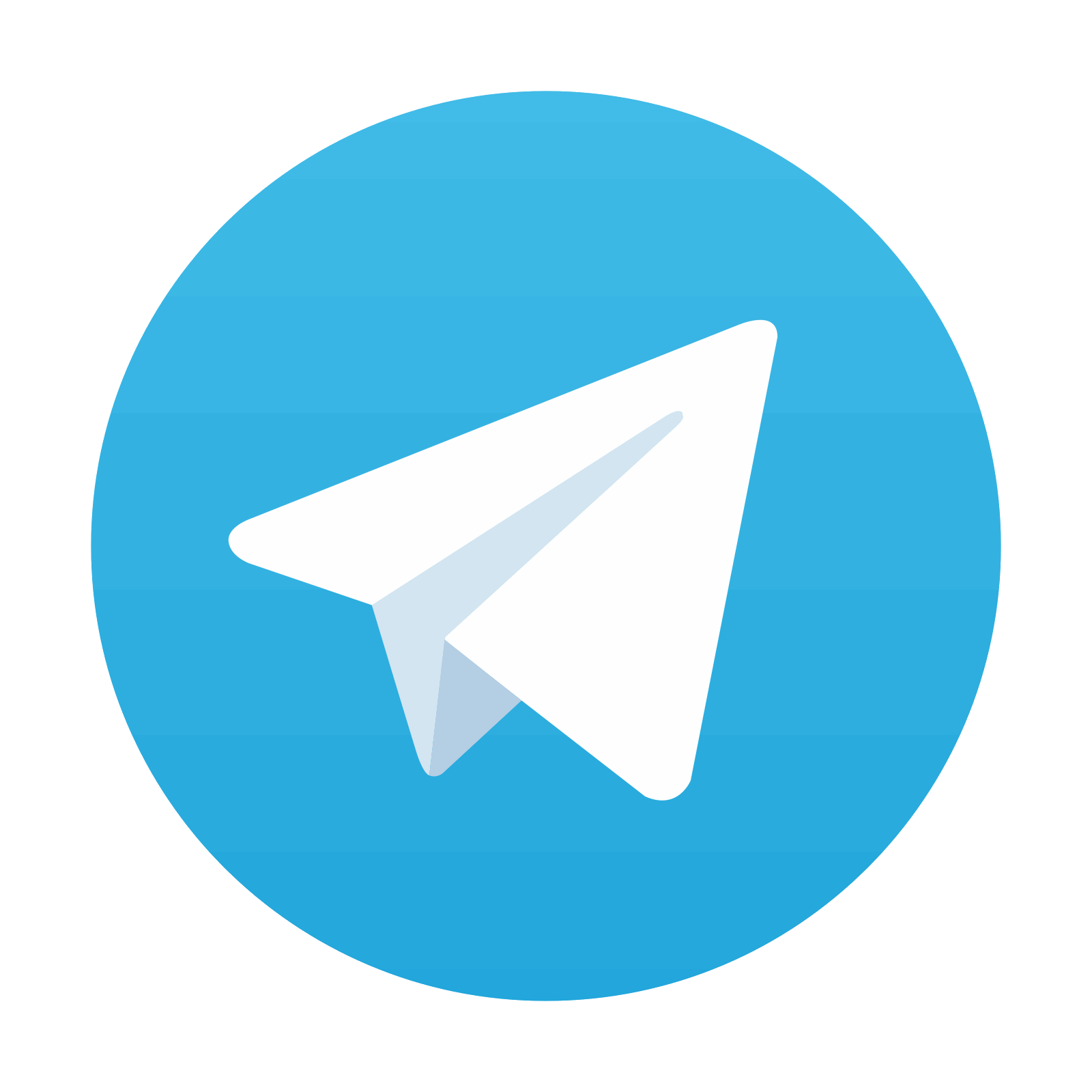
Stay updated, free articles. Join our Telegram channel

Full access? Get Clinical Tree
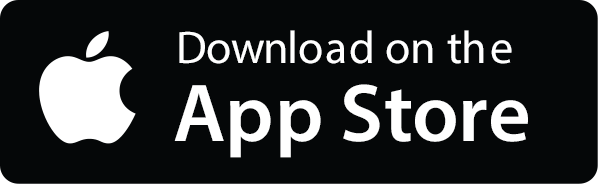
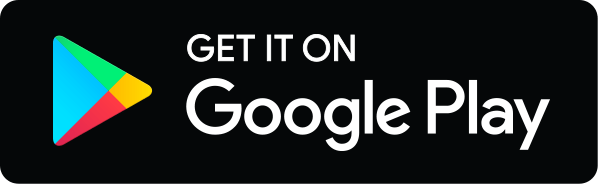