Chapter 27
Primate Transfusion Medicine
Stephen Cital1, Angela Colagross-Schouten2 and Laura Summers3
1San Francisco Zoo, San Francisco, California, USA
2California National Primate Research Center, Davis, California, USA
3Carrington College, Stockton, California, USA
Introduction
Richard Lower first demonstrated the transfusion of blood from one animal to another at Oxford in 1665. Since then, scientists have discovered how to prevent coagulation of blood to allow for transfusions without the donor present, elucidated the advantages of using species-specific blood for transfusions, and uncovered the importance of blood typing. Discoveries such as these have not only benefitted human medicine, but veterinary medicine as well, setting the groundwork for mechanisms for crossmatching, collection and storage of blood, and replacement therapies. These advances were achieved in great part due to the important role animals have played in transfusion medicine research. In particular, non-human primates (NHPs), specifically the Rhesus Macaque, were involved in critical developments in the understanding of blood compatibility, such as the discovery of the rhesus (Rh) factor. This factor, named for the rhesus monkey, is one of several antigens in the Rh blood group system, and is the most important blood group system after the ABO system in humans.
Blood transfusions in NHPs have been used extensively in research as a means for disease transmission (Jehuda-Cehen et al. 1991), disease treatment (Jahrling et al. 2007), blood storage advances, hemoglobin synthesis, therapies for irradiation, and tissue transplantation (Van Es et al. 1977). As NHPs have great genetic, physiologic, and immunologic similarities to humans, they are considered excellent models for the study of blood transfusions. Experience gained in the use of this species has also aided the use of blood transfusions in a clinical setting for captive NHPs, as is done in small animal practice.
NHP red blood cells (RBCs) are morphologically similar to human RBCs. Primate RBCs are biconcave with an approximate diameter of , depending on the species, age, and sex of the animal (Thrall 2004). RBCs of chimpanzees, gibbons, baboons, rhesus monkeys, and some New World (NW) monkeys have a half-life of 14–16 days and a life span of 50–60 days; approximately half of that for humans (Rowe 1994).
Indications for blood transfusions
The various indications for blood transfusions in NHPs are often dictated by the housing and history of the animals. Such indications are distinguished as either having an acute or chronic onset (Figure 27.1).

Figure 27.1 A pale Squirrel Monkey displays signs of lethargy secondary to severe anemia.
Acute anemia
Acute blood loss in NHPs is often associated with clinical signs such as skin pallor (especially around the mouth), pale mucous membranes, weakness, lethargy, and tachypnea (Tocci 2010). Causes can include blood loss from trauma, surgical complications, prolonged menses in species that menstruate, or pregnancy complications, including dystocia or retained placenta(s). Infectious agents can also cause acute anemia by destroying RBCs, such as during parasitemia with one of several Plasmodium spp. (malaria) (Egan et al. 2002). Other parasitic causes of anemia include hemobartonellosis in squirrel monkeys (Adams et al. 1984), Trypanosoma cruzi in NW monkeys, Hepatocystis spp. in Old World (OW) monkeys and apes, and babesiosis, which should be especially considered in NHPs that have been recently imported or are housed in outdoor facilities. Viral infections can cause an anemia, such as with simian parvovirus (O’Sullivan et al. 1994; Green et al. 2000). Acute aplastic anemia can also result from infection, exposure to certain drugs, or with some autoimmune disorders. It is interesting to note that marmosets do not develop anemia as rapidly or to the same extent as other monkeys because their levels of hemolytic complement are very low (Rommel et al. 1980).
During acute blood loss, a packed cell volume (PCV) or hematocrit (HCT) <20% is generally considered indicative of need for a RBC transfusion, as delivery of oxygen to the tissues is likely compromised. Additionally, identifying and addressing the cause of blood loss is paramount to therapeutic success, as is addressing underlying health concerns such as dehydration, diarrhea, and potential infections. When determining transfusion volumes, although not specific to NHPs, a general rule used based on what is seen in dogs and cats is that every 3 mL/kg of whole blood (WB) administered will increase the recipient HCT by 1%. In severe anemia cases, the use of packed RBCs (PRBCs) might be more efficient than WB, as only 1 mL/kg is needed to increase the HCT by 1%, again, based upon recommendations for dogs and cats (Short et al. 2012).
Chronic anemia
Chronic conditions can be more insidious and difficult to identify as causes are less visually obvious and animals have a greater ability to adapt to a decreasing PCV over time. Therefore, patients demonstrate fewer or more subtle clinical signs. Gastrointestinal (GI) blood loss often goes undetected and can be the result of gastric ulceration associated with chronic stress, Helicobacter pylori infection (although this association is debatable), or prolonged use of non-steroidal anti-inflammatory drugs (NSAIDs). The presence of GI adenocarcinoma, commonly found in aged rhesus macaques, can also cause a slow chronic blood loss that can lead to anemia. Visually undetectable blood loss from the GI tract can be detected using fecal occult blood tests, which provide rapid, although non-specific, confirmation of GI bleeding.
Small amounts of blood loss over time can also result from epistaxis, such as in cases of moraxella infection in indoor housed OW monkeys, or hematuria associated with cystitis, idiopathic hematuria, or neoplasia. Females with endometriosis or uterine polyps can have heavier menses during their cycle, resulting in a greater blood loss, which can lead to anemia over the course of several months. Infectious agents can also cause a chronic anemia by preventing the regeneration of RBCs such as in simian acquired immunodeficiency syndrome (SIV), simian retrovirus (SRV), or tuberculosis, when the bone marrow’s ability to regenerate RBCs is impaired by inadequate hormone production or destruction. Kidney damage or infections can also impact the body’s ability to regenerate RBCs due to decreased production of erythropoietin.
Dietary inadequacies including vitamin deficiency (e.g., iron, folate, B12) and nutritional deficiencies resulting from diets not meeting unique species-specific needs, such as vitamin E responsive hemolytic crisis seen in the Aotus species (Abee et al. 2012), can also be a source of progressive chronic anemia. Likewise, malabsorption secondary to health issues including poor appetite, diarrhea, or severe cryptosporidiosis can also deplete iron stores, resulting in a decreased ability to regenerate RBCs. Another metabolic cause is anemia secondary to oxidative stress, as in the wasting syndrome accompanied by anemia in the marmoset (Logan and Khan 1996; Otovic et al. 2015).
In most cases, the decision to transfuse an NHP with chronic blood loss is based on clinical signs rather than a laboratory value threshold. Patients might only demonstrate fatigue or mild weakness under conditions of stress, even with large reductions in RBC mass. However, generally a HCT <17% warrants a blood transfusion. Additionally, it is important to carefully evaluate the patient’s complete blood count (CBC) to assess for regeneration and/or signs of infection. Performing a diagnostic work up, as well as addressing any additional underlying health problems, is necessary to provide the patient with the best chance of benefitting from a blood transfusion and, ultimately, an uneventful recovery. Ignoring health problems that are a source of chronic blood loss, such as a GI adenocarcinoma, will result in an animal’s ongoing need for blood transfusions.
Blood components
Whole blood
WB contains plasma, proteins, platelets, RBCs, and white blood cells and is considered fresh until 6 hours after collection. After 6 hours, the platelets are no longer functional, but the blood can be stored and used for up to 25 days, depending on the anticoagulant used. At 4°C or frozen, NHP blood products have a comparable storage life to human blood products, although the in vivo lifespan of RBCs in NHPs is 50–60 days, approximately half that of humans (Rowe 1994).
WB is used most commonly in cases of acute blood loss with associated hypovolemia. In situations where blood donors are readily available, it is also convenient and expeditious, as there is no need for processing and the transfusion can occur as soon as the donor’s blood has been collected. Cryopreservation is most often performed in larger zoo or research settings where numerous animals of the same species reside and clinical cases for using those products arise.
Packed red blood cells
Packed red blood cells (PRBCs) can also be used to treat severe cases of anemia. PRBCs are collected by centrifuging WB, removing the plasma component, and adding RBC preservatives to enable storage at 4°C for up to 35 days in dogs (Wardrop et al. 1997) and 42 days in humans (Koch et al. 2013), depending on the anticoagulant and RBC preservative used. Indications for transfusion of PRBCs include chronic anemia in patients with conditions that predispose to volume overload, such as cardiac disease or failure. It should be noted that the length of storage time of RBCs can result in a decrease in their function and survival in vivo (Aucar et al. 2009; Doctor and Spinella 2012; Hann et al. 2014).
Fresh frozen plasma
In NHPs with a severe coagulopathy, fresh frozen plasma (FFP) might be indicated and can be administered at doses of 10 mL/kg up to three times daily (Abee et al. 2012). Indications for the use of FFP, as suggested in human medicine, include replacement of coagulation factors or protein in patients with liver disease, anticoagulant overdose, or disseminated intravascular coagulation (DIC) with clinically relevant hemorrhage (Roback et al. 2010).
Fluid support
If WB, blood components, or artificial blood substitutes are unavailable, the importance of tailored fluid therapy cannot be overstated. Particularly in hypovolemic patients, fluid therapy can play a vital role in the restoration of vascular volume, which ultimately will aid in tissue perfusion. Moreover, monitoring the effects of appropriate fluid administration includes assessing circulatory function by means of cardiac output. An arterial blood pressure line is ideal for recording changes in circulatory function, but these values can also be monitored less accurately with non-invasive blood pressure devices and careful attention to trends (Muir 2013).
Blood groups and types
New and Old World monkeys and apes have similar blood types as described in the human ABO system (Socha and Morr-Jankowski 1986; Malaivijitnond et al. 2008). The A and B represent two different proteins or antigens that can be associated with the RBC and O represents RBCs that have neither of these antigens present. There are also corresponding antibodies in the plasma to identify foreign antigens introduced into the body. For example, NHPs with blood type A have anti-B antibodies, those with blood type B have anti-A antibodies, while those with blood type AB have no antibodies and those with blood type O have both antibodies. However, unlike in humans, the A, B, and O blood group antigens in New and Old World monkeys are not bound to RBCs and are found only on epithelium, vascular endothelium, and in exocrine secretions and saliva, as demonstrated in rhesus and cynomolgus macaques (Chen et al. 2009; Premasuthan et al. 2011).
Blood type O is considered relatively rare in macaques, while the prevalence of other blood types has been associated with geographical regions. A rapid multiplex PCR used to determine blood types of Indian, Chinese, and cynomolgus macaques found that the most common blood group was B, followed by AB and A (Premasuthan et al. 2011). In rhesus macaques, blood group A was more common in animals of Chinese origin than those of Indian origin, and blood group O was not detected, suggesting that this blood type is rare in rhesus.
Another common blood protein antigen is the Rh factor, named after the rhesus monkey, discovered during animal studies performed to define and understand blood antigens. This blood antigen is very similar between humans and rhesus macaques. Although there are more than 200 other blood antigens in many different blood group systems, the ABO and Rh remain the most critical when identifying compatible blood donors through crossmatching (Table 27.1 and 27.2).
Table 27.1 Non-human primate blood groups (Lee and Doane 2005)
ABO blood type | Rhesus Macaque | Cynomologus Macaque | African Green (Vervet) Monkey | Baboon | Chimpanzee |
A | Rare | Present | Likely | Present | Present |
B | Common | Present | Likely | Present | Not present |
AB | Rare | Present | No data | Present | Not present |
O | Likely | Present | Likely | Rare | Rare |
Incidence might differ depending on sex, age, environment (indoor/outdoor), housing (group/individual/paired), or hormone levels (adolescence/pregnancy).
Table 27.2 Gorilla blood group system (Socha 2012)
Blood group system | Blood group | Phenotypes observed |
A-B-O | B | B |
M-N | N MN | N M1N or M2N; V.O |
Rh-Hr | Rh-positive | RC, RCF, R |
Secretor status | Sec |
Blood typing and compatibility testing
The large number of antigens expressed on the surface of the RBC in NHPs, paired with the lack of expression of A, B, or O antigens on RBCs, makes the blood typing process more complex than in humans. Even so, there are several methods described in the literature. For rhesus macaques, a commercially available method is the reverse gel system assay (Chen et al. 2009). However, this method requires that NHP serum samples be pre-absorbed on human type O RBCs, which might not be practical in many clinical settings. Alternatively, blood can be sent to one of many commercial laboratories that provide crossmatching services utilizing PCR. This might be useful in characterizing the blood types of all animals in an established colony, allowing the practitioner the luxury of knowing which animals are compatible prior to an emergency situation.
However, in most situations, a rapid cost-effective alternative is the major and minor crossmatch procedure (Weiss and Wardrop 2010). This is a relatively simple and straightforward method that can be performed in most basic laboratories and provide information regarding blood compatibility within 1 hour. The method requires a blood sample from the donor and recipient, and uses visual assessment of RBC agglutination and hemolysis to assess for compatibility (see Chapters 9 and 10). The major crossmatch tests donor erythrocytes against the serum of the recipient, whereas the minor crossmatch tests the serum of the donor against the recipient erythrocytes. It is important to note that this type of crossmatch should be performed as close to the time of transfusion as possible to avoid missing any newly developed incompatibilities.
Immunologic transfusion complications
Despite the large number and variety of blood antigens that exist in NHPs, use of non-typed blood in large volumes is not an uncommon practice and transfusion reactions appear to be uncommon (Abee et al. 2012). A study conducted to assess the onset and severity of transfusion reactions as a result of transfusing incompatible blood based on ABO blood type and differences in simian-type RBC specificities in rhesus macaques and baboons did not document any acute transfusion reactions, even after multiple transfusions (Socha et al. 1982). Based on these results, an NHP that has never had a blood transfusion has a very minimal risk of reaction. This is likely the result of low levels of pre-existing antibodies against donor RBCs, in combination with depressed or delayed antibody production when large quantities of blood are administered at short intervals. With repeated transfusions, higher antibody titers might result in an accelerated elimination of transfused RBCs, reducing the effectiveness of the blood transfusion (Socha et al. 1982). In situations when non-naive patients receive blood, crossmatching of the donor and recipient is therefore advisable.
Conversely, in situations involving critically ill NHPs that require a blood transfusion to prevent an imminent demise, it is advisable to immediately proceed with the transfusion rather than wait for confirmation of blood compatibility. In the authors’ experience with rhesus and cynomolgus macaques, when there is an acute need for a transfusion a significant transfusion reaction is not observed in NHPs that have not had pre-transfusion crossmatching. This might be because of the high prevalence of the common dominant blood type B in rhesus macaques (Socha and Morr-Jankowski 1986; Premasuthan et al. 2011) or because NHPs share a geographical blood group distribution.
Donor selection
Typically, blood transfusions rely on the presence of a blood donor or the availability of stored blood products, since commercial blood banks for NHP blood do not exist. Because NHPs are social species, there are ethical and regulatory requirements for social interaction with conspecifics. Therefore, a potential blood donor is often readily accessible as long as the animals have a similar health status and history. Whenever possible, the donor NHP should be homologous species. Heterologous transfusions can result in considerably shortened erythrocyte lifespan. Crossmatching is recommended for transfusions as in traditional pet species, but crossmatching might not be practical in certain diminutive species of primates. A limiting factor with crossmatching is that outside labs are often not willing to handle NHP blood due to zoonotic disease concerns.
The ideal donor is an adult of the same species in good body condition with no history of recent illness. In an emergency situation, it might not be practical to attempt to screen a donor for all diseases that might endanger the recipient. In the laboratory animal setting, having specific pathogen-free (SPF) blood products collected and stored is advantageous for emergency situations. If time is a pressing matter and traditional crossmatching cannot be performed minimally, it is advisable to collect a single drop of blood from the donor to evaluate PCV and cell morphology prior to blood collection.
Crossmatching using major and minor methods is always best practice (see Chapters 9 and 10). However, as previously mentioned, this might not be practical in certain emergency settings. Blood substitutes, such as hemoglobin-based oxygen-carrying solutions (HBOCs), are excellent oxygen carrier replacements for patients suffering from anemia or hypovolemia when other blood products are unavailable. There is a plethora of literature in human medicine, which can be readily translatable to NHP species. In general, guidelines used in dogs and cats can be applied to the treatment of NHPs when using HBOCs (see Chapter 6).
Blood collection
Blood collection from a donor NHP is very similar to that performed in small animals. The biggest difference is that the donor will likely require sedation and alternative collection sites will be used, given that blood collection from a jugular vein in NHP is not practical because of their anatomy. NHPs generally have shorter necks, with jugular veins that are well protected by muscle and other soft tissue, making access difficult or impossible. Other vessels, such as the cephalic and saphenous (popliteal) veins, can be used, but collection times might be increased due to their smaller size, which can also increase the chance of clot formation during collection. The preferable collection site is the femoral vein. Venipuncture for this site is directed within the “femoral triangle” (Figure 27.2) of the inguinal-proximal leg using the largest and most size-to-vessel appropriate gauge needle that can still allow reliable hemostasis when blood collection is complete.

Figure 27.2 Femoral triangle in a Blue-eyed Lemur; the line in the center indicates where to insert the needle for collection from either the femoral vein or artery.
Blood is collected into syringes containing an anticoagulant; options including sodium citrate, heparin, acid-citrate-dextrose (ACD), citrate-phosphate-dextrose (CPD), and CPD with adenine (CPDA-1) have been described. Anticoagulant to blood ratios should be used as described in the manufacturer’s guidelines. If using manufactured human or veterinary blood collection bags, following instructions regarding collection as indicated on the product information is recommended. The volume of blood collected from an individual donor should be limited to 10% of the donor’s blood volume or roughly 10% of body weight. The addition of replacement crystalloid fluids equivalent to the volume collected is encouraged, given either intravenously (IV) or subcutaneously.
Sedation and tranquilization protocols for donors
Medications administered to NHP donors should be minimized. Ketamine 5–20 mg/kg as an intramuscular (IM) or IV injection is effective in most NHP species. The addition of muscle relaxants or anxiolytics such as midazolam or diazepam are also helpful in enabling successful blood collection (Table 27.3
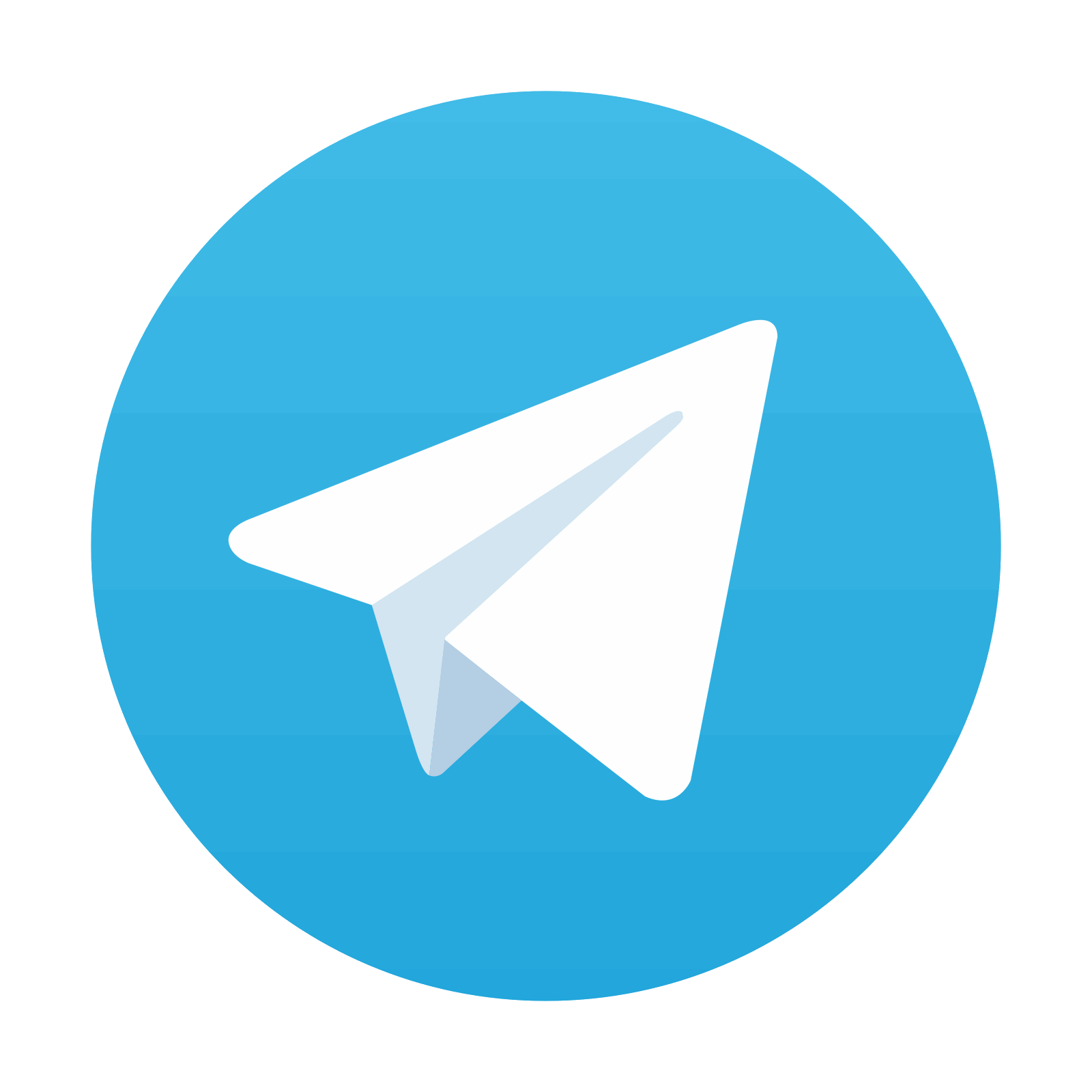
Stay updated, free articles. Join our Telegram channel

Full access? Get Clinical Tree
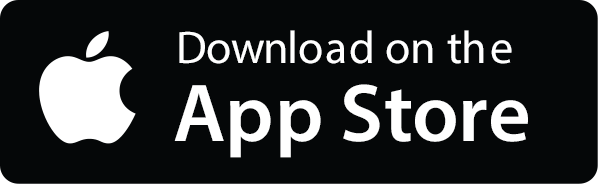
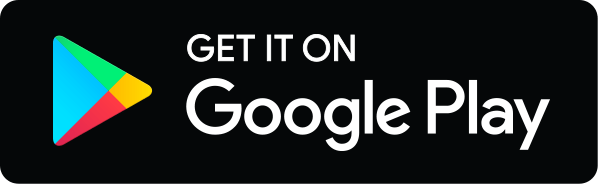