CHAPTER 9 Comparative placentation
Upon entering the uterine cavity, the embryo is initially nourished by secretions from the uterine glands. Collectively, these products are known as histotrophe or ‘uterine milk’. However, with development this arrangement rapidly becomes inadequate. To counteract the insufficiency, a close relationship has to be established between extra-embryonic tissues, which are vascularized from the embryo proper, and the maternal circulatory system. This allows the embryo to import blood-borne maternal nutrients, the haemotrophe, and to export its own waste products. Together, histotrophe and haemotrophe are referred to as embryotrophe. To accomplish exchange between the mother and her embryo a temporary organ, the placenta, is formed by contributions of both extra-embryonic and maternal tissues. As will be explained below, formation of the placenta (placentation) necessitates close synchrony between the state of uterine receptivity and the stage of embryonic development.
PERI-IMPLANTATION CONCEPTUS DEVELOPMENT
In most domestic species, the embryo reaches the uterine cavity prior to blastulation (see Chapter 6). At the end of blastulation, the embryo consists of a sphere of trophectoderm surrounding the inner cell mass and the blastocyst cavity (Fig. 9-1). When engaged in placental formation, the trophectoderm is referred to as trophoblast. As is true of most of the extra-embryonic tissues, the trophoblast is essential during intra-uterine life but it is expelled at parturition as part of the afterbirth.
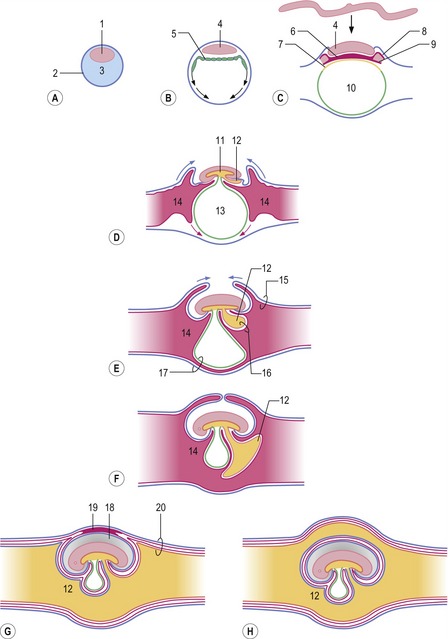
Fig. 9-1: Development of the extra-embryonic membranes presented as longitudinal sections through progressively older embryos (A to F). 1: Inner cell mass; 2: Trophectoderm; 3: Blastocyst cavity; 4: Epiblast; 5: Hypoblast; 6: Intra-embryonic mesoderm; 7: Endoderm; 8: Somatic extra-embryonic mesoderm; 9: Visceral extra-embryonic mesoderm; 10: Primitive yolk sac; 11: Primitive gut; 12: Allantois; 13: Definitive yolk sac; 14: Extra-embryonic coelom; 15: Chorion; 16: Splanchnopleura; 17: Fusion between yolk sac wall and chorion allowing for formation of choriovitelline placenta; 18: Amniotic cavity; 19: Mesamnion; 20: Chorioallantois allowing for formation of chorioallantoic placenta. G: In cattle and pigs, the chorion and the dorsal portion of the amniotic wall remain fused in the mesamnion. H: In horse and dog, the allantois surrounds the amnion completely.
At about the time of hatching from the zona pellucida, the inner cell mass differentiates into the epiblast and hypoblast (Fig. 9-1). The hypoblast gradually forms an inner lining of the epiblast and trophectoderm. When complete, the enclosed cavity may be referred to as the primitive yolk sac, analogous to the yolk sac found in avian embryos. The process of gastrulation results in establishment of the three germ layers: endoderm, mesoderm and ectoderm (see Chapter 7). During this process, the endoderm gradually displaces the hypoblast beneath the epiblast. Meanwhile the extra-embryonic mesoderm is split into somatic (or parietal) and visceral (or splanchnic) sheets lining the extra-embryonic coelom. The extra-embryonic somatic mesoderm associates with the overlying trophectoderm to give rise to the chorion, whereas the visceral mesoderm together with the hypoblast and endoderm forms the splanchnopleura. Eventually, the body foldings of the embryo proper result in the formation of the primitive gut and the definitive yolk sac from the primitive yolk sac (see Chapter 7). The definitive yolk sac wall fuses with the chorion in some species to establish a choriovitelline placenta.
The allantois develops as an evagination from the hindgut (Fig. 9-1). The evagination occurs after formation of the definitive yolk sac and, due to its origin from the hindgut, its wall is made up of endoderm on the inside with a covering of visceral mesoderm on the outside, the two layers together forming the splanchnopleura. The visceral mesoderm of the yolk sac is the area where blood and blood vessel formation is first seen, and this development is later followed by vascularization of the visceral mesoderm associated with the allantois (see Chapter 12). In contrast, the somatic mesoderm, including that of the chorion, remains avascular initially. As the embryo grows, the allantois gradually expands into the extra-embryonic coelom, eventually occupying most of this cavity. Where the allantoic wall and the chorion meet, they fuse, forming the chorioallantois which gradually becomes vascularized from vessels in the allantoic visceral mesoderm and gives rise to the chorioallantoic placenta.
CHANGES IN THE ENDOMETRIUM AND MATERNAL RECOGNITION OF PREGNANCY
During the period when the embryo is still moving freely in the uterine cavity, the uterus prepares for placentation. Oestrogens and progesterone are the principal hormones produced within the ovary (see Chapter 3). High levels of oestrogens are secreted into the bloodstream during prooestrus and oestrus (the follicular phase of the oestrous cycle); progesterone predominates during the following periods of metoestrus and dioestrus (the luteal phase) when the early embryo moves from the oviduct into the uterus. It is these cyclic phases of hormone production in the ovary that stimulate marked changes in the endometrium, the lining of the uterus.
In ruminants, the corpus luteum produces oxytocin as well as progesterone. Oxytocin stimulates the endometrium to synthesize prostaglandins (PGF2α; see Chapter 3) which have been identified as the main cause of luteolysis in ruminants (as well as in pigs and horses). In ruminants, interferon-tau (IFN-t) is produced by the trophectoderm, inhibiting formation of endometrial oxytocin receptors. Thus, in the presence of an embryo, oxytocin cannot stimulate synthesis of PGF2α and luteolysis is prevented. In addition, IFN-t stimulates production of histotrophe from the endometrial glands.
Pigs employ another strategy to interrupt the luteolytic pathway. Although oxytocin is also produced by the corpora lutea in the pig, and promotes synthesis of endometrial PGF2α, pig embryos prevent luteolysis differently. Oestradiol is produced from their trophectoderm around Days 11 to 12 of development causing PGF2α to be secreted into the uterine lumen instead of into the maternal blood stream. In the lumen, PGF2α is quickly degraded. In addition to changing the secretion of PGF2α from endocrine (into the maternal circulation) to exocrine (into the uterine lumen), oestradiol is also believed to stimulate myometrial contraction facilitating distribution of embryos within the very long uterine horns. It has been shown that at least four embryos must be present in order to prevent luteolysis in the pig.
CLASSIFICATION OF PLACENTA
There are enormous differences among species with respect to how placentation is initiated and, accordingly, how its final architecture develops. Many different classification schemes for the placenta, based on a variety of criteria, have been proposed over the years. One scheme is based on nature of the extra-embryonic tissue that contributes to the placenta, leading to placentae being classified as either choriovitelline or chorioallantoic. In the choriovitelline placenta, the yolk sac wall combines locally with the chorion to form an area for exchange (Fig. 9-1). In domestic animals a functional choriovitelline placenta is seen only in carnivores and horses. The chorioallantoic placenta, which is the primary functional placenta in all domestic species, is established by fusion between the allantoic wall and chorion establishing the chorioallantois. In pigs and ruminants, the yolk sac involutes 3 to 4 weeks after conception and never forms a functional placenta.
Another classification scheme is based on the structure of the chorioallantoic surface and its interaction with the endometrium. Areas where the chorioallantois interacts with the endometrium and engages in placental formation are referred to as chorion frondosum. In contrast, areas where the chorioallantois is free, not engaged in placental formation, and therefore has a smooth surface, are known as chorion laeve. In pigs and horses, chorion frondosum is diffusely distributed over the entire chorioallantoic surface and so the placenta is categorized as being diffuse. The porcine chorioallantoic surface area is increased by foldings, revealed as primary plicae and secondary rugae, and is thus referred to as being folded. In the horse, because chorionic villi are gathered in numerous specialized ‘microzones’, known as microcotyledons, and extending into crypts of the endometrium, the equine placenta is also referred to as being villous.
A third classification scheme is based on the number of tissue layers separating the fetal and maternal circulations, thereby forming the placental barrier (Fig. 9-2). There are always three fetal extra-embryonic layers in the chorioallantoic placenta: the endothelium lining the allantoic blood vessels; chorioallantoic mesenchyme, originating from the fused somatic (chorionic) and visceral (allantoic) mesoderm; and the chorionic epithelium, i.e. the trophoblast. However, the numbers of layers retained in the maternal portion of the placenta varies with species. Before placentation, the endometrium presents three layers that could contribute to the placental barrier: the endometrial epithelium, connective tissue, and vascular endothelium.
< div class='tao-gold-member'>
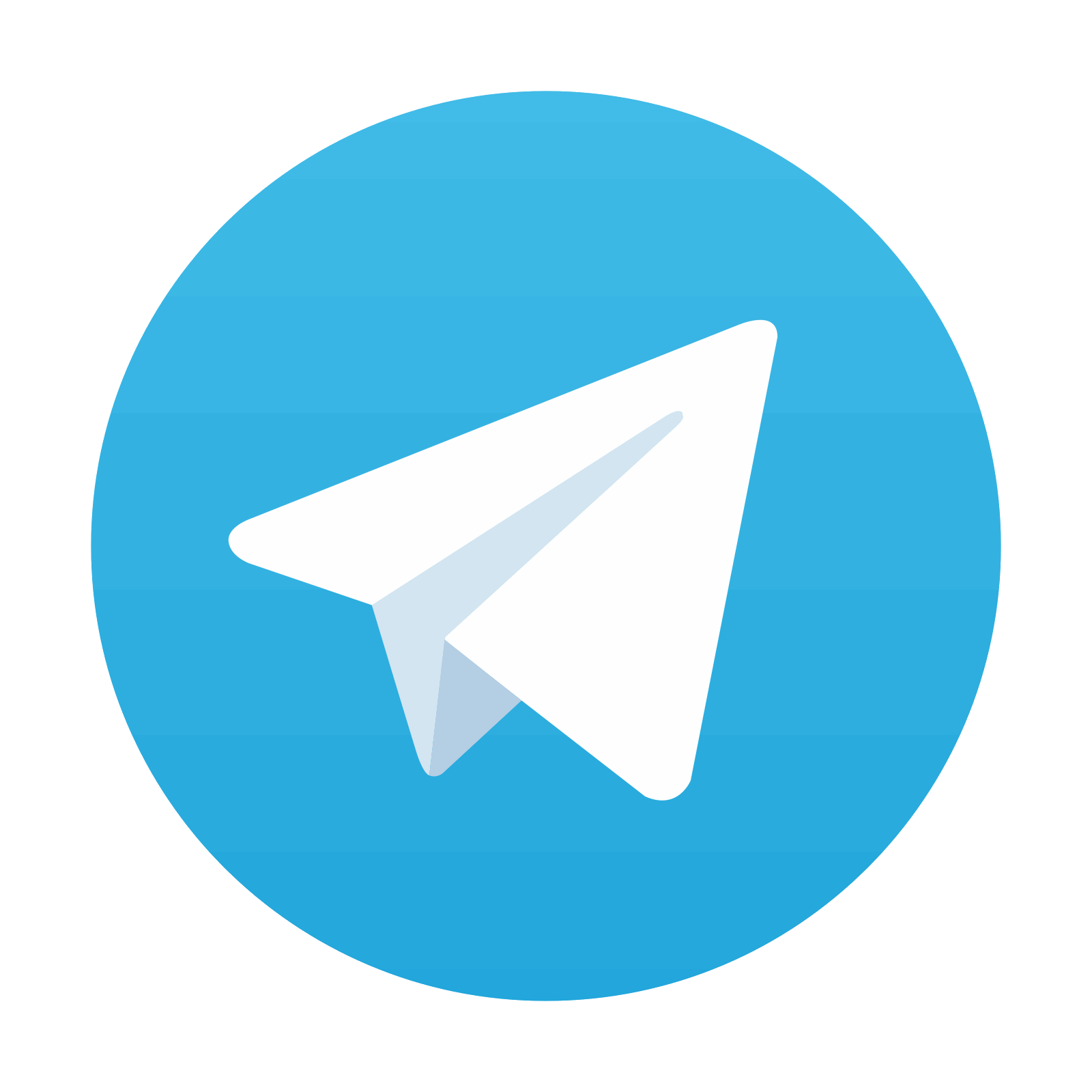
Stay updated, free articles. Join our Telegram channel

Full access? Get Clinical Tree
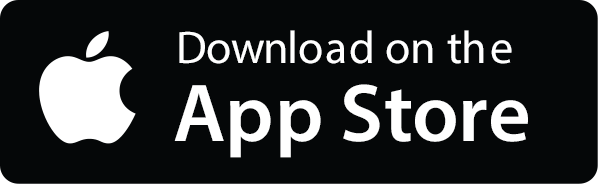
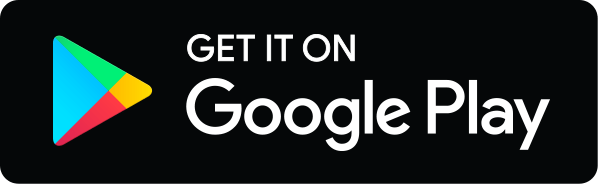