, Monika Hassel2 and Maura Grealy3
(1)
Centre of Organismal Studies, University of Heidelberg, Heidelberg, Germany
(2)
Spezielle Zoologie, Universität Marburg FB Biologie, Marburg, Germany
(3)
Pharmacology and Therapeutics, National University of Ireland Galway, Galway, Ireland
10.1 Positional Information and Generation of New Patterns
10.1.1 How Are Cells Enabled to Differentiate Well-Organised and in Accordance with Their Location? We Develop Working Hypotheses
We have discussed in the previous Chap. 9 the problem of how cells can behave in accordance with their location in the whole embryo. Here they have to construct nerve tissue, there to form muscle, and over there manufacture a skeletal element. But the DNA of the nucleus does not contain information about where a cell is at a given moment.
Two basic mechanisms ensure that at the end of development the various cell types can be found at their correct place:
1.
The cells are assigned a distinct differentiation program in their location by local influences or by their provenance; they might have inherited cytoplasmic determinants from their mother cell. During or after termination of differentiation they actively search for the place to settle temporarily or permanently. Examples are the melanocytes of our skin, nerve cells of the peripheral nervous system, the cells of the immune system or the germ cells (Summary in Chap. 15).
2.
More often cells choose their ‘profession’ in accordance with their residence and take a differentiation pathway correspondingly. It is the local environment that tells them what should be done at this particular place. Position-pertinent cell differentiation is always launched from outside, from activating or permitting influences originating in the cell’s exterior.
In Chap. 9, we already became acquainted with two sources of guiding cues: (1) External cues: Information comes from the external physical conditions: gravity, light, for example. In animal development, unlike development in plants, the influence of external physical cues on patterning appears to be restricted to very early embryonic development. (2) Maternal cytoplasmic determinants stored in the oocyte, for instance mRNA coding for transcription factors. (3) Now in this Chapter we add cell interactions and concerted behaviour as main and sustained means to create new patterns. In the majority of embryos, most probably of all animals, there is no detailed system of regional plasm in the egg, yet neither is there complete cytoplasmic homogeneity. There is instead some system consisting of a few reference points to which developmental events throughout the embryo are related. Development proceeds through interactions between cells which may act variously as the source, destroyer, transmitter or receiver of developmental signals. The most direct sort of interaction is embryonic induction. Signals provided by neighbours which determine the fate of cells formatively, are known as inductive signals (inductors) or as permitting signals; these are dealt with in the following sections.
10.1.2 In the Establishment of the Body’s Architecture New Patterns Are Created Which Are Not Yet Predefined in the Egg Cell
Pattern Formation as Result of Cell–Cell-Communication
As stated above the pattern of cytoplasmic determinants can only be a first guide for orientation. Think of a Hydra: by budding, the parental animal gives rise to hundreds of offspring; hundreds of offspring give rise to thousands of offspring and so forth, until after thousands of years vast numbers of polyps have been produced without intervention of an egg cell and sexual reproduction. How could the detailed development and shape of all these hydras be specified by cytoplasmic determinants laid down in the one original egg cell many years ago? Or imagine apple-trees of the variety Golden Delicious. Trees all over the world have been grown by grafting and are the cloned offspring of one egg cell that existed decades ago. How could the pattern of cytoplasmic determinants in the founder egg cell determine in advance the exact pattern of all these trees with all their branches, leaves and blossoms?
Pattern formation is a reproducible “epigenetic” accomplishment. Epigenetic refers to processes that are determined by events ‘above’ (Greek epi = on, above) the level of genetic information. (Since in recent years the expression “epigenetic” has got a very restricted meaning, pointing to certain chemical modifications of the DNA and associated proteins (chromatin, Sect. 12.5) we henceforth will follow this usage and confine the term “epigenetic” to posttranslational modifications of the chromatin.) The processes decisive for pattern formation are interactions of cells with other cells or with extracellular substrates (extracellular matrices). (A general synopsis is given in Figs. 2.8 and 11.1–11.4.) Of course, genetic information is required to produce those molecules which mediate cell interactions. For example, genes specify the signal molecules that can be exposed on the cell’s surface or secreted into the surroundings, and receptors that can be used to receive and recognize such signals. But all these interactions are significantly determined also by non-genetic principles such as the degree of hydrophilicity or lipophilicity of the interacting molecules and the physical laws of diffusion or the physical forces of adhesion.
10.1.3 Cell-Cell Interactions Bring About Physical Morphogenesis and Continue Maternally Arranged Steps of Determination
Among the first molecules made by the embryo, produced utilizing maternal mRNA or its own zygotic genes, are molecules enabling strong adhesion between the cells. In the mouse embryo this becomes visible under the microscope. In the 8-cell embryo the process of compaction takes place (see Fig. 5.29b): cells adhere strongly along their contact surface. Synthesis of special cell adhesion molecules (CAM) results in large-area adhesion. In the blastocyst of mammals, and likewise in the blastula of amphibians or sea urchins, cell adhesion molecules confer an epithelial organization to the outer cellular layer. Changes in the local structure of the cells with such molecules, together with secreted lubricants, enable cell movements in gastrulation. The mechanical forces generated with such molecules are dealt with in Chap. 14; they serve the physical creation of shapes during the entire embryonic development. An important class of physically acting cell adhesion molecules is known as cadherins, molecules anchored in the cell membrane and protruding into the extracellular space. Other membrane-associated molecules mediate signal transfer thus enabling locality-pertinent behaviour as explained below in Sect. 10.2.
10.1.4 In the Embryo Positional Information Is at Work. The Effect It Takes Is Demonstrated by a Classic Transplantation Experiment
The term positional information was coined by Lewis Wolpert (see Chap. 1, Box 1.1). In terms of content the idea was proposed by Hans Driesch (see Box 1.1 and Sect. 4.1). The experimental verification was presented by Hans Spemann. In a famous experiment which addressed the question of positional information (Spemann: “development according to location”) non-determined, prospective epidermis of the belly region was transplanted to the area fated to become the mouth region of a newt. The transplant formed mouth and teeth according to its new location (but horny teeth according to the genetic potency of the donor; tadpoles of frogs have horn teeth and not teeth of dentin as do salamanders! (see Fig. 5.12).
How positional information is encoded is a matter of dispute, and no generally accepted theory exists, simply because there is no single system providing such information. Some speculative proposals are discussed in Box 10.2. Compared to such speculative models the actual examples discussed in the following sections show that in fact positional information is drawn from various sources.
10.2 Pattern Formation by Exchange of Signal Between Adjacent Cells: Lateral Inhibition and Lateral Help
With termination of the first cleavage, in Drosophila with the formation of the cellular blastoderm, further processes of pattern formation rely on signal exchange between the cells and mutual agreements between neighbours. Such agreements are found even in classic “mosaic embryos” such as Caenorhabditis elegans (Sect. 4.4) and the ascidians (Sect. 4.7). Here we refer to two examples in the development of Drosophila. Of general significance are neighbourly interactions termed lateral inhibition (a term borrowed from the neurophysiology of vision) or lateral help. Examples are given in the following sections.
10.2.1 Nerve Cell or Epidermis, That Is the Question. The NOTCH/DELTA System Effects Binary, Alternative Decisions Through the Principle of Lateral Inhibition
When the egg of Drosophila has reached the stage of cellular blastoderm and gastrulation commences through the ventral primitive groove (see Fig. 4.36) the cells of the blastoderm compartmentalize into three groups:
Along the ventral midline cells which switch on the genes twist and snail immerse into the depth of the embryo to give rise to mesodermal tissue (e.g. muscles).
The cell bands adjoining on the left and right side also have a tendency to immerse into the interior to become there neuroblasts and construct the ventral nerve cord.
The residual cells of the blastoderm should stay on the surface, adopt the task of epidermoblasts and form the epidermis.
The segregation of the neuroblasts from the epidermoblasts requires a process of decision because in the border area the cells are still undecided and tend to oscillate. How is the decision made? Initially, all of the cells are nearly equivalent and have the potential to become either epidermis or nervous system, although in the ventral side there is an initial bias for becoming neuroblasts. But the cells are not yet irreversibly committed to one path or the other. If transplanted into a more dorsal position, ventral cells adopt the features of a dorsal cell and participate in forming the epidermis. Likewise, if displaced ventrally, prospective epidermal cells comply with the social rules and instructions valid at the new location, and become nerve cells.
Both groups segregate definitively from each other by the exchange of signals while they still are more or less clustered in intermingled groups. In this process of separation signal molecules exposed on the surface of the cells are of particular significance (Fig. 10.1). One of the surface molecules is encoded by the gene Notch. When the NOTCH protein is defective due to a mutation in the Notch gene, all cells develop into neuroblasts instead of a mixture of neuroblasts and epidermoblasts. NOTCH interacts with another membrane protein exposed by neighbouring cells and encoded by the gene delta. Both NOTCH and DELTA are transmembrane proteins containing in their extracellular moiety repetitive sequences, called EGF repeats because they had previously been found in the epidermal growth factor EGF. The extracellular domains of the NOTCH and DELTA proteins exposed by adjacent cells bind mutually and mediate a loose cohesion; but they have multiple additional functions. In the present example NOTCH plus DELTA serve the supression of the neurogenic bias in the epidermoblasts. In the recent literature DELTA is classified as an inhibitory signal, NOTCH as its receptor. This classification was chosen because upon mutual attachment and activation, an intracellular domain is cleaved from the NOTCH molecule, enters the nucleus of the cell and acts as gene controlling transcription activator. This cleaved off intracellular domain is abbreviated NICD (Notch Intracellular Domain).
Activating of NOTCH signalling is needed by the cells to become epidermis. In order to reach this end, the NOTCH receptor – once stimulated by the DELTA ligand – initiates via its NICD a cascade of events resulting in the switching on of a gene complex called enhancer of split (ESPL-C). This complex suppresses the inherited neurogenic bias and confers the ability to adopt instead epidermal features. When NOTCH is defective the intrinsic preference to take the neurogenic path is not properly suppressed. DELTA is used as a competition suppressing signal by cells being slightly in advance in taking the neurogenic path themselves. It enables them to suppress the neurogenic tendency in their neighbours by showing them their DELTA-decorated cell surface and thus to induce the NOTCH pathway in the neighbour. To realize their own neurogenic potency, the DELTA-decorated cells must continue to express another complex of genes, called achaeta scute-complex ( AS-C).
The two cell-type programming gene complexes (the epidermis-specific ESPL-C and the nerve-cell specific AS-C) cannot be simultaneously activated: their expression is mutually exclusive. Eventually, the differently programmed cells separate mechanically. The nerve cell precursors are shifted into the interior of the embryo (Fig. 10.1), whereas the epidermal cells remain outside and secrete the shielding cuticle.
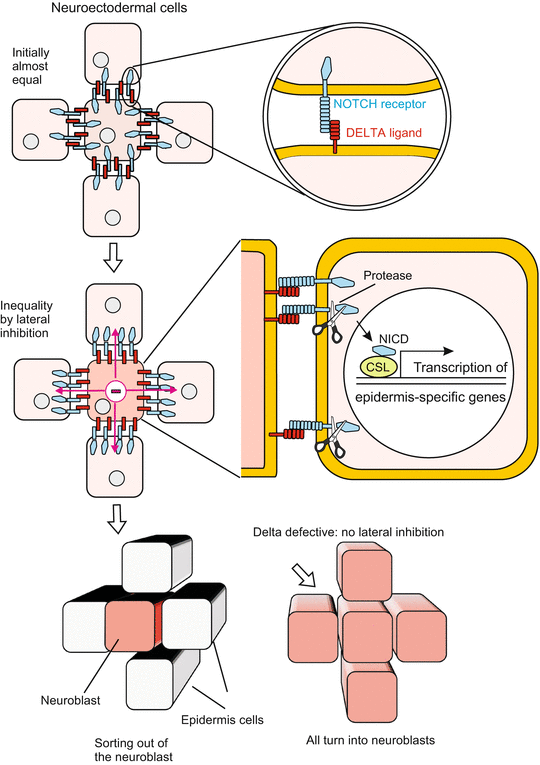
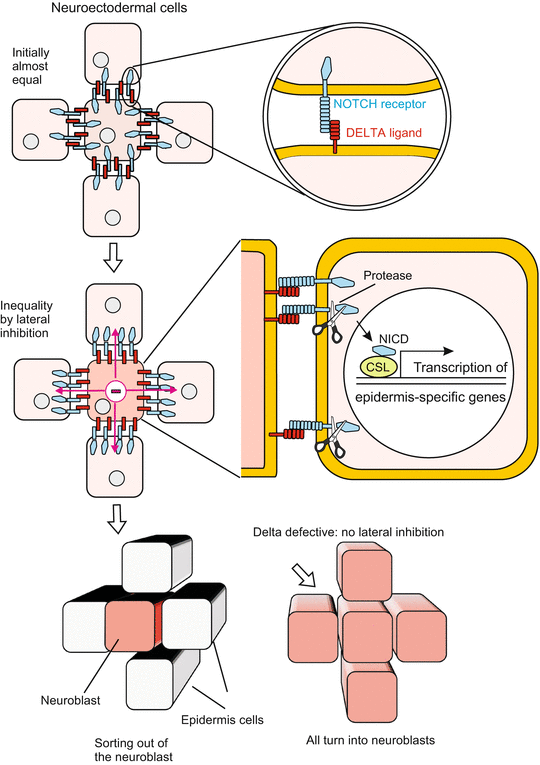
Fig. 10.1
NOTCH/DELTA signalling. Segregation of a neuroblast from epidermoblasts in the ventral blastoderm of a Drosophila embryo. Initially, in the ventro-lateral blastoderm all cells are (almost) equivalent, with a bias toward becoming neuroblasts. This bias is indicated by the presence of transcription factors that are encoded by the neurogenic achaete–scute gene complex. Local inhibitory cell interactions mediated by cell surface proteins restrict the expression of the neurogenic factors to one cell, the neuroblast. The surface proteins in question NOTCH and DELTA are encoded by the Notch and delta genes, whereby NOTCH is viewed as receptor and DELTA as its ligand. Upon activation of Notch signalling, the intracellular domain NICD of NOTCH is cleaved off, enters the nucleus and acts as gene regulatory transcription factor. The particular cell that is fated to become a definitive neuroblast is thought to be specified by random fluctuations in some signalling activity. Once a definitive neuroblast is specified, it suppresses the neurogenic bias in adjacent cells by DELTA/NOTCH-mediated signalling. Finally, the neuroblast is singled out and delaminated into the interior of the embryo. In Notch as well as in delta null mutants all cells of the ventral blastoderm continue to express the achaete–scute gene complex and adopt the neuroblast cell fate, which leads to lethal hypertrophy of the nervous system
We still shirked answering the question: How is the decision reached which cells are allowed to enter the interior of the embryo becoming definitive neuroblasts and which cells are destined to stay outside forming epidermis? The hypothesis presented as an explanation refers to a basic principle in biology: Initially minor differences are enhanced by competition between the participating cells. Lateral inhibition brings about winners and losers.
In the ventro-lateral border area where undecided cells are located all cells expose NOTCH as well as DELTA. The future neuroblasts possess slightly more inhibitory DELTA molecules, either due to their position, provenance or chance. Presenting a few more DELTA is a slight starting advantage in competition: the neighbour is slightly more suppressed than the cell presenting a higher amount of inhibitory signals. As a consequence of received inhibition, in the neighbour expression of DELTA is reduced while NOTCH molecules for reception of the inhibitory signal remain preserved. Thus a weak difference experiences progressing self-enhancement by positive feed back. The neighbours remain susceptible for inhibitory signals (because they retain NOTCH), but their ability to produce inhibitory signal declines (because DELTA expression is more and more curtailed). Eventually they will be the losers.
Reaching alternative decisions using the NOTCH/DELTA system is not restricted to this particular case. Genes coding for members of the NOTCH and DELTA families were found in all animal organisms in which homologous genes have been searched for. Also in vertebrates segregation of future neuroblasts and epidermoblasts along the border of the neural plate is mediated by a NOTCH/DELTA system (see Fig. 16.10). Moreover, the system plays a role not only in the segregation of future nerve cells and epidermal cells, but in many processes of alternative decisions when boundaries are drawn between territories of different destiny. There exist several different isoforms of NOTCH as well as of DELTA enabling different fields of application; an example will be presented in Fig. 10.25.
10.2.2 Here Is the Question: Photoreceptor No. 7 or Sevenless? A Case of Lateral Help
The compound eye of Drosophila comprises about 800 small eyes called the ommatidia. Each ommatidium is composed of 20 cells. Of these, four cells are used to construct the dioptric apparatus consisting of lens and crystal body – in the following “lens” for short – while eight cells are induced to become photoreceptors. These cells are arranged in a precise, consistent pattern.
In cross sections of the eye always only 7 photoreceptors are seen, R6 to R7 forming a circle and R7 or R8 taking the centre position. R7 and R8 are arranged in tandem order along the longitudinal axis, R7 taking the top and R8 the bottom position (Fig. 10.2). All photoreceptors form with their hem of microvilli (rhabdomeres) the light absorbing mid zone of the ring.
The first photoreceptor to develop is the central cell, called R8; it is the BOSS cell. BOSS refers to the gene bride of sevenless. The protein encoded by this gene is exposed on the surface of R8 and shown to the adjacent cell R7 as an identity card. R7 in turn is equipped with a membrane-associated receptor to check the identity card of its neighbour. The receptor is a transmembrane tyrosine kinase derived from the gene sevenless. If photoreceptor R8 is unable to offer the correct signal due to a mutation, or R7 is unable to recognize the message, the cell R7 will not develop properly. Instead of developing into a photoreceptor, it makes a lens. Lens cell is the default option if the photoreceptor program is not turned on (Fig. 10.2).
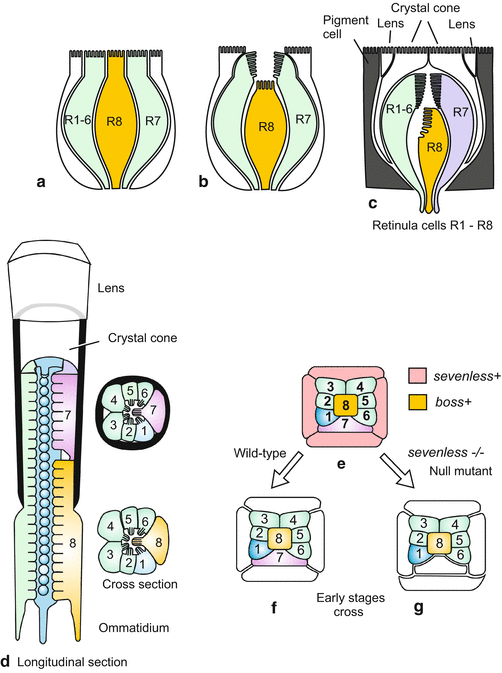
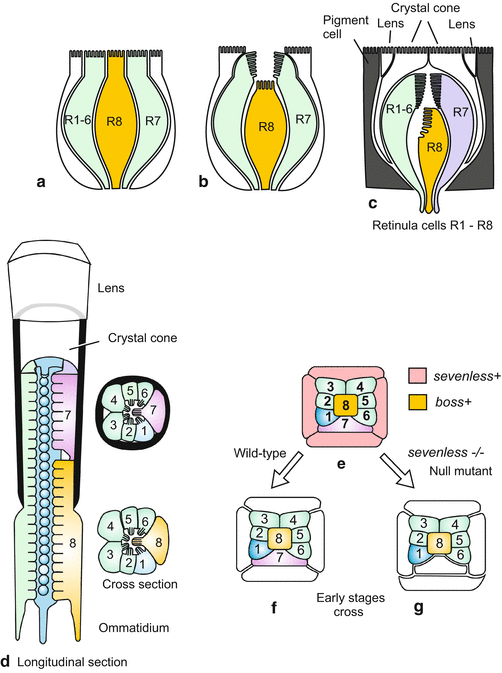
Fig. 10.2
(a)–(g) Pattern formation and commitment in the Drosophila ommatidium by close-range interactions. The photoreceptor R7 is formed only when the cell R8 expresses the gene boss +, and the cell number 7 the gene sevenless +. The proteins coded by these genes are exposed on the cell surface and presented to the neighbours. If either of the two gene products is absent or defective, photoreceptor R7 is replaced by an additional lens cell. Lens cell formation is the default option if the photoreceptor program is not turned on
These two examples of neighbourly inhibition or help could well also be classified as induction phenomena. This topic is addressed in the following sections.
10.3 Embryonic Induction and the Spemann Organizer
10.3.1 The Principle of Embryonic Induction: Cells Emit Signals into Neighbouring Areas
Induction is a matter of great significance. In induction, signal-emitting (inducing) cells instruct or permit signal-receiving (responding) cells to take a specific developmental path. Such instructive or permissive interactions are among the fundamental events leading to the cooperative formation of tissues and organs. The term ‘induction’ is used in biology in diverse contexts (e.g. induction of an enzyme refers to turning on transcription of a gene whose product is an enzyme). To distinguish between the various meanings, developmental biologists often speak of embryonic induction (although inductive influences are not restricted to embryogenesis). In terms of embryology induction embraces emission of a development controlling signal by (inducing) emitters and the response by the signal receiving cells
Transfer of inductive information can be accomplished by signal molecules exposed on the cell surface. In Sect. 10.2 we gave two examples: in the Drosophila ommatidium the cell R7 is instructed to develop into a photoreceptor by the adjacent photoreceptor R8, and cross talk between neuroblasts and epidermoblasts in Drosophila facilitates their segregation from each other. Both are examples of induction. However, the term is usually associated with signal molecules capable of spreading into a larger area by diffusion, though spread by diffusion is not a strict criterion defining inductive molecules.
By transplanting cells or pieces of tissue, and by eliminating putative emitters of inductive signals, inductive interactions have been revealed in all animal organisms that have been investigated with adequate methods. Attempts to cause the development of structures in the wrong places have been successful in sponges, hydrozoans, sea urchins and vertebrates. Even in embryos previously considered to represent a genuine mosaic type of development, such as Caenorhabditis elegans and ascidians, more and more inductive interactions between adjacent cells are being detected.
A structure artificially induced in the wrong location is called ectopic. The first discovery of ectopic induction was in 1909 in Hydra: a small piece taken from the mouth cone (hypostome) or the subtentacular region and inserted into the gastric region organizes the formation of a second head and body axis (see Fig. 10.19). For many years inductive phenomena have been most extensively studied in amphibian embryos (Sects. 5.1 and here 10.3.3). These famous experiments were paradigmatic for similar experiments in other animal embryos.
10.3.2 The Receiver Must Be Competent
In radio broadcasting a message is heard only if the receiver is tuned to the transmitting station sending the message. Likewise, in embryonic induction the response to a signal requires competence of the recipient cells, where competence is defined as the ability to respond to an inductive signal. The acquisition of competence implies the production and display of receptors (‘antennae’) for the inducing signal. Competence often is restricted to a narrow window of a few minutes or hours.
10.3.3 The Spemann Organizer and the Induction of Heads and Trunks in the Vertebrate Embryo
Not only in the simple Hydra, also in the highly complex vertebrate embryo induction of an additional head, and even of an additional, almost entire embryo can be brought about by transplantation of only a small piece of tissue. As we have seen in the Sect. 5.1 in the late blastula and early gastrula there is a small area, known as the upper blastopore lip or organizer, which by itself will form the anterior segment of the notochord (plus prechordal plate), has been shown to be an outstanding signal emitter. In 1924 Hans Spemann and coworkers drew the attention of the scientific world to the astonishing inductive power of the upper blastopore lip of the amphibian gastrula: a blastopore lip inserted into a blastula at an ectopic site organizes the surrounding cells into a supernumerary gastrulation, resulting in a secondary body axis.
The equivalent signal emitter is called embryonic shield in fish, Hensen’s node in birds, and simply node in mice. All these structures are homologous; they correspond to each other both in terms of evolution and function. Even a small transplant taken from the organizer of any vertebrate can release the development of complex structures such as heads and trunks in any other vertebrate. The designation “organizer” was chosen by Spemann because the upper blastopore lip prompted its surroundings to initialize a second site of gastrulation culminating in the development of a second embryo (Siamese twin) with head and trunk (see Fig. 5.13).
In the amphibian embryo the organizer is established at a site located diagonally to the sperm entrance. In the course of the cortical reaction components of the WNT signalling system had been relocated to this diagonally located point. These components include mRNA for WNT signalling molecules and components of the associated signal transduction cascade such as Dishevelled (Dsh) and β-CATENIN (Fig. 10.3). The action of these components turn this area into the organizer. When, for example, the mRNA for β-catenin in the organizer area, or beforehand at the vegetal egg pole, is inactivated by anti-sense RNA or UV irradiation organizer activity fails. On the other hand, injection of mRNA for β-catenin (or β-CATENIN protein) into blastulae rescues the emitter. If injected ectopically, β-catenin mRNA leads to the establishment of a second, ectopic organizer (see Fig. 5.17). Oocytes and young embryos are well suited to such experiments because the translation machinery is ready to work with high efficiency and even ectopic mRNA is translated into much protein.
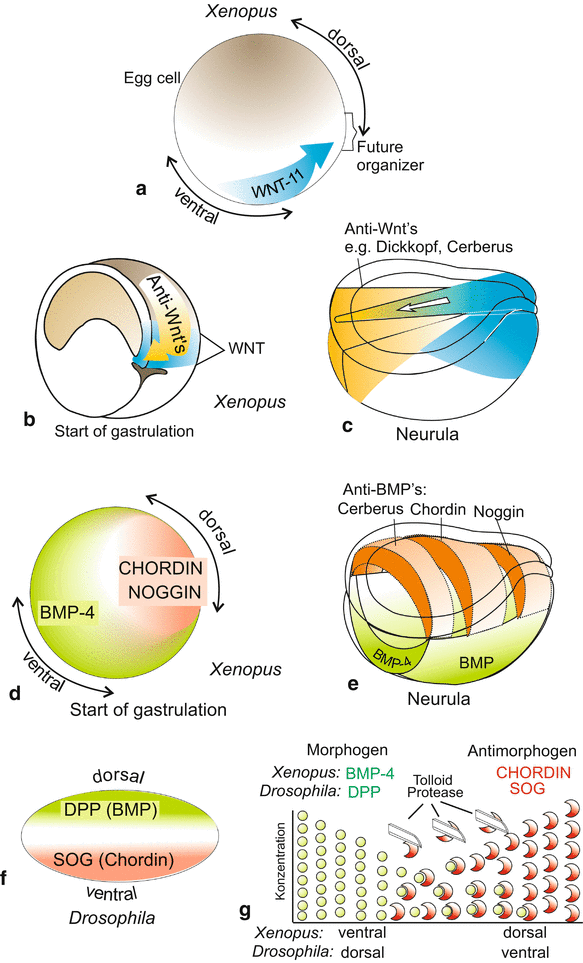
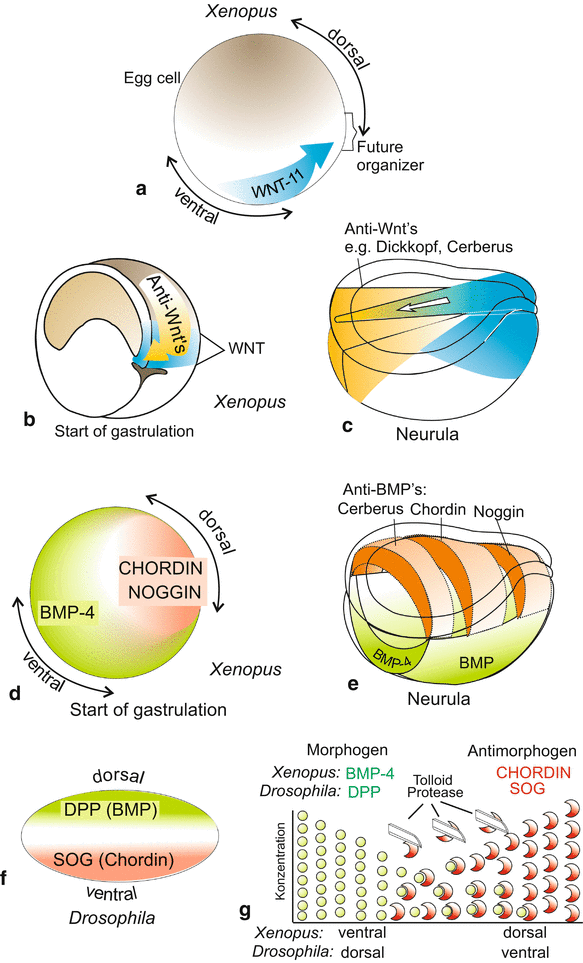
Fig. 10.3
(a)–(g) Morphogens in the early embryo (shortly before gastrulation starts) in Xenopus and Drosophila by comparison. (a) Induced by maternal mRNA stored in the oocyte, a signal generator known as Spemann organizer is established. Already before cleavage the process of cortical rotation, released by the sperm’s entry, displaces maternal mRNA from the vegetal egg pole to the site of the future organizer. These translocated factors comprise, besides mRNA for WNT-11, some more components of the WNT pathway, in particular β-catenin. (b) Gastrulation movements shift cohorts of cells which produce anti-WNT morphogens using their zygotic genes, into the interior of the embryo. These cohorts of cells become the prechordal mesoderm and the anterior roof of the archenteron. (c) In the organizer a secondary WNT signalling system (blue) is set up producing mainly WNT-8. In the region of the prechordal mesoderm plus anterior intestinal roof anti-WNT’s are produced which neutralize WNT-8 and thus stabilize the head-tail body axis. (d)–(f) The antagonistically acting morphogens BMP-4 and CHORDIN in Xenopus are sequence homologous to the corresponding antagonistic morphogens DPP and SOG in Drosophila. They are expressed in patterns inverted by 180°, corresponding to the apparently inverted anatomical body plan of vertebrates and arthropods. (g) In the subframe beneath it is schematically illustrated how the antagonistic morphogens diffuse towards each other and associate in the overlap area forming heterodimers. Proteases contribute to the sharpening of the distribution pattern. BMP (Bone Morphogenetic Protein) is homologous to DPP (DecaPentaPlegic). SOG (ShOrt Gastrulation) is homologous to CHORDIN
In the conventional scheme of the canonical WNT signal transducing pathway β-CATENIN takes a downstream position (see Fig. 11.6). In spite of this seemingly subordinate position it can release the entire signalling system because β-CATENIN is a transcription factor and among its target genes are Wnt genes and the genes of the other components of the pathway, including its own β-catenin gene. The cart is put before the horse.
In birds and mammals, the part of the amphibian upper blastopore lip is played by Hensen’s node (node in mammals) at the anterior end of the primitive groove. Hensen’s node is homologous to the upper blastopore lip, is endowed with similar inductive power when transplanted into an amphibian blastula, and generates similar signal molecules. In addition, all these “organizers”, the amphibian upper blastopore lip and the node in birds and mammals, express certain homeobox containing genes such as goosecoid and siamois. These genes confer the ability to produce inducers. Note, however, that the final structures induced by these organizers, such as a forehead or a second head-trunk structure, can only be initiated by the primary inducing signals. Many events must follow to realize such complex structures.
10.3.4 The Spemann Organizer Is Subdivided in Head and Trunk Organizer
The statement of this headline has been first shown to be valid in the amphibian embryo but is valid in other vertebrate embryos as well. As in Sect. 5.1 described in detail, in gastrulation the organizer area successively moves through the blastopore into the interior. Already while streaming towards the blastopore it is protracted and lengthened and subdivided in two sub-areas:
a head organizer, which in the early gastrula is located near the blastopore lip, and
a trunk organizer, which is located behind and above the head organizer (see Fig. 5.14).
When the cell material that rolls around the blastopore lip into the interior has become the middle stripe of the roof of the archenteron and thus the primordium of the notochord, the action ranges of the head and trunk organizers are well separated. The anterior notochord primordium induces head, the posterior primordium induces trunk. Several factors have been identified exerting corresponding inductive powers:
1.
Head formation: The effect of the head organizer is in all vertebrates mediated by a cocktail of cysteine-rich proteins with CERBERUS, DICKKOPF-1, FRIZBEE-b and further secreted proteins as ingredients. When one injects the mRNA of, for instance, dickkopf (and simultaneously suppresses the inhibitory BMP-4), a complete head emerges, but no trunk (see Fig. 5.17). In sum head-formation supporting factors are antagonists of the canonical WNT-8 signalling system (Fig. 10.3, see also Fig. 5.18).
This statement may be confusing, since the organizer includes head organizing power and is established, as said above, by means of a WNT-system. Subsequently this topographical region is a source of WNT-8, now said to prevent head formation. Apparently, this region has the capacity not only to produce WNTs but also antagonists which bind and neutralize WNTs. To resolve this apparent contradiction and to understand anti-WNT activity we have to take into consideration the fact that the cells of the upper blastopore lip are not stationary but flow. The cells that pass the lip at the start of gastrulation occupy at the end of gastrulation the anterior roof of the archenteron forming thereafter the prechordal mesoderm. Here they appear to release the anti-WNTs, enabling head formation and aiding in establishing a gradient of WNT-8 extending from posterior to anterior.
2.
Trunk formation. The effect of a trunk organizer is brought about by a collection of other cysteine-rich proteins, in particular of NOGGIN, CHORDIN, FOLLISTATIN and NODAL-related 3. When in the Xenopus egg stored mRNA for these inducing factors is destroyed by UV irradiation and subsequently mRNA of, for instance, noggin is injected into the prospective belly region of the blastula, the embryo develops back structures such as notochord and neural tube. But a head is lacking. NOGGIN induces structures of the trunk only. Trunk-inducing factors are antagonists of the BMP factors (BMP-4, BMP-2), which radiate from the opposite, ventral side of the embryo (Fig. 10.3, see also Fig. 5.18).
The interaction of WNTs with their antagonists generates double gradients with peak activity at opposite poles and running in directions reverse to each other (Figs. 10.3 and 10.4). The Wnt antagonists aid in programming head structures. By working in opposite direction the WNT and anti-WNT gradients arrange, in cooperation with other factors such as retinoic acid and diverse FGF’s, that the anterior part of the neural tube will form the brain and the posterior part the spinal cord (see also Fig. 16.1).
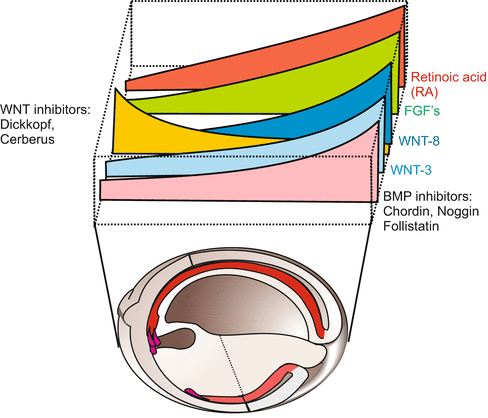
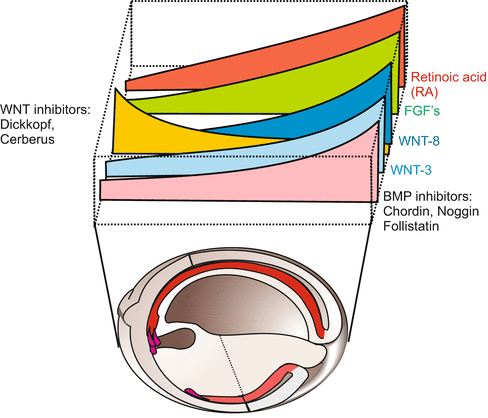
Fig. 10.4
Parallel and reverse gradients of signalling molecules and of neutralizing anti-factors in the dorsal area of a Xenopus neurula
Simultaneously and perpendicular to the WNT-/anti-WNT gradients double gradients of the factors BMP and CHORDIN are installed in reverse orientation (Fig. 10.3). This is accomplished in that the BMP and CHORDIN molecules diffuse towards the equator, and bind and neutralize each other in the region of overlap. Together the two gradient systems, the WNT/anti-WNT gradients and the BMP/CORDIN gradients stabilize irrevocably the body axes, the antero-posterior and the dorso-ventral axis.
Under aspects of evolution it is remarkable that homologues of these factors which provide for regional specification in the amphibian gastrula, are called into action also in echinoderms and Drosophila (Fig. 10.3f). The factors BMP-4 and CHORDIN in amphibians correspond with, and are homologous to, DPP (DECAPENTAPLEGIC) and SOG (SHORTGASTRULATION) in Drosophila. Like in amphibians, in the fly embryo both factors are essential for the establishment of the dorso-ventral axis but with inverse algebraic sign. Homologous factors specifying the dorsal side in amphibians specify the ventral side in the fly and conversely, homologous factors specifying the ventral side in amphibians specify the dorsal side in the fly. How this inversion might have arisen in evolution will be discussed in Chap. 22 (Fig. 22.14).
In the neurula the gradients of WNT-8 on the one hand, and BMP on the other hand, form a system of Cartesian coordinates (Fig. 10.5). The possible significance of such a system will be discussed below in the Sect. 10.5.3.
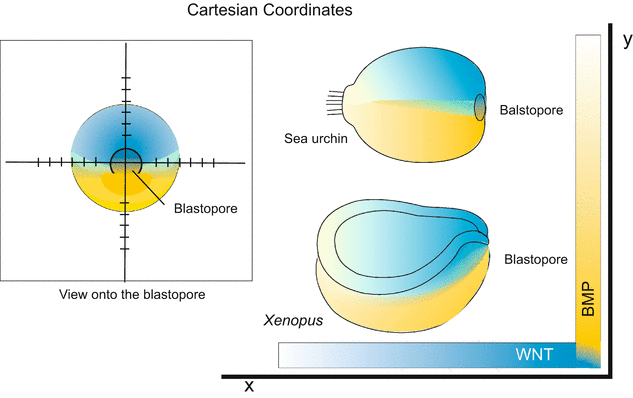
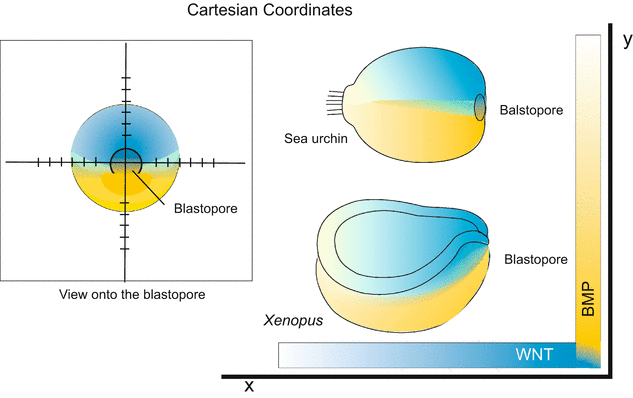
Fig. 10.5
Cartesian coordinates by perpendicular gradients of WNT and BMP, exemplified by the embryos of sea urchins and Xenopus. Both the animals belong to the deuterostomes, therefore the blastopore can serve as site of reference
10.4 Cascades of Induction and Identification of the Signal Molecules
10.4.1 Inducing Signal Molecules Are Difficult to Identify; Methods of Biochemistry and Molecular Biology Combined with Appropriate Bioassays Made the Breakthrough
Evidence for inductive events is usually deduced from transplantation studies: When host tissue touches transplanted tissue with inducing power, it responds by developing a structure which would not otherwise form. Although such experiments have been performed many times and in many organisms, identification of the inducing signals turned out to be very difficult. Embryos are tiny, surgical interventions difficult, and signal molecules present in only minute quantities, so that tons of embryos would have to be extracted to obtain chemically analyzable traces of the inducing substance.
Despite these unfavourable circumstances, impressive progress has been made in Drosophila and Xenopus. In Drosophila, mutagenesis helped identify genes for signal molecules and their receptors. Using the methods of molecular biology (Chap. 12, Box 12.2) eventually the genes of several inducing factors and receptors were sequenced, and more signal molecules are still being identified. Inducing factors and receptors can be expressed in large amounts in appropriate experimental systems such as transfected bacteria or animal cells.
In vertebrates, progress also depended on methods from molecular biology, although classic biochemical methods have also been employed with some success. The discovery of induction stimulated an intense race to identify the putative inducer. In fact the amphibian inducers proved remarkably elusive and the first were isolated only in 1987.
In the hunt for inducers appropriate bioassays are crucial. The “animal cap assay” (see Figs. 5.16 and 16.1b) by which competent but still undifferentiated tissue is used to identify the presence of inductive substances, was both the source of many pitfalls and of final success. Caps are excised from the animal hemisphere of blastulae (for decades newt blastulae) and placed in small bowls containing neutral buffer solution. Without exposure to induction factors the animal cap forms a ciliated epithelium as it is characteristic of the blastoderm of a blastula. In classical experiments the Dutch scientist P Nieuwkoop placed explants taken from different regions of the blastula near the cap. After some time of contact with added tissue, or incubation in supernatants, the caps were examined microscopically for the presence of differentiated cells. Various types of differentiated cells were found and even complex structures such as cartilage, brain tissue or eyes. Thus, the beginning was promising.
However, the hunt for inducers, in particular, the hunt for neural inducers, was soon confounded by the fact that just about any culture condition triggered neural development, an effect that became known as ‘autoneuralization’. Fortunately Xenopus caps do not autoneuralize readily or develop mesodermal cells, such as muscle cells, blood cells and cells typical of the notochord.
Such mesodermal cells were seen by Nieuwkoop when he placed tissue taken from the vegetal hemisphere onto animal caps. Hence from the added explant mesoderm-inducing factors had been released.
In the end, two lines of research converged:
The first line of research was based on hard work. From tons of incubated chick eggs and other biological sources a mesoderm-inducing substance was extracted, tested using the animal cap assay and purified. Animal caps formed mesodermal cells such as muscle cells when treated with traces of the substance. Identification was facilitated by a happy coincidence: animal caps excised from Xenopus blastulae were maintained in culture. When the medium was supplemented by certain growth factors used in the culture of mammalian cells, the caps differentiated into mesodermal cells. A similar substance was found in the supernatant of a cultured Xenopus cell line. The mesoderm-inducing substances from the mammalian and the amphibian source turned out to be related to each other. They shared a particular property, binding to heparin, with known growth factors isolated from supernatants of mammalian cells, especially with factors belonging to the FGF (fibroblast growth factor) and TGF-β (transforming growth factor beta) families. A partial sequencing of the amino acids pointed to a protein related to ACTIVIN; this protein is in fact a member of the known TGF-β family. The TGF-β prototype is produced by blood platelets and supports growth of transformed (cancerous) cells. Both FGF and TGF-β showed inductive properties. These findings suggested that other known ‘growth factors’ should be assayed for inductive properties, and that similar molecules should be sought in the amphibian embryo using molecular probes.
The second path of successful investigation began in about 1990 when the powerful methods of molecular biology became available also for Xenopus laevis, although in this tetraploid frog identification of corresponding genes by mutagenesis and positional cloning, as performed in Drosophila, is not possible. A breakthrough was achieved using the cDNA subtraction procedure. Messenger RNA extracted from organizer region was converted to cDNA and subtracted from cDNA derived from indifferent tissue (the principle of the method is explained in Box Ch12.2 and specialist books). Organizer-specific cDNA was translated and the resulting proteins obtained in quantities that allowed tests of inductive effectiveness in bioassays. In a modified and simplified bioassay the mRNA of putative induction factors is injected into uncleaved eggs of Xenopus, or into cleavage stages, at sites where presence of mRNA of this sort is not to be expected. When the mRNA is accepted by the embryo and properly translated it can become effective at the selected site. In addition, meanwhile a large array of methods is available to deactivate the endogenous (body’s own) factors, e.g. by means of antibodies, anti-sense oligonucleotides, morpholinos or RNAi (see Box 12.2).
10.4.2 The Development of an Embryo Is Based on Cascades of Successive Induction Processes and Communication by Means of Numerous Signalling Substances
A remarkable, unexpected observation was the fact that not only transplanted tissue with its numerous components but a single isolated substance is capable of releasing the development of complex structures composed of many different cell types such as a head or a trunk, and that such complex structures arise in due sequential order. When a single substance induces the development of a complex structure this is possible because the inducing signal releases a cascade of subsequent events that follow automatically like a domino effect. Thus the action of the Spemann organizer is followed by many secondary and tertiary processes of induction (for instance lens and cornea induction, Sect. 10.4.4). Yet, also before the Spemann organizer starts its work, induction processes take place.
Processes of induction before the establishment of the organizer: The embryonic development of Xenopus is governed and controlled by cascades of inductive processes. The cascade starts as early as fertilization, or at the latest in the early blastula. P. Nieuwkoop and thereafter Japanese researches have revealed the origin of the mesodermalizing induction (also called vegetalizing induction): a broad ring of equatorial cells in the blastula is specified to develop mesodermal tissues by signals emanating from the vegetal pole region of the blastula. Which molecules actually confer this effect is a matter of dispute yet. Candidates are molecules which later are produced also in the organizer, for instance NODAL and CHORDIN. In the animal cap assay these factors effect differentiation of mesodermal but also of neural fate. Whether factors such as CHORDIN evoke nervous tissue or notochord or both may be a function of concentration, or a sequence of events is launched: the factor assayed may induce axial (dorsal) mesoderm, the precursor of the notochord, and this induces nervous tissue on its part. We recall: signals emitted by the roof of the archenteron (axial mesoderm) cause the overlying animal ectoderm to develop the central nervous system.
10.4.3 Absence of Certain Factors Might Be a Signal as Well: According to a Widespread Hypothesis Ectodermal Cells Become Nerve Cells Spontaneously If Not Prevented to Follow Their Inherent Propensity
The development of the nervous system was, and still is, in the centre of interest for experimentally working embryologists. Spemann once classified the neural induction radiating from the upper blastopore lip and thereafter from the roof of the archenteron as “primary induction” when the result was a central nervous system embedded in a twinned embryo, or in a head or a trunk. He spoke of secondary induction when the eye vesicle induced a lens in the overlaying ectoderm as described in Sect. 10.4.4.
Factors that undoubtedly support neural differentiation are NOGGIN and CHORDIN. They bind and thus neutralize the ventralizing factors BMP-4 and BMP-2. BMP-4 on its part causes animal cap cells to form regular epidermis instead of ciliated blastoderm. The neuralizing NOGGIN and CHORDIN are thought to accomplish their task by binding BMP-4 in the extracellular space and overriding its anti-neuralizing effect, so that cap cells can follow their inherent propensity and differentiate into nerve cells. Thus, NOGGIN and CHORDIN would be permissive factors.
Unfortunately not all vertebrate embryos behave equally well. Already in classical arduous experiments aimed at identifying neural inducers using the assay with animal caps from newts one was confronted with the confusing experience that it was more difficult to prevent than to induce neural differentiation, quite contrary to Xenopus or the chick who do not develop neural structures spontaneously. It would therefore not be surprising when in future besides permissive factors also positively inducing factors will be found. Under discussion are various FGFs and retinoic acid.
10.4.4 The Classical Textbook Example of a Secondary Embryonic Inductive Event Is the Induction of the Eye Lens
Development of the eye (Figs. 5.21 and 16.21). In its inner ‘kernel’ the eye is a derivative of the brain and thus of the neural tube, whose development is initiated by the ‘primary’ induction emanating from the roof of the archenteron. Two bulges of the lateral wall of the midbrain enlarge, giving rise to the optic vesicles. By a process of invagination the vesicle transforms into the double-walled optic cup. The inner wall gives rise to the layers of neurons and photoreceptors, collectively called retina, whereas the outer wall will form the pigmented layer. Mesodermal cells enclose the optic cup and supplement the eye ball with the layers of the vascular choroid coat and the sclera. Among the solid structures, only the lens and cornea are still missing.
Inductive events. The optic cup comes into contact with the overlying ectoderm. In a process once classified as secondary induction, the cup stimulates the formation of an ectodermal lens placode (placode = thickening) in the right time, at the right place and of the right size. As a consequence of the induction process in a circular area of the ectoderm a lens-specific transcription factor (L-MAF) is expressed, which on its part switches on genes for lens proteins (crystallins). A circular lens placode is formed that sinks down.
As the lens placode detaches from the ectoderm, it becomes covered by the surrounding ectodermal epithelium. A tertiary signal emanating from the lens causes the covering ectodermal epithelium to transform into a translucent cornea.
There was a long-lasting scientific dispute about lens induction. Surprisingly, it was discovered that in some vertebrates, and in fact in some amphibian species, lens formation does not depend on having received an inducing stimulus from the eye cup. Lens formation takes place even when the eye vesicle is removed. This applies also for Xenopus, though the lens remains meagre in the absence of the eye vesicle. Apparently, the cells of the future optic vesicle begin to emit inducers before the optic vesicle is detectable. The finished optic cup has a subsidiary, amplifying influence which in some species is still strong and required, whereas in other species it can be omitted.
Some of the signals controlling induction and formation of the eye structures are well-known: SHH (Sonic hedgehog) defines in cooperation with BMP-4 the dorso-ventral axis of the eye; the lens forms where dorsal and ventral signals meet. FGF/WNT control later steps of differentiation. We will deal with eye development and its neural connection with the brain in Sect. 16.7.
10.4.5 Signal Molecules Such as SONIC HEDGEHOG May Be Used in Different Organisms and Organs for Different Purposes
Nature does make it not easy for the researcher or the student. On the one hand a biological function can be controlled by several synergistically acting, even non-homologous factors; on the other hand one and the same factor can be used in different locations, for different functions and in the formation of different, non-homologous structures, dependent on where and when the factor is used to convey a signal. Informative examples are the factor encoded by the gene sonic hedgehog (shh) and the lipid retinoic acid.
Sonic hedgehog
Contemporary developmental biology benefits much from molecular techniques known collectively as ‘reverse genetics’. In particular the use of heterologous gene probes (Chap. 12, Box 12.2) allows the rapid determination of whether a gene known from, say Drosophila, is also present in another organism by screening gene banks or using Drosophila sequences as heterologous probes to fish genes from genomic banks of other species. Such investigations yielded many surprising results, for example in the case of the potential signalling molecules coded by the Drosophila gene hedgehog and the signal molecules coded by corresponding genes in vertebrates, with sonic hedgehog as a prominent example.
The mutation hedgehog makes a Drosophila larva look like a hedgehog with a crew cut; the back is covered by chitinous spines. In the embryo the gene is expressed in various stripes and areas. First, hedgehog is expressed at the anterior border of the (para-)segments in those cells whose nuclei contain the transcription factor ENGRAILED (see Fig. 4.38). In other regions of the larval fly such as the imaginal discs, another variant of HEDGEHOG is produced. This variant detaches from the cell surface. HEDGEHOG is not only a signal molecule but also a proteolytic enzyme and cleaves itself into a domain remaining in the membrane and a component that floats away. The detached HEDGEHOG domain serves as a signal molecule, capable of reaching distant targets by diffusion. HEDGEHOG is used for short-distance as well as for long-distance communication in vertebrates (Danio, Xenopus; chick, mouse) also.
In vertebrates sonic hedgehog is expressed in the upper blastopore lip and subsequently in the derivative of the invaginated lip cells, the notochord. The notochord presents SONIC HEDGEHOG protein as an inducing cue to the adjacent neural tube in a contact-dependent manner. In response to this cue the ventral-most midline cells of the neural tube form the floor plate (Fig. 10.6). In the neural tube the induced floor plate takes over the production of HEDGEHOG. The signal stimulates the development of motoneurons on either side of the floor plate (see also Fig. 16.11).
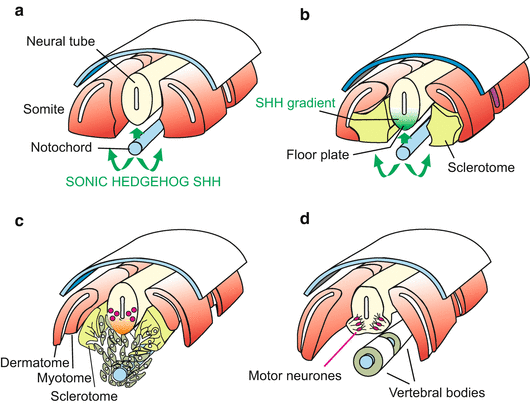
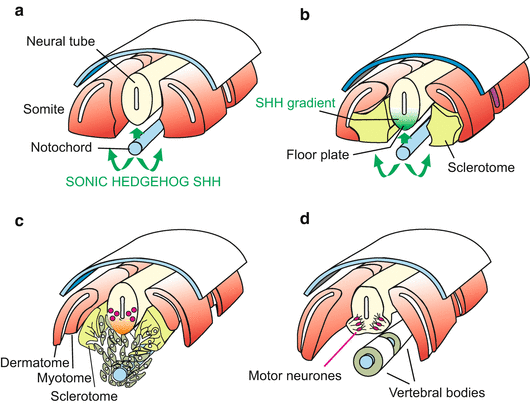
Fig. 10.6
(a)–(d) SONIC HEDGEHOG signalling in vertebrates. The protein encoded by the gene sonic hedgehog can appear in a membrane-associated form or in a form that detaches from the cell surface and spreads into the surrounding spaces where it reaches distant target cells. SONIC HEDGEHOG directly presented by the notochord to the adjacent neural tube induces (a) the formation of the floor plate in the neural tube. Subsequently the floor plate also produces HEDGEHOG, inducing (b) neuroblasts to differentiate into motoneurons (c), (d); see also Fig. 16.11c. SONIC HEDGEHOG released by the notochord into its surroundings induces (c) the sclerotome cells to detach from the somite, migrate around the notochord and form the vertebral bodies (d)
The soluble diffusing component of SONIC HEDGEHOG reaches the somites. In the somites, the HEDGEHOG signal induces the sclerotome cells to detach. They migrate towards the emitter of the signal, enclose the notochord and construct the vertebrae (Fig. 10.6).
SONIC HEDGEHOG gets another chance to demonstrate its versatility when the limbs are formed. In the limb bud of the chick embryo HEDGEHOG is expressed at the posterior border, where a signal emitter known as ZPA (Zone of Polarizing Activity) is located. When cells expressing HEDGEHOG are transplanted to the anterior border of the limb bud, supernumerary fingers are formed (see Fig. 10.13). This intriguing phenomenon is discussed further in Sect. 10.8.
Also in the establishment of the left-right asymmetry in our body SONIC HEDGEHOG is an essential factor (Sect. 10.6).
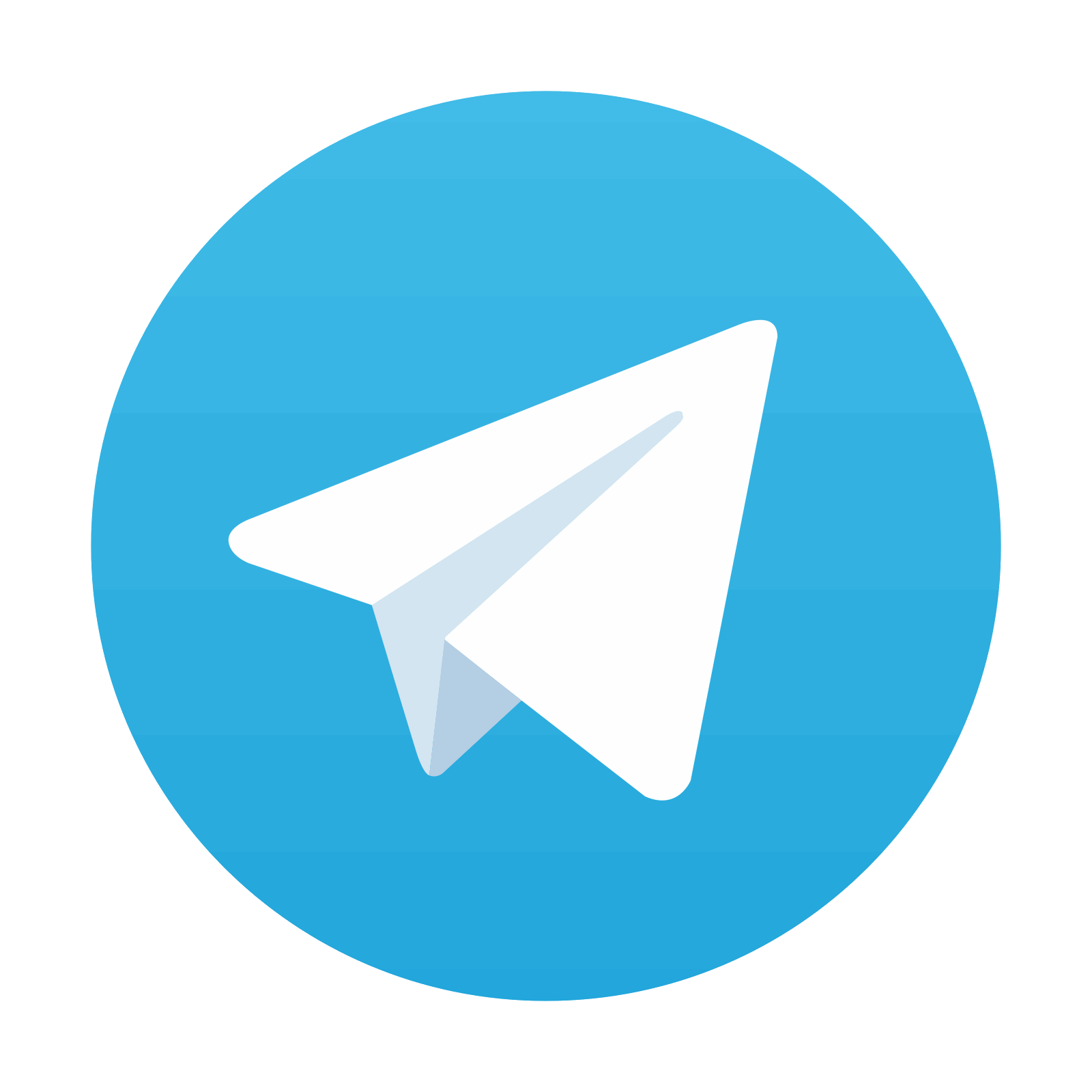
Stay updated, free articles. Join our Telegram channel

Full access? Get Clinical Tree
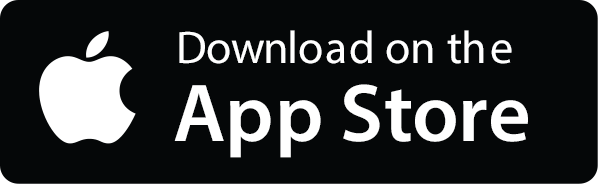
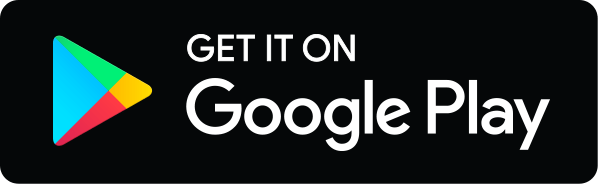