CHAPTER 18 Curtis W. Dewey & Lauren R. Talarico Skeletal muscle is the effector organ for the somatic motor nervous system. In general, clinical signs of skeletal muscle dysfunction include weakness with preservation of sensory function—e.g. nociception (deep pain perception), proprioception—muscle atrophy, and muscle pain (myalgia). In some diseases, muscle hypertrophy is present rather than atrophy. Also, myalgia is not a feature of some myopathies. Skeletal muscle is composed of multinucleated cells termed “myofibers” that are arranged in bundles called “fascicles.” Each myofiber is innervated by an axonal process of a motor neuron at a specialized area of the sarcolemma (muscle cell plasma membrane) called the endplate (see Chapter 19). The myofibers contain the contractile apparatus, which comprises interlocking myofilaments (actin, myosin, troponin, tropomyosin). Muscle contraction occurs when calcium is released from the sarcoplasmic reticulum (the myofiber endoplasmic reticulum) following sodium influx into the myofiber (depolarization). Adenosine triphosphate (ATP) is required for the coordinated contraction and relaxation of the muscle fiber. A muscle enzyme called creatine kinase (CK) is required to immediately replenish ATP from adenosine diphosphate (ADP) by cleaving a high-energy phosphate group from the compound phosphocreatine, also found in the myofiber. There are two main types of myofibers, differentiated upon the intensity of histochemical staining at different pHs (Fig. 18.1) with the ATPase reaction (muscle biopsy samples). Type I fibers are “slow-twitch” (relatively high levels of oxidative enzymes and lipid, low glycogen content) fibers and type II fibers are “fast-twitch” (relatively high levels of glycogen, lesser amounts of oxidative enzymes and lipid) fibers. A uniquely staining fiber type is also found only in masticatory muscle, called type IIM. One motor neuron will innervate a number of myofibers within a fascicle, and the fiber types of those myofibers will all be the same. The motor neuron and the myofibers it innervates comprise what is called a motor unit. The motor neuron dictates the fiber type of the myofibers of its motor unit. The myofibers of a single motor unit are normally scattered through a fascicle, giving a “checkerboard” appearance to the stained biopsy sample. Figure 18.1 Cryosections from the vastus lateralis muscle reacted with myofibrillar ATPase at pH 9.8 shows type I fibers stained lightly and type II fibers stained dark. Type II fiber atrophy is present, consistent with an endocrine disorder such as hypothyroidism, or Cushing’s syndrome. (G. Diane Shelton, University of California, La Jolla, CA, 2014. Reproduced with permission from G. Diane Shelton.) In some cases, it will be difficult to clinically discern a myopathy from a neuropathy or neuromuscular junction disorder. A diagnosis of myopathy is based upon neurologic examination findings as well as specific diagnostic tests. Typical diagnostic tests to pursue when a myopathy is suspected include serum CK measurement (elevations suggest muscle damage), and electrodiagnostics (remember that electromyographic [EMG] abnormalities can be seen with neuropathies and myopathies). However, these tests may yield abnormal results for reasons unrelated to neuromuscular disease. In particular, CK activity may be elevated in anorexic cats. Historical information, examination findings, and a decrease in CK activity with nutritional support may be used to help differentiate this elevation from that seen in neuromuscular disease. Muscle and/or nerve biopsies can be very helpful in elucidating the cause of a peripheral nervous system disorder. In addition to the ATPase reaction, there is a barrage of histochemical stains and reactions that can be applied to cryosections of properly frozen muscle biopsies to help characterize the nature of the muscle disorder (Fig. 18.2 to Fig. 18.9). A summary of myopathies of dogs and cats is shown in Table 18.1. Table 18.1 Myopathies of dogs and cats. Fig. 18.2 is of hematoxylin and eosin (H&E) stained muscle cryosections showing a large pale staining necrotic fiber. H&E staining is used for the evaluation of general morphology. Fig. 18.3 is of modified Gömöri trichrome stained cryosections showing a prominent intramuscular nerve branch. Myelin stains pink with this stain. The modified Gömöri trichrome stain is a good general morphological stain, highlights intramuscular nerve branches and stains nemaline rods, and identifies ragged red-like fibers. Figure 18.2 Hematoxylin and eosin stain muscle cryosections. (G Diane Shelton, University of California, La Jolla, CA, 2014. Reproduced with permission from G Diane Shelton.) Figure 18.3 Modified Gömöri trichrome stained cryosections. (G Diane Shelton, University of California, La Jolla, CA, 2014. Reproduced with permission from G Diane Shelton.) Fig. 18.4 shows staining with periodic acid-Schiff highlights glycogen or polysaccharide deposits that would suggest a glycogen or polysaccharide storage disorder. Note the figure has a glycogen deposit within a muscle fiber that is stained dark purple. Fig. 18.5 is of a nicotinamide adenine dinucleotide reductase reaction that identifies mitochondrial accumulations and tubular aggregates. In this muscle section, dark-blue reactions are present under the sarcolemma and extend into the sarcoplasm, consistent with lobulated fibers. Figure 18.4 Staining with periodic acid-Schiff. (G Diane Shelton, University of California, La Jolla, CA, 2014. Reproduced with permission from G Diane Shelton.) Figure 18.5 Nicotinamide adenine dinucleotide reductase reaction. (G Diane Shelton, University of California, La Jolla, CA, 2014. Reproduced with permission from G Diane Shelton.) Fig. 18.6 demonstrates the oil red O stain that localizes the presence of lipid droplets composed of neutral triglycerides within muscle fibers supporting a metabolic myopathy which may be mitochondrial in origin, or resulting from a defect in fatty acid oxidation or carnitine metabolism. Fig. 18.7 shows how cryosections react with esterase to highlight large numbers of macrophages in a necrotizing myopathy. Esterase stains lysosomal accumulations in macrophages and also in degenerating muscle fibers. Fig. 18.8 shows the cryosection reaction highlighting the motor endplates. The acid phosphatase reaction also highlights increased lysosomal activity in macrophages and within muscle fibers (Fig. 18.9; note in this cryosection the red stained areas within macrophages). Figure 18.6 Oil red O stain. (G Diane Shelton, University of California, La Jolla, CA, 2014. Reproduced with permission from G Diane Shelton.) Figure 18.7 Cryosections reacting with esterase. (G Diane Shelton, University of California, La Jolla, CA, 2014. Reproduced with permission from G Diane Shelton.) Figure 18.8 Cryosections reacting with esterase highlight the dark reddish brown stained motor endplates. Figure 18.9 Acid phosphatase reaction. (G Diane Shelton, University of California, La Jolla, CA, 2014. Reproduced with permission from G Diane Shelton.) The immunoreagent Staphylococcal protein A-horseradish peroxidase identifies circulating serum antibodies that bind to the sarcolemma, to myofibers, to muscle nuclei in cases with anti-nuclear antibodies and to motor endplates in cases of myasthenia gravis following incubation of muscle cryosections with patient serum (Fig. 18.10). In the figure, distinct sarcolemmal labeling can be seen that is consistent with antibodies against an unidentified sarcolemmal protein(s) in a dog with polymyositis. Figure 18.10 Immunoreagent Staphylococcal protein A-horseradish peroxidase. (G Diane Shelton, University of California, La Jolla, CA, 2014. Reproduced with permission from G Diane Shelton.) The term “muscular dystrophy” refers to a wide variety of inherited myopathies with specific defects of skeletal muscle proteins. The most common form of MD in both humans and animals is associated with the sarcolemmal protein dystrophin. Dystrophin is located on the X-chromosome and thus is an X-linked disorder (X-linked muscular dystrophy, or XLMD). Dystrophin is thought to have an important structural role for myofibers and may also serve a vital role in cellular homeostasis, possibly as a regulator of intracellular calcium transport. X-linked dystrophin-deficient muscular dystrophies in dogs and cats are believed to be the veterinary analogs of Duchenne and Becker muscular dystrophy of humans, and have been described in various dog breeds (Golden Retriever, Rottweiler, German Shorthaired Pointer, Irish Terrier, Samoyed, Belgian Shepherd (Groenendael), Miniature Schnauzer, Rat Terrier, Wire-haired Fox Terrier, Samoyed, Brittany Spaniel, Japanese Spitz, Weimaraner, Labrador Retriever, Old English Sheepdog, Grand Basset Griffon Vendéen, Corgi) and cats (domestic shorthair, Siamese, Maine Coon). Specific gene mutations have been defined in Golden Retrievers, German Shorthaired Pointers, Cavalier King Charles Spaniels, Japanese Spitz dogs and Rottweilers. Progressive muscle atrophy predominates in dogs (although some muscle groups tend to hypertrophy), whereas muscle hypertrophy is the hallmark of XLMD in cats. The pathologic change in affected muscle is characterized histologically by variation in myofiber size (including both degenerating and regenerating myofibers), with necrosis and mineralization of myofibers (Fig. 18.11). A number of dystrophin-associated proteins (e.g. sarcoglycans, dystroglycans), as well as laminins (basement membrane proteins) may also be deficient in other forms of MD. Figure 18.11 H&E (A) and alizarin red (B) stained muscle biopsy specimens from a dog with Duchenne muscular dystrophy. In A, a wide variation in muscle fiber size can be appreciated, and in B, there is evidence of intracellular calcium accumulation. (G. Diane Shelton, University of California, La Jolla, CA, 2014. Reproduced with permission from G. Diane Shelton.) Dystrophin-deficient muscular dystrophy is best described in the Golden Retriever. Marked elevations in serum CK activity detected during the first few weeks of life are a hallmark of this disease. Clinical signs of partial trismus and a “bunny-hopping” gait may be appreciated as early as 6 wks of age in male puppies. Although MD is largely restricted to males, it has been reported in female dogs. Additionally, female carriers of dystrophin mutations often show elevations in their serum CK activities, without significant clinical disease. In affected dogs signs typically progress over 3–6 mos, after which time the disease often stabilizes. Common clinical signs include progressive muscle atrophy of the limbs, head, and trunk, exercise intolerance, a stilted gait, plantigrade stance (with associated tarsal joint contracture), excessive salivation (pharyngeal dysfunction), weak bark (dysphonia), kyphosis that progresses to lordosis, and hypertrophy of the muscles of the base of the tongue. Proximal limb muscles, particularly the cranial sartorius muscle, may undergo hypertrophy in some dogs. Dysphagia and exercise-induced myalgia may present as sole clinical findings initially, prior to the onset of generalized weakness. Hiatal hernia and gastroesophageal reflux can be seen clinically, secondary to diaphragmatic and esophageal dystrophy, respectively. Spinal reflexes are normal initially but may become decreased due to muscle fibrosis. Inhalation pneumonia from pharyngeal/esophageal dysfunction and heart failure due to cardiomyopathy have also been reported. Uncommonly, some puppies display a more fulminant form of MD, and die within 10 days of birth. There appears to be considerable variation in time of disease onset and severity of clinical signs in cats with XLMD. Although affected cats may exhibit characteristic signs, such as a “bunny-hopping” pelvic limb gait in the first months of life, they may also have mild or inapparent clinical signs of myopathy until approximately 2 yrs of age. Progressive “stiffness” in gait and muscle hypertrophy are prominent features of MD in cats, in contrast to the weakness and atrophy characteristic of the canine disorder. Stress may induce open-mouthed breathing and/or syncopal episodes in cats with MD, presumably due to a combination of cardiac and respiratory muscle involvement. A form of MD associated with laminin alpha 2 deficiency (normal dystrophin) has been described in two young female cats (Siamese and domestic shorthair). These cats exhibited progressive muscle atrophy and weakness, beginning in the pelvic limbs, at approximately 5–6 mos of age. Spinal reflexes were depressed to absent. Both cats progressed to nonambulatory status over 6–12 mos. A diagnosis of MD is based upon signalment, characteristic clinical findings, marked serum CK elevations (often over 10,000 Units/L), bizarre high-frequency discharges on electromyograph, and muscle biopsy results (myofiber degeneration/regeneration with or without mineralization). Overall, the degree of elevation of CK in affected dogs does not correlate to the severity of their clinical signs. Serum CK levels in female dogs with MD have been less dramatically elevated compared with most males with the disorder. Cats with dystrophin-deficient muscular dystrophy frequently show myotonic discharges and fibrillation potentials on EMG evaluation, particularly in the proximal appendicular muscles. Motor nerve conduction studies remain normal. A lack or absence of dystrophin can be demonstrated immunocytochemically on a muscle biopsy specimen or by western blot analysis. Occasionally, dystrophin-associated proteins and laminins may also be deficient, with or without obvious lack of muscle dystrophin. Muscles of affected Golden Retrievers undergo fibrosis earlier than in other affected breeds, a process that appears to be cytokine-driven. Golden Retrievers with MD may show consistent abnormalities on thoracic and pelvic radiographs. Diaphragmatic asymmetry, with an undulating pattern and either left crural flattening or ventral displacement, may be seen on thoracic radiographs, and can be accompanied by a hiatal hernia. Characteristic pelvic radiographic abnormalities include narrowing of the body of the ilia, ventral deviation of the tuber ischii, elongation of the obturator foramina, and lateral elongation of the wings of the ilia. The pelvis is overall tilted vertically, narrowed, and elongated. These pelvic changes may be secondary to myopathy-induced contractures resulting in bone remodeling and appear to be specific to the form of MD seen in Golden Retrievers. Cats with MD may develop marked diaphragmatic hypertrophy, with megaesophagus seen secondary to esophageal stricture. Figure 18.12 Characteristic posture of a dog with Labrador Retriever myopathy. The age at which the dogs were evaluated was between 4 and 7 mos, but all dogs had exhibited an abnormal gait and posture within the first several weeks of life. Characteristic clinical signs of dysfunction include a palmigrade and plantigrade stance (Fig. 18.13), splayed digits in the forelimbs (Fig. 18.14), generalized weakness, and exercise intolerance. Figure 18.13 Characteristic posture of a Rottweiler with distal myopathy. (Courtesy of Dr. Stephen Hanson.) Figure 18.14 Splaying of the digits, characteristic of Rottweiler distal myopathy. (Hanson et al., 1998. Reproduced with permission from Wiley.)136 Clinical signs are usually appreciated when affected puppies and kittens begin to ambulate. Affected animals typically appear worse after a period of rest. Cold temperatures also tend to cause exacerbation of clinical signs. The gait is stiff and tends to improve or even normalize with activity. The pelvic limbs are often more severely affected than the thoracic limbs; in canine myotonia, they may be advanced simultaneously in a “bunny-hopping” fashion. It may be difficult for affected dogs to flex the stifle joints. The thoracic limbs are often held abducted while ambulating, due to a decreased ability to flex the proximal limb joints. Myotonic patients may have difficulty rising from a sternal position. Myotonic kittens tend to snag their claws when walking on carpet. When myotonic kittens are startled, they may hyperextend all four limbs and fall into lateral recumbency for approximately 10 sec. Startling in these kittens may also result in bilateral prolapse of the nictitans, blepharospasm (due to spasm of the orbicularis oculi muscles), flattening of the ears, and retraction of the lips. Generalized muscle hypertrophy (especially proximal appendicular and neck muscles and the tongue in dogs, gastrocnemius muscles most prominent in cats) is often appreciated and percussing the muscle may leave an indentation, referred to as a “myotonic dimple” (Fig. 18.15). Some patients will exhibit dysphagia and respiratory problems (e.g. stridor) because of sustained contraction of pharyngeal and laryngeal musculature, respectively. Affected kittens may exhibit signs of dysphonia, characterized by a hoarse meow and quiet purr. Unusual physical characteristics apparent in all of a group of related myotonic Schnauzers were prognathism (shortened mandible) and medially displaced canine teeth. Figure 18.15 Myotonic dimpling in the caudal thigh musculature of a myotonia congenita patient. (Dr. G Kortz, 2014. Reproduced with permission from Dr. G Kortz.) Figure 18.16 EMG tracing of a dog with myotonia. Age of onset of dysfunction ranges from 8 mos to 9 yrs (mean, 5 yrs). Clinical signs are usually limited to apparently nonpainful pelvic limb lameness, which is more obvious at a trot than at a walk. In most cases, the lameness has an insidious onset and progresses over weeks to months before reaching a plateau. Occasionally, acute onset of lameness is reported. Bilateral involvement occurs in approximately 26% of cases. When both pelvic limbs are affected, the degree of dysfunction may not be symmetric; also, one limb may be affected initially, the other becoming dysfunctional at a later date. Although classically considered a nonpainful disorder, one study found that a painful response could be elicited from the majority of affected dogs with hip abduction and/or digital pressure applied to the distal aspect of the fibrotic muscle. The fibrous muscle prevents full extension of the pelvic limb during ambulation. The lameness in the affected limb is characterized by internal rotation of the stifle and external rotation of the hock as the limb is advanced (Fig. 18.17). The foot performs a “flipping” motion at the end of each stride. The resultant gait is often described as “jerky” or “goose stepping.” Affected muscle tissue may be visibly abnormal and the distal myotendinous area is often firm and hypertrophied when palpated (Fig. 18.18). Figure 18.17 Typical pelvic limb gait of a dog with fibrotic myopathy. Figure 18.18 Bilateral fibrosis of the gracilis muscles in a dog with fibrotic myopathy. Nemaline myopathy is a rare, presumably inherited disorder described in young related cats. Congenital nemaline myopathy has also been reported in two dogs, a 10-mo-old Border Collie, and an 11-yr-old Schipperke. Nemaline rods were also observed in muscle biopsy specimens from a dog with hyperadrenocorticoid myopathy and a dog with hypothyroid myopathy. Finally, nemaline rods have been reported as incidental findings in muscle biopsies of dogs with neuromuscular disease. The presence of nemaline rods in a muscle biopsy is not necessarily specific for nemaline myopathy. A diagnosis of nemaline myopathy should be suspected when there are numerous nemaline rods present in the absence of any other cause for a myopathy. The pathogenesis of nemaline myopathy is unknown, but special stains of muscle biopsy specimens reveal rod-shaped inclusions within myofibers (nemaline rods). In human nemaline myopathy, these rods have been shown to be composed of cytoskeletal proteins identical to those found in the Z-band area of the contractile filament apparatus. A myofiber cytoskeletal protein abnormality is suspected. Accumulations of tubulin-positive crystalline inclusions, and dystrophin and spectrin proteins in addition to nemaline rods, have been reported in cats as well. A myofibrillar myopathy showing accumulations of alpha-actin (Z-disc material) and desmin within the myofiber has been reported in an Australian Shepherd dog. Dancing Doberman disease This is an idiopathic syndrome in adult Doberman Pinschers that has characteristics of both a neuropathy and a myopathy. It is discussed in more detail in Chapter 17. Figure 18.19 Fluoroscopic image of a dog with cricopharyngeal achalasia before (A) and after (B) cricopharyngeal myotomy. Prior to surgery, very little of the contrast bolus passed through the upper esophageal sphincter. (Ladlow and Hardie, 2000.)188 Treatment of cricopharyngeal achalasia is myotomy or myectomy of the cricopharyngeus muscle. This surgical therapy is highly effective for this disorder. However, if the diagnosis is incorrect, cricopharyngeal myotomy/myectomy may not only be of no therapeutic value but also lead to life-threatening aspiration pneumonia. Lastly, inappropriate or insufficient treatment of aspiration pneumonia and/or malnutrition preoperatively in animals with cricopharyngeal achalasia frequently worsens postoperative outcome. The authors have treated one case of cricopharyngeal achalasia with therapeutic Botox injections. The abnormal cricopharyngeal musculature was identified with an EMG. The Botox was injected into the abnormal muscle and repeated 3 wks later. This procedure was both therapeutic and used to prognosticate this patient’s overall success with a permanent myectomy. This patient showed clinical improvement after this procedure and went on to have a permanent myectomy performed. Episodic muscle hypertonicity (“cramp”)6, 106, 145, 166, 220, 221, 222, 223, 249, 282, 294, 318, 343, 361, 362, 363 This uncommon disorder, initially reported in the Scottish Terrier breed (“Scotty cramp”), is characterized by episodic muscle hypertonicity. The episodes are of variable frequency and severity and are induced by stress, exercise, and excitement. The disease appears to be inherited as an autosomal recessive trait in Scottish Terriers. Although the pathogenesis is not completely understood, clinical manifestations of this disorder may be due to a functional deficiency of serotonin in the CNS. Drugs that potentiate CNS serotonergic effects (e.g. acepromazine) alleviate clinical signs, whereas those that decrease CNS serotonergic effects (e.g. amphetamine) either worsen or induce clinical signs. Similar conditions have been described in a number of different breeds. These and other movement disorders are covered in more detail in Chapter 10. Myokymia and neuromyotonia109, 131, 132, 266, 336, 347, 366 This category of disease (especially myokymia) is often discussed with tremor and movement disorders, but it is probably more accurately categorized as a neuropathy (see Chapter 17). It is discussed in this chapter because the manifestations of the disorder appear clinically more like a myopathy than a neuropathy. It is also briefly discussed in Chapter 10. The underlying pathophysiological cause for the intermittent and excessive muscle contraction characteristic of myokymia/neuromyotonia is thought to be hyperexcitability of motor axons; in humans, this hyperexcitability is thought to be due to abnormalities of voltage-gated potassium channels (VGKC) in these nerves, which is often due to an autoimmune process. In addition to autoimmune disorders of VGKC, there are some heritable disorders of these ion channels; for example, the disorder known as episodic ataxia with myokymia is due to a point mutation in the VGKC gene (KNA1). Whether acquired (autoimmune) or inherited, the VGKC involved in this disorder are fast potassium channels, also referred to as delayed rectifier channels, whose function is necessary for the cessation of depolarization as well as repolarization of the axon. If these channels are dysfunctional and/or decreased in density, prolongation of depolarization will allow more calcium to enter (calcium channels will remain open), with a subsequent excessive release of acetylcholine transmitter quanta into the synaptic cleft. This, along with delayed repolarization, will lead to excessive and repetitive muscle contraction. Confusion regarding this clinical phenomenon is likely perpetuated both by an unnecessary number of descriptive terms as well as the vast array of primary disorders that can lead to the manifestation of this type of muscular activity. The terms “myokymia” and “neuromyotonia” probably refer to the same class of disorders, differing solely in the frequency (in Hz) of the episodic involuntary muscle fiber contraction that characterizes the disease syndrome. The term myokymia, which is derived from the Greek word kyma (which means “wave”), is probably the most descriptive term for the vermicular (“worm-like”), rippling, or undulating motion of the skin (most notably on the proximal limbs) caused by the spontaneous intermittent contraction of subcutaneous musculature. Myokymic discharges are characteristically bursts of single motor unit action potentials that have a frequency of 5–150 Hz. Neuromyotonic discharges are described as similar episodic discharges of higher frequency (150–300 Hz, often with a waning amplitude), which are believed to be more likely than myokymic discharges to culminate in generalized contractions of large muscle groups (e.g. limb musculature). These intermittent bursts of motor unit action potentials occur as doublets, triplets, or multiplets on EMG examination, and sound (over the loudspeaker) like soldiers marching. These muscle fiber discharges persist during sleep and when the patients are under general anesthesia. The terms myokymia and neuromyotonia likely refer to different stages of severity of the same clinical condition. According to some sources, myokymia is considered a clinical manifestation of the overall disease syndrome of neuromyotonia. In other words, the umbrella term for the disorder is neuromyotonia, which includes the phenomenon of myokymia. Since the majority of reported cases in the veterinary literature describe patients that displayed myokymia and then rapidly progressed to generalized muscle contraction and collapse, this is probably the correct use of the terminology. In addition to these terms, neuromyokymia, continuous muscle fiber activity (CMFA), continuous motor unit activity (CMUA), neurotonia, pseudomyotonia, and episodic nonpostural repetitive myoclonus have been proposed. The list of disorders in humans that have been associated with concurrent myokymia and neuromyotonia is extensive and includes several autoimmune or suspected autoimmune disorders; it includes caudal fossa tumors, Guillain–Barré syndrome, multiple sclerosis, radiation-induced plexopathy, timber rattlesnake envenomation, chronic inflammatory demyelinating polyneuropathy (CIDP), thymoma, lymphoma, plasmacytoma, small-cell lung carcinoma, Hashimoto’s thyroiditis, Addison’s disease, rheumatoid arthritis, and acquired myasthenia gravis. The disorder has also been associated with penicillamine treatment. In addition to the multitude of terms already mentioned, the terms Isaacs’ syndrome, Mertens’ syndrome, Isaac–Mertens’ syndrome, and Morvan’s syndrome (also includes signs of encephalopathy) all refer to myokymia/neuromyotonia due to autoimmune response against VGKC. Myokymia and neuromyotonia have been reported in eight dogs and one cat. The dogs included three Jack Russell Terriers, two Yorkshire Terriers, a Border Collie, a Cocker Spaniel, and a mixed-breed dog. The one feline report was a 6-yr-old domestic shorthaired cat. With the exception of one dog with facial myokymia (6-mo-old Cocker Spaniel, age at onset of 4 mos) who had occasional involvement of the left shoulder musculature, all of the other reported cases exhibited neuromyotonia with more generalized muscle stiffness, in addition to myokymia. Of the neuromyotonia cases, the cat was the least severely affected, remaining ambulatory despite the involuntary muscle contractions. With the exception of one Border Collie with an age at onset of 2 yrs, the other dogs with neuromyotonia had a very young age at onset of disease (2–11 mos). These dogs all had a similar clinical presentation, in which the excessive muscular contraction culminated in collapse. In all cases, the dogs were fully conscious during the collapsing episodes. The episodes in these dogs were typically triggered by stress, excitement, or exercise and lasted for several minutes to several hours. Between episodes, the dogs returned to normal, or their pre-episode condition (see comments below on the Jack Russell Terriers). Episodes were heralded in three dogs by intense facial rubbing, similar to what has been reported in hypocalcemic dogs. Common to all of these reported cases of myokymia/neuromyotonia was moderate to severe hyperthermia during the episodes. In two cases, death during an episode was attributed to hyperthermia. Another common feature was persistence of spontaneous muscle contraction during sleep and general anesthesia. All three reported Jack Russell Terriers exhibited generalized ataxia in addition to the myokymia/neuromyotonia episodes. Whether this finding represents concurrent hereditary ataxia or a condition similar to human episodic ataxia with myokymia is unknown. Two of the Jack Russell Terriers also exhibited mild cyanosis during episodes. As with humans with myokymia/neuromyotonia, the diagnosis rests primarily on characteristic clinical features of the disease along with demonstrating the characteristic EMG abnormalities (i.e. episodic bursts of spontaneous muscle activity of specific frequencies). Other characteristic supportive features include serum elevations of ALT, AST, and CK concentrations. Muscle/nerve biopsy results in humans may be normal or indicative of axonal degeneration and/or demyelination. Muscle histopathology was normal in the four dogs for which it was performed. The Jack Russell Terriers had evidence of axonal degeneration and demyelination of peripheral nerves, with very mild muscle changes. One Yorkshire Terrier had normal muscle biopsy results. The cat had evidence of myofiber necrosis and regeneration, and intramuscular nerve branches were normal. Although none of the veterinary cases had an obvious underlying disorder for which the myokymia/neuromyotonia was considered a secondary or associated phenomenon, this should be addressed in such cases, based on what is known in the human literature on the subject. Unlike the scenario in human medicine, there is no assay for circulating anti-VGKC antibodies for dogs or cats (to diagnose autoimmune causes for myokymia/neuromyotonia), and no canine or feline hereditary mutations for the VGKC gene have been identified. A variety of drugs have been effective in treating human myokymia/neuromyotonia, including procainamide, phenytoin, carbamazepine, acetazolamide, mexiletine, and gabapentin. These drugs have membrane-stabilizing properties and may also be of some benefit for veterinary cases of myokymia/neuromyotonia. In people with underlying autoimmune disorders, immunosuppressive drugs are used in addition to membrane-stabilizing agents. The cat responded favorably to oral phenytoin, and one Yorkshire Terrier responded well to procainamide. Two other dogs had transient responses to such therapy. The Border Collie responded for several months to oral mexiletine, and then reverted to the previous frequency of episodes. One Jack Russell Terrier responded to oral procainamide, but died during an episode in the second month of treatment. Four dogs either died during an episode (two dogs) or were euthanized due to disease severity (two dogs), suggesting at least a guarded prognosis for this disorder. However, more experience with this disease, especially with treatment options recommended for people with the disorder (only one of the deceased dogs was treated with one of these drug options), will be necessary before an accurate estimate for prognosis can be formulated for myokymia/neuromyotonia in dogs and cats. Figure 18.20 Cat with cervical weakness demonstrating cervical ventroflexion. Treatment of this condition is oral potassium gluconate at an initial dose of 5–8 mEq/kg/day, divided into two doses. Normal potassium levels are often achieved within 1–3 days with this therapy. Maintenance therapy of 2–4 mEq/day is usually sufficient after achieving normal serum potassium levels. Potassium administration via intravenous fluids is usually counterproductive, because the dilutional and diuretic aspects of fluid administration actually further lower the potassium level. In life-threatening hypokalemia, concentrated intravenous potassium solutions can be administered at a rate of 0.4 mEq/kg/hr. However, this is potentially dangerous and can lead to fatal cardiac arrhythmias without close monitoring of the serum potassium level and the electrocardiogram. An alternative is a dopamine infusion of 0.5 mg/kg/min. This may cause a transient increase in serum potassium, and allow time for oral potassium supplementation. The prognosis for this condition with proper therapy is generally favorable. Most cats exhibit obvious improvement within 1–3 days of potassium supplementation, although complete recovery may take several weeks.
Myopathies: Disorders of Skeletal Muscle
Introduction8, 36, 40, 44, 61, 102, 141, 179, 214, 232, 301, 315
Degenerative/Developmental
Metabolic
Inflammatory/Infectious
Ischemic
Traumatic
Muscular dystrophy
Centronuclear myopathy
Exercise intolerance and collapse of Labrador Retrievers
Distal myopathy of Rottweilers
Myotonia congenita
Fibrotic myopathy
Nemaline myopathy
Myositis ossificans (fibrodysplasia ossificans progressiva)
Pharyngeal/esophageal dysfunction of Bouviers
Polysystemic disorder of English Springer Spaniels
Cricopharyngeal achalasia
Episodic muscle hypertonicity (“cramp”)
Myokymia and neuromyotonia
Hypokalemic myopathy
Hyperkalemic periodic paralysis
Hyperadrenocorticoid (Cushing’s) myopathy
Hypothyroid myopathy
Malignant hyperthermia
Exertional myopathy
Lipid storage/mitochondrial myopathies
Glycogen storage disorders (glycogenoses)
Masticatory myositis
Extraocular myositis
Laryngeal/pharyngeal myositis
Autoimmune polymyositis
Dermatomyositis
Feline hyperesthesia syndrome
Infectious myositis
Tetanus
Ischemic neuromyopathy
Infraspinatus contracture
Iliopsoas muscle injury
Quadriceps contracture
Coccygeal muscle injury
Disorders of skeletal muscle in dogs and cats
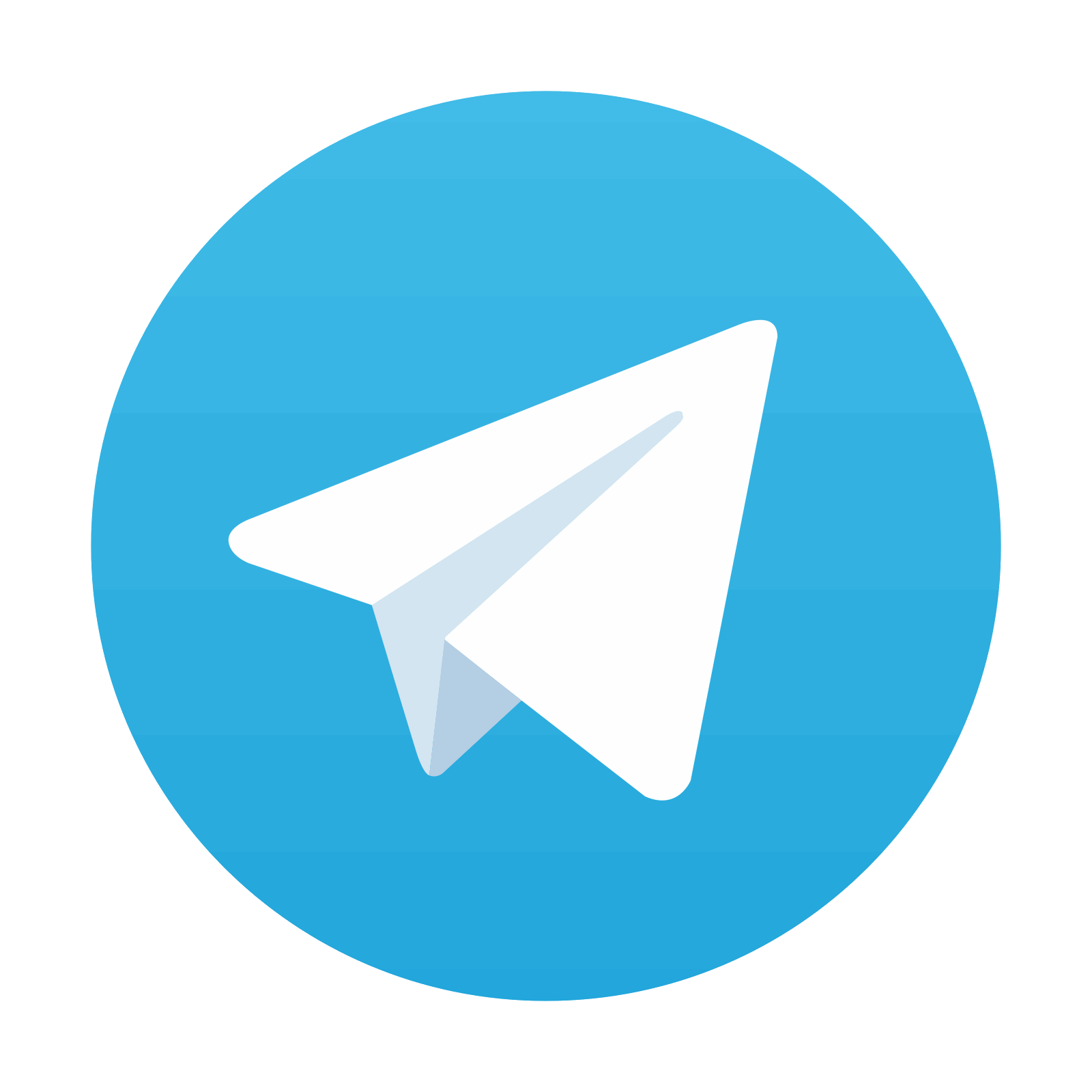
Stay updated, free articles. Join our Telegram channel

Full access? Get Clinical Tree
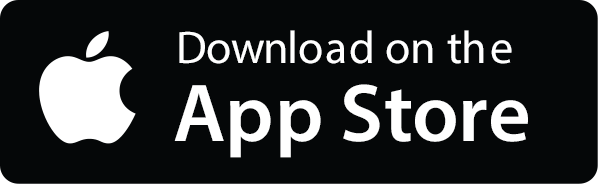
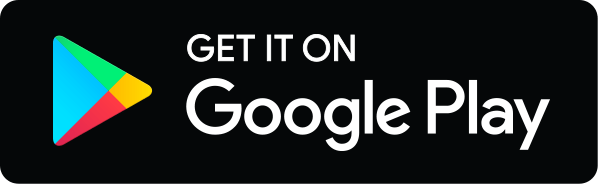