Chapter 3
Red Blood Cell Products
Caroline Kisielewicz
Chestergates Veterinary Specialists, Chester, Cheshire, UK
Introduction
The first successful blood transfusion between dogs was reported in 1665, but transfusions have only become commonplace in dogs and cats over the last 30–60 years (Lower 1665). Anemia results from hemorrhage, hemolysis, or ineffective erythropoiesis and red blood cell (RBC) transfusions are administered to anemic animals to replace this missing component of blood, thereby increasing oxygen-carrying capacity. Blood transfusions are not curative, but they are part of a supportive management strategy for anemic animals while a diagnosis is determined or a treatment is initiated. Availability of blood products is steadily increasing through the commercial provision of these for dogs and to a lesser extent for cats, development of donor programs, and access to blood component substitutes. With better awareness of the current storage and administration controversies, the appropriate use of these products for managing anemic animals is progressively improving the standard of care being provided for them (Kisielewicz and Self 2014).
Blood products
Fresh whole blood (FWB) is that which is collected and administered to a recipient within 4–6 hours; it contains all of the components of blood such as erythrocytes, leukocytes, platelets, and plasma proteins, including clotting factors. This product is most appropriate for the management of acute severe hemorrhage from surgery, trauma, or coagulopathies, although when other RBC component products are not available it could potentially be used to manage any cause of anemia (Prittie 2003).
Blood that is not used within 6–8 hours of collection becomes stored whole blood. This contains RBCs, WBCs, and plasma with reduced viability of platelets and reduced labile clotting factors (i.e., fibrinogen, factor VIII, and von Willebrand factor) (Nolte and Mischke 1995; Tsuchiya et al. 2003; Gosselin et al. 2013; Walton et al. 2014). It has a shelf-life of up to 35 days for dogs and cats, depending on the additive solutions used (Marion and Smith 1983; Bucheler and Cotter 1994). Stored whole blood (WB) is primarily used to manage acute severe hemorrhage if FWB is unavailable; it is not ideal for treating hemorrhage resulting from thrombocytopenia and certain coagulopathies due to the lack of platelets and clotting factors, respectively.
The use of WB to manage animals with hemolytic or chronic anemia can lead to circulatory (volume) overload. This, in addition to a constant shortage of blood products to meet demand, led to blood component separation in human blood banking in the 1980s and later in the veterinary field. FWB is centrifuged and supernatant plasma is removed, thereby creating packed RBCs (PRBCs), which contains RBCs, leukocytes, platelets, remnant plasma, and anticoagulant (Figure 3.1). This product is predominantly used for the management of hemolysis and chronic anemia. With the use of various additive solutions, PRBC products have a shelf-life of 35–42 days (Wardrop et al. 1994a,b, 1997). The supernatant plasma is used to produce fresh frozen plasma, frozen plasma, or cryoprecipitate and cryosupernatant (Figure 3.2).

Figure 3.1 A unit of canine packed red blood cells.

Figure 3.2 A diagrammatical representation of the different blood products that can be made using whole blood collected from an animal.
Canine transfusion medicine continues to adopt human blood-banking advancements as dozens of commercial canine blood banks are now found across North America, in addition to non-commercial in-house blood donation programs and resident blood donor dogs in some hospitals. Feline blood products are not as readily available. Many cats require sedation or anesthesia to allow blood to be collected, which adds risk to the procedure; the small volume of blood collected from cats also complicates component separation (Henson et al. 1994; Klaser et al. 2005). The growing demand for feline blood products has resulted in the increasing development of feline donation programs. Additionally, housing blood donor cats within some animal blood banks, as well as the opening of feline-only blood banks has resulted in the availability of commercial feline blood products in recent years.
Storage
With the advent of anticoagulants and additive solutions, blood banking has become a feasible option for increasing the availability of blood products. However, the inappropriate storage of these products can lead to acute life-threatening transfusion reactions in recipients (Patterson et al. 2011). Meticulous guidelines should therefore be implemented and observed in all veterinary centers that store RBC products.
Blood collection bags and additive solutions
Blood is typically collected from a donor animal directly into a blood collection bag of phthalate plasticized polyvinyl chloride (PVC) containing citrate-based anticoagulant (Figure 3.3). The addition of phosphate to the anticoagulant reduces the loss of phosphate from RBCs and allows continued production of adenosine triphosphate (ATP) by RBCs during storage (Hess 2006). Additive solutions were developed in human blood banking over a number of decades and have subsequently been used for the storage of canine and feline RBCs (Marion and Smith 1983; Wardrop et al. 1994a). These solutions provide nutrients for RBCs to enable longer storage and maintain their viability, particularly if the blood product is not intended to be used within 24 hours of collection. The anticoagulant-additive solutions most commonly used in canine and feline blood banking include citrate-phosphate-dextrose (CPD) with or without saline-adenine-glucose-mannitol (SAG-M), CPD-adenine (CPDA-1), and acid-citrate-dextrose (ACD) (Marion and Smith 1983; Price et al. 1988; Bucheler and Cotter 1994; Wardrop et al. 1994a, 1997; Castellanos et al. 2004).

Figure 3.3 This blood collection bag containing citrate-phosphate-dextrose-adenine (CPDA-1) anticoagulant can be used to collect a unit of whole blood from a dog despite being designed for human medicine.
There are ongoing studies into the development of superior additive solutions to further prolong the storage time of blood products in the human field, although these have yet to be introduced to the veterinary field (Hess et al. 2000; Kurup et al. 2003; Jarvis et al. 2003). A recent study investigated the effect of four different additive solutions on leukoreduced canine PRBC units stored for 41 days. The degree of hemolysis and ATP and 2,3-diphosphoglycerate (2,3-DPG) concentrations were satisfactory after 21 days of storage, but were significantly changed by 31 days with all additive solutions (Lacerda et al. 2014). Further studies are required for assessment of in vivo post-transfusion viability of RBCs before recommendations can be made regarding the use of these additive solutions.
Storage of RBC products
A dedicated refrigerator, exclusive for RBC product storage, is necessary (Figure 3.4). It is essential that blood products be separated from other products to avoid chemical and biological contamination. The refrigerator should have a continuous temperature monitoring device with an alarm and should be opened only when essential. Temperature logs should be regularly assessed to ensure that no marked fluctuations have occurred, which can affect the quality and safety of the RBC products. These products should be stored between 1 and 6 °C (34 and 42 °F) as inappropriate temperature conditions can cause hemolysis and increase the incidence of microbe contamination and proliferation in blood products (Sharma et al. 2004; Aalaei et al. 2014).

Figure 3.4 a. This refrigerator is dedicated to blood product storage. b. Inside the refrigerator, blood products are maintained horizontally with space between each bag. (Images courtesy of Marie K. Holowaychuk, DVM, DACVECC.)
RBC products have traditionally been recommended to be stored vertically in an upright position with air space between the units to allow the bags to “breathe” (Figure 3.5), but an in vitro study in humans suggested that horizontal storage provided optimal blood gas values and levels of hemolysis (Hogman et al. 1995). Products should be mixed gently every other day by inversion to allow all erythrocytes exposure to the nutrients in the additive solution whilst simultaneously checking for any visual abnormalities, although the same human study suggests that once weekly mixing is sufficient (Hogman et al. 1995).

Figure 3.5 Blood products are hung upright to maintain vertical storage to allow oxygen exposure to all sides of the bag.
Blood products have an expiry date determined by the combination of anticoagulant and additive solutions used. In order to minimize waste, it is recommended that older products are used first (Figure 3.6). A recent study found that the age of the PRBC unit administered to dogs does not appear to contribute to mortality when considering all transfusions. However, administration of PRBCs with longer storage duration is associated with the development or progression of coagulation abnormalities and thromboembolic disease. Dogs with hemolysis, in particular those with immune mediated hemolytic anemia (IMHA), have increased mortality when PRBC stored for longer is administered. The study concludes by suggesting that when transfusing PRBC to dogs with IMHA, units stored for <14 days should be chosen and older units reserved for managing dogs with anemia due to hemorrhage or ineffective erythropoiesis (Hann et al. 2014). This study clearly shows the importance of keeping a transfusion log book for recording details about the recipient, the blood products used, and information about the transfusion itself. An example of the different aspects that might be considered for inclusion in a transfusion log book can be found in Table 3.1.

Figure 3.6 This pharmaceutical refrigerator dedicated for blood product storage has an alarm that sounds when it is opened or the temperature increases beyond a set level. A reminder is posted on the front of the door to encourage use of older blood products before newer products to avoid wastage. (Image courtesy of Marie K. Holowaychuk, DVM, DACVECC.)
Table 3.1 Transfusion recipient, blood product, and transfusion details that should be included in every transfusion log
Recipient | Blood product | Transfusion details |
Name | Type of blood product | Date of transfusion |
Identification number | Donor blood type | Reason for transfusion |
Species and breed | Volume | Crossmatch results |
Gender and age | Production and expiry dates | Volume administered |
Body weight | Duration of transfusion | |
Recipient blood type | Transfusion reactions | |
Previous transfusions |
Storage lesions
RBCs develop lesions within a few hours of storage, attributable to morphologic changes, metabolic derangements, and oxidative damage, which progressively worsen during storage. These changes, collectively known as storage lesions, are detrimental to erythrocyte viability and function and result in reduced RBC survival following transfusion (Donadee et al. 2011; Obrador et al. 2015).
2,3-DPG modulates hemoglobin (HGB) oxygen affinity in dogs, but cats require chloride for oxygen affinity and their 2,3-DPG concentrations are naturally low (Bunn 1971). Lactic and pyruvic acid accumulate with blood storage, resulting in a progressive pH decrease. This acidic environment promotes a marked decrease in 2,3-DPG within the first 24 hours of canine RBC storage to undetectable levels within 2 weeks of human PRBC storage (Price et al. 1988; Raat and Ince 2007). HGB oxygen affinity increases as 2,3-DPG decreases, thereby theoretically reducing oxygen unloading after blood transfusion, which can negatively affect tissue oxygenation as well as patient morbidity and mortality (Raat and Ince 2007). Once transfused, RBC synthesize 2,3-DPG in the hours after transfusion, thus restoring normal levels within days (Heaton et al. 1989).
P50 is a point on the oxygen HGB dissociation curve at which HGB is 50% saturated with oxygen. It is therefore a measure of the affinity of HGB for oxygen; during anemia, P50 increases because the curve shifts to the right. While P50 decreases progressively during canine RBC storage, the P50 of stored HGB in cats decreases relatively little (from 35 mmHg to 31–32 mmHg) after 35 days of storage as cats require chloride for oxygen affinity and their 2,3-DPG concentrations are comparatively low (Wong and Haskins 2007).
ATP provides energy in RBCs and is used for maintaining the shape and deformability of the cells, as well as membrane transport functions and synthesis of nucleotides and glutathione (Obrador et al. 2015). ATP decreases in stored canine and human PRBCs (Price et al.1988; Raat and Ince 2007); the rate of decrease depends on the additive solutions used. As the ATP concentration decreases, erythrocyte shape degenerates and deformability deteriorates. This leads to erythrocyte hemolysis and increasing free HGB concentrations, which scavenge nitric oxide after transfusion, thus resulting in vasoconstriction (Raat and Ince 2007; Donadee et al. 2011). Reduced deformability also impedes RBC transit through the microcirculation, leading to reduced capillary perfusion and impaired oxygen delivery to tissues (Relevy et al. 2008).
Erythrocyte membrane microvesiculation develops in the absence of sufficient ATP and 2,3-DPG concentrations, as well as protein rearrangement and lipid oxidation (Raat et al. 2005; Bosman et al. 2008; Karon et al. 2012). Microvesiculation leads to the accumulation of pro-inflammatory and procoagulant microparticles (Hess 2006; Rubin et al. 2013). Microparticles develop physiologically from many cell types, including RBCs, leukocytes, and platelets, to protect against early cell death by shedding oxidized and polymerized lipids (Willekens et al. 2003). They are phospholipid-rich vesicles that contain membrane-bound and cytoplasmic proteins, and are usually removed by the mononuclear phagocyte system, but they accumulate progressively during PRBC storage and might have a role in some transfusion reactions (Rubin et al. 2010; Herring et al. 2013). There is ongoing research into the significance of microparticles in the development of storage lesions and associated adverse effects in transfusion recipients.
Nitric oxide made by vascular endothelium has a role as a local vasodilator. When RBCs become deoxygenated while moving through tissue circulation, they release vasodilatory compounds, a process partly dependent on ATP release from RBC. Free plasma ATP stimulates nitric oxide production, resulting in an increase in local blood flow, thus improving oxygen delivery to tissues (Liu et al. 2013). Because ATP concentrations are decreased in stored blood products, some studies have investigated the ability of stored RBC to improve peripheral tissue oxygenation after transfusion. This remains controversial, with various studies reporting conflicting findings. Whilst tissue oxygenation was not adversely affected in critically ill human patients receiving older stored blood products (>20 days) in one study, another showed a decrease in tissue oxygenation with transfusion of older units (>21 days) (Walsh et al. 2004; Kiraly et al. 2009).
Dogs with experimentally induced pneumonia had significantly more hemolysis and shorter duration of survival after transfusion with 42-day-old stored blood, in comparison to dogs receiving 7-day-old blood (Solomon et al. 2013). A similar more recent study confirmed shorter duration of survival and increased consequences of infection in dogs with experimentally induced pneumonia receiving 42-day-old stored blood, compared to 7-day-old blood (Wang et al. 2014). An increased prevalence of infection has been found in pediatric human patients undergoing cardiac surgery and receiving blood stored for >16 days, but particularly when stored for >25 days (Cholette et al. 2015). Human trauma patients receiving multiple units of older (>14 days) stored RBC products also have a significantly increased risk of mortality, leading to the recommendation to administer relatively fresh units to this patient group (Weinberg et al. 2010). However, conflicting evidence remains regarding the relationship between longer duration of blood product storage prior to transfusion and clinical outcomes. A recent human study, among others, demonstrated that the age of RBCs was not independently associated with increased mortality or an increase in length of hospital stay in critically ill patients (Aubron et al. 2014).
In summary, there is accumulating evidence for the presence of storage lesions and their negative effects on transfusion recipients. Research is ongoing to identify methods to prevent or minimize the development of storage lesions. Dogs with IMHA receiving blood transfusions might benefit from receiving blood stored for <14 days (Hann et al. 2014). While this could be the case for other conditions in both dogs and cats, current veterinary data is lacking.
Leukoreduction
Leukoreduction involves the removal of leukocytes and platelets from blood products by filtration. This procedure has become routine in human medicine in numerous countries, but remains uncommon in the veterinary field. Pre-storage leukoreduction of canine blood at 4 °C decreases the leukocyte count by 99.9% without adversely affecting in vitro and in vivo viability of erythrocytes after 35 days of storage (Brownlee et al. 2000). Leukoreduction of feline blood by gravity flow through a neonatal leukoreduction filter at 4 °C removes 100% of leukocytes although about 13% of the blood volume is lost in the filter (Schavone et al. 2012).
Leukocytes are metabolically active and produce cytokines that accumulate during storage and can contribute to storage lesions and possibly transfusion reactions in dogs (Nielsen et al. 1996; Ekiz et al. 2012; Graf et al. 2012). Erythrocyte hemolysis, decreases in 2,3-DPG, and release of vasoactive compounds are significantly lessened in leukoreduced stored canine blood products (Brownlee et al. 2000; Ekiz et al. 2012; Graf et al. 2012). Leukoreduction attenuates some post-transfusion inflammatory responses seen after transfusion of 21-day-old stored PRBCs in healthy dogs compared to dogs transfused with non-leukoreduced stored PRBCs (McMichael et al. 2010). Pro-inflammatory monocyte chemoattractant protein-1 increases after transfusion of 28-day-old PRBCs to healthy dogs irrespective of whether blood is leukoreduced or not, indicating that not all inflammatory responses induced by transfusion of older stored blood products are attributable to leukocytes and their products (Callan et al. 2013).
The available evidence suggests that it would be beneficial to leukoreduce all stored RBC products, but further studies are needed to assess whether the benefits outweigh the financial cost of this procedure, which is up to $30 per blood collection depending on the leukocyte reduction filter used. More information regarding leukoreduction techniques can be found elsewhere in this textbook (see Chapter 17).
Indications
With the availability of component blood products, the use of RBC products in managing various conditions in dogs and cats can be tailored to their specific requirements. RBC products are predominantly used to manage anemia. In the case of severe acute hemorrhage, as can result from trauma, surgery, gastrointestinal hemorrhage, or neoplasia, dogs and cats typically require circulating volume replacement with crystalloid and/or colloid fluid therapy. After fluid replacement, if hemorrhage has been severe or is ongoing, it might be appropriate to administer WB (Callan et al. 1996; Castellanos et al. 2004; Klaser et al. 2005). In addition to RBCs, WB provides plasma proteins to improve oncotic pressure, which can be decreased with dilution from crystalloid fluid administration. Anemia resulting from hemorrhage can also be managed with PRBC transfusions in combination with plasma products if WB is unavailable.
Coagulopathies and disorders of hemostasis such as disseminated intravascular coagulation, congenital or acquired clotting factor deficiencies, vitamin K deficiency, or severe cirrhotic liver disease can be managed with FWB to improve the anemia and provide clotting factors to treat the coagulopathy. However, administration of plasma products alone might be more appropriate if the anemia is not severe (Kerl and Hohenhaus 1993; Weingart et al. 2004). Animals with acute hemorrhage due to severe thrombocytopenia might also benefit from FWB transfusion. The platelets provided in FWB are unlikely to increase the measured platelet count or the numbers of platelets seen during blood smear examination, but might be sufficient to reduce or stop bleeding temporarily (Helm and Knottenbelt 2010).
WB has previously been advocated for managing severe hypoproteinemia, but this can result in circulatory volume overload, as well as unnecessary exposure to RBC antigens; therefore, administering plasma products, albumin, or artificial colloids is probably more appropriate (Callan et al. 1996; Castellanos et al. 2004).
Hemolysis can result from immune-mediated disease, infectious disease, oxidative erythrocyte damage, neoplasia, drug reactions, and genetic abnormalities (Figure 3.7). PRBC administration is more appropriate than WB transfusions for managing severe regenerative anemia due to hemolysis, as these animals usually have normal or increased circulating blood volumes, but lack oxygen-carrying capacity (Castellanos et al. 2004; Kisielewicz et al. 2014). Non-regenerative anemia, as develops with chronic inflammatory disease, iron deficiency, bone marrow disorders, infectious diseases, drug reactions, and ineffective erythropoiesis, is also best managed with PRBC products to avoid circulatory volume overload (Castellanos et al. 2004; Klaser et al. 2005; Kisielewicz et al. 2014).

Figure 3.7 This dog with severe scleral and mucous membrane icterus was diagnosed with immune-mediated hemolytic anemia and admitted to hospital to receive a packed red blood cell transfusion. (Image courtesy of Marie K. Holowaychuk, DVM, DACVECC.)
With recent increases in the availability of feline blood components, considerations of whether to use FWB or PRBC should apply to both dogs and cats.
Deciding when to transfuse
Because there are potential risks of transfusion reactions and disease transmission, as well as significant costs and difficulties in acquiring blood products, transfusions should be restricted to dogs and cats that would definitely benefit from them (Howard et al. 1992; Callan et al. 1996; Prittie 2003; Wardrop et al. 2005). Universal, standardized, and objective guidelines for transfusion triggers to determine which animals might benefit from RBC transfusion have long been sought after in both human and veterinary patients, but remain ambiguous despite clinical and experimental studies (Schwartz et al. 1981; Viele and Weiskopf 1994; Herbert et al. 1999; Carson et al. 2002).
Human studies have shown similar outcomes for patients with restricted transfusion triggers (HGB < 7–8 g/dL [70–80 g/L]) compared to those with liberal transfusion triggers (HGB < 10 g/dL [100 g/L]), suggesting that a restrictive transfusion trigger strategy should be considered (Herbert et al. 1999; Carson et al. 2011). The former study aimed to maintain the HGB concentration between 7 and 9 g/dL (70 and 90 g/L) versus 10 and 12 g/dL (100 and 120 g/L) in the restrictive and liberal transfusion trigger groups, respectively, with significantly lower mortality in the restrictive group (Herbert et al. 1999). This could be extrapolated to dogs and cats whereby achieving a packed cell volume (PCV) or hematocrit (HCT) within the reference range is unnecessary and might even adversely affect morbidity and mortality. In other human studies, morbidity and mortality remained low provided that HGB remained >7 g/dL (70 g/L), but increased substantially when HGB was <5 g/dL (50 g/L) in patients who refused blood transfusion for religious reasons (Viele and Weiskopf 1994; Carson et al. 2002). Conversely, an experimental study found that a HGB concentration as low as 5 g/dL (50 g/L) was tolerated in healthy euvolemic people without signs of impaired oxygenation (Weiskopf et al. 1998). A similar model involving healthy euvolemic dogs showed that cardiac output and oxygen extraction remained within normal limits provided that HCT was maintained above 10% (Schwartz et al. 1981).
Altogether, it seems that a conservative approach to transfusion of RBC in human and animal patients is warranted, rather than liberally administering RBC products. Although the consequences of liberal transfusion strategies or the application of conservative versus liberal transfusion triggers have not yet been studied prospectively in veterinary patients, a retrospective study in dogs revealed that transfusion of canine PRBC units at higher pre-transfusion PCVs was associated with increased mortality, possibly secondary to increased risk of transfusion-related complications (Holowaychuk et al. 2014). It is prudent that clinicians carefully consider the necessity of administering PRBC products to animals prior to instituting the transfusion.
Factors to be considered when deciding the necessity of transfusion for anemic animals include evaluating the degree of anemia based on HCT or PCV, and assessing markers of impaired cellular oxygenation such as increased lactate concentration. Clinical examination findings suggestive of severe anemia or shock such as tachycardia, tachypnea, pale mucous membranes (Figure 3.8), bounding or weak peripheral pulse quality, and reduced mentation and exercise tolerance must be assessed (Kerl and Hohenhaus 1993; Prittie 2003; Kisielewicz et al. 2014).

Figure 3.8 Pale mucous membranes are one of the clinical examination findings that can suggest the presence of moderate to severe anemia.
Scoring systems
Importantly, assessing HGB concentration or HCT alone does not account for the chronicity of anemia and the presence of adaptive mechanisms that might preclude transfusion (Prittie 2003). Previously, a “transfusion need scale” and more recently an “anemic dog clinical assessment score” (ADCAS) have been reported to assist with decision making (Kerl and Hohenhaus 1993; Kisielewicz et al. 2014). The ADCAS involves assessing five variables (mucous membrane color, pulse quality, heart rate, respiratory rate, and mentation and exercise tolerance) in euvolemic anemic dogs (Kisielewicz et al. 2014). The higher the score (out of 12), the more likely it is that the dog will benefit from a blood transfusion (Table 3.2). Despite these guidelines, consensus dictates that each anemic animal should be considered individually in terms of the severity and chronicity of anemia, as well as the underlying diagnosis or concurrent diseases. Clinical judgment alongside assessment of a combination of clinical and laboratory variables provides a comprehensive approach.
Table 3.2 Anemic dog clinical assessment score (ADCAS)
Normal (0) | Mild (1) | Moderate (2) | Severe (3) | |
Mucous membrane color | Salmon pink | Slightly pale | Moderately pale | Severely pale |
Pulse quality | Normal | Bounding | Weak | – |
Heart rate (beats/minute) | 65–109 | 110–140 | >140 | – |
Respiratory rate (breaths/minute) | 15–24 | 25–40 | >40 | – |
Mentation/exercise tolerance | Bright, walking | Quiet, able to walk | Lethargic, able to stand | Lethargic, unable to stand |
Preparing for transfusions of RBC products
After determining that a dog or cat requires a blood transfusion, Box 3.1 provides a brief guide as to how to proceed with a PRBC transfusion and is applicable to both species.

Full access? Get Clinical Tree
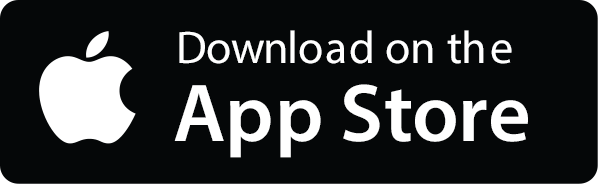
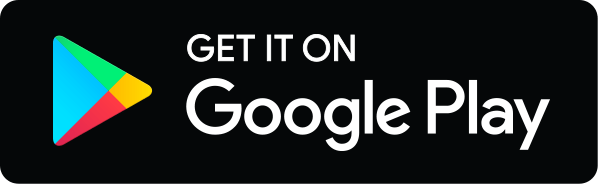