Bone responds to diseases and injuries with either an increase in mineral or a decrease in mineral. Abnormal bone is either too light or too dark in radiographs. Different bone diseases often present with similar radiographic findings. In many cases, a definitive diagnosis is not possible from radiographs alone. Although you do not need patient history and results of laboratory testing to read the radiographs, this information often is useful to interpret your findings. The musculoskeletal structures are evaluated for soft tissue abnormalities as well as abnormal position, size, shape, margination, and opacity of the bones. Knowledge of normal anatomy is essential. Comparison radiographs of the contralateral limb, or of a known normal limb, are helpful to differentiate normal variations from pathology. Also useful are reference materials such as radiology and anatomy textbooks, websites, and models, the latter including skeletons and bone specimens. At the end of this chapter is an appendix with lists of differential diagnoses for numerous radiographic findings. When describing bone lesions, it is important to note their distribution: Soft tissue abnormalities frequently accompany and often precede active bone disease. Recognizing the presence or absence of soft tissue swelling is extremely useful when trying to decide whether the appearance of a bony structure represents a normal anatomic variation, an inactive lesion, or an active disease process. In some cases, the cause of pain or lameness is limited to the soft tissues, such as sprains (ligament injuries), strains (muscle injuries), bruises, inflammation, and others. Conditions that can mimic a soft tissue abnormality include bunching of the skin, superimposed skin folds, and dirt, debris, or fluid on the skin or in the hair coat. Soft tissue swelling may be localized or diffuse. Localized swelling can be caused by a mass. Diffuse swelling often is caused by fluid (e.g., edema, hemorrhage, inflammation, others). Swelling may be identified by the displacement or effacement of the nearby fascial planes. A soft tissue mass is a localized area of swelling with a defined margin. The margin may or may not be visible in radiographs, depending on the opacity interface with the adjacent tissues. A soft tissue mass in muscle or surrounded by fluid, for example, is not distinguished because the opacities are the same. A soft tissue mass must be large enough to distort the border of the muscle or displace a fascial plane to be identified. Types of soft tissue masses include tumor, hematoma, cyst, abscess, granuloma, and lymphadenomegaly, the latter occurring at a known location for a lymph node. Lipomas are fat opacity masses, they often can be distinguished from soft tissue in survey radiographs (Figure 5.1). Many lipomas develop in fascial planes. Mixed opacity lipomas may result from fluid mixing with fat or due to a more aggressive type of lipoma such as dissecting lipoma or liposarcoma. The margins of liposarcomas tend to be indistinct. Figure 5.1 Lipoma. Lateral view of a dog crus depicting a fat opacity mass in the soft tissues caudal to the tibia (arrow). Table 5.1 Epiphyses and apophyses: ages at appearance and fusion An arteriovenous fistula is an abnormal communication between an artery and a vein, which can appear as a soft tissue opacity mass. They occur more often in a limb and may be accompanied by a periosteal response or pressure remodeling in the adjacent bone. Lymphedema is an abnormal accumulation of lymphatic fluid in one or more limbs. Swelling typically is more severe in the distal part of the limb due to the effects of gravity. Unless the edema is due to trauma, the skeletal structures usually are normal. A loss or reduction of soft tissue may be due atrophy or the physical absence of tissue. Atrophy generally results from disuse, which may be due to pain, immobilization, or paralysis. A physical deficit may be caused by severe trauma or surgical removal. Gas in the soft tissues can result from a penetrating wound, migration of gas from a nearby damaged structure (e.g., ruptured trachea), or a gas‐producing bacterial infection. The gas may be localized or diffuse and usually produces a heterogeneous pattern due to multiple bubbles. Soft tissue emphysema can occur in the subcutaneous, intramuscular, or interfascial tissues. The volume of subcutaneous gas sometimes is quite large and widespread, displacing the skin away from the body and making the patient appear bloated. Conditions that may be mistaken for soft tissue emphysema include fat opacity in the soft tissues (e.g., fascial plane, lipoma) and superimposition of skin folds. Mineralization in the soft tissues may be dystrophic, metastatic, neoplastic, or due to foreign material. There are several different mechanisms by which soft tissue structures can mineralize, but they are difficult to differentiate in radiographs. The location of the mineralization and results of laboratory testing sometimes provide clues to the etiology. Soft tissue mineralization ranges from disorganized to highly organized. Clinical signs usually are absent unless a secondary complication develops, such as interference with mobility, ulceration, or infection. Dystrophic mineralization occurs locally in damaged, degenerating, or dead tissue. Serum calcium and phosphorous usually are normal. Metastatic mineralization occurs in normal tissues away from the site of disease. It frequently is associated with abnormal serum calcium and phosphorous, but blood work sometimes is normal. Calcinosis cutis is mineralization in the skin. It most often is associated with hypercortisolism, but it can result from trauma, chronic inflammation, and others. In radiographs, calcinosis cutis typically appears as thin, linear mineralization near the skin surface. It may be focal or multifocal. Calcinosis circumscripta is mineralization in the subcutaneous tissues. The etiology is unknown. It is uncommon in dogs and rare in cats, most often reported in young, large breed dogs. Calcinosis circumscripta tends to occur at bony prominences and under footpads. In radiographs, it appears as well‐defined, rounded clusters of heterogeneous mineralization (Figures 5.2 and 5.3), sometimes called tumoral calcinosis. It often develops near a skeletal structure and may be mistaken for bone disease, but there is no periosteal response nor evidence of osteolysis. Figure 5.2 Calcinosis circumscripta. Lateral view (A) and dorsopalmar view (B) of a dog manus depicting a well‐defined mass of heterogenous mineralization in the soft tissues near the third digit. Careful inspection reveals that the bones are not involved. The digits were gently separated using gauze tied around the toes. Figure 5.3 Calcinosis circumscripta. Lateral view (A) and caudocranial view (B) of a dog humerus depicting heterogenous mineralization in the soft tissues near the elbow and shoulder (arrows). Vascular mineralization occurs in the walls of blood vessels, usually arteries. In dogs, it most often is seen along the abdominal aorta and its major branches. In cats, particularly older animals, it more often occurs in the thoracic aorta. Vascular mineralization frequently is idiopathic, but it may be associated with a systemic or endocrine disease. Mineral and metal opacity foreign materials usually are readily identified in radiographs, but they must be confirmed in orthogonal views to avoid misdiagnosing a superimposition artifact. Soft tissue opacity foreign materials seldom are distinguished unless adjacent to fat or gas (e.g., glass, wood, plastic, plant materials). A fistulogram may help locate a foreign object if a draining tract or site of entry is found. Bone is approximately one‐third organic material (osteoid) and two‐thirds inorganic material (mineral). Osteoid is soft tissue opacity and not differentiated from the adjacent soft tissues. In most cases, only the mineral portion of bone is visible in radiographs. The average number of bones in a dog is about 320 and the average number in a cat is about 240. A typical dog skeleton includes: There are two general types of bone: cortical and cancellous. Cortical bone forms the outer layer or cortex of most bones, including all long bones. Cortical bone is also called compact bone and makes up about 80% of the total bone mass in the body. In radiographs, it is relatively uniform in appearance and homogeneous in opacity (Figure 5.4). Figure 5.4 Cortical and cancellous bone. Lateral view of a dog elbow depicting the appearance of cancellous bone (black arrow) and cortical bone (white arrow). Cancellous bone consists of a network of thin bony plates called trabeculae with many open spaces, or “pores”, in between. Cancellous bone is also called trabecular bone and makes up about 20% of the skeletal mass. Because of the trabeculae, the surface area of cancellous bone is nearly 10 times that of cortical bone. Trabecular bone is found at the ends of long bones, in the vertebrae, and in flat bones. In radiographs, it is more porous and heterogeneous in opacity than cortical bone (Figure 5.4), sometimes described as sponge‐like in appearance. Bones commonly are classified as either long, short, flat, or irregular in shape. Long bones are longer than they are wide. They consist of a shaft and two ends. Long bones are found in the limbs (e.g., humerus, femur, radius, ulna, tibia) where they provide strength and mobility. Short bones are as wide as they are long, sometimes described as cuboidal. They are found in the carpi and tarsi, where they provide stability and some movement. Flat bones are relatively broad and flattened, as the name suggests. They are found in the head, ribs, and limb girdles (e.g., pelvis, scapula), where they provide protection for the internal structures. Irregular bones are more complex in shape and do not fit into the other classifications. These include the vertebrae, many of the bones in the skull, and sesamoid bones. Sesamoid bones are located in tendons where they help reduce strain near a freely moving joint. They are small and usually round or ovoid in shape. Some sesamoid bones are lined on one side with articular cartilage (e.g., patella, palmar sesamoids, plantar sesamoids). Most bones form via endochondral ossification, which means they develop in a cartilaginous frame. Some bones form via intramembranous ossification, which means they grow in connective tissue without an intervening cartilage model. Many of the bones in the skull form via intramembranous ossification. During endochondral ossification, growing cartilage is systematically replaced by bone. The points in the cartilage frame where bone initially forms are called ossification centers. Primary ossification centers develop in the shafts of long bones and in the bodies of short bones, irregular bones, and sesamoid bones. Secondary ossification centers develop at the ends of long bones and form bony prominences, which serve as attachment sites for ligaments, tendons, and other structures. There may be multiple secondary ossification centers in one bone. Secondary centers sometimes fail to fuse to the parent bone and instead develop into separate small, round or oval‐shaped mineral opacity structures. These are called accessory ossification centers or accessory ossicles. The locations of many accessory ossicles are known (often they occur near a joint) and they are described and illustrated in this chapter. Knowledge of accessory ossification centers is important because they may be mistaken for pathology, such as avulsion fractures. Long bones form in a cartilage frame. Longitudinal growth occurs at growth plates called physes, which are located near one or both ends of the bone. A physis appears in radiographs as soft tissue opacity band. The different parts of a long bone are named in relation to the physis (Figure 5.5). Figure 5.5 Parts of a long bone. Craniocaudal view of an immature dog femur depicting normal anatomy. P = physis, E = epiphysis, M = metaphysis, D = diaphysis, A = apophysis, CBZ = cut‐back zone. Notice that the cortical margin is not as sharp in the cut‐back zone. The diaphysis is located “between the physes.” It is the shaft of the long bone, the diaphysis appears in radiographs as homogenous cortical bone on either side of a less opaque medullary cavity. Although cortical bone completely encircles the medullary cavity, the bony cortex appears as two distinct divisions due to the summation of bone (Figure 5.6). Figure 5.6 Long bone cortices. A. Cross‐sectional CT image of a dog’s long bone. Notice that the total thickness of bone through which the x‐rays are passing (red arrows) is much less in the middle than on either side. The cortex is the same thickness all around the medullary cavity, but summation of bone will make parts of the cortex appear more opaque in a two‐dimensional radiograph. B. Lateral view of a dog humerus depicting the more opaque cranial and caudal cortical bone (arrows). The metaphysis is located “next to the physis.” It is the wider end of the shaft of a long bone and consists of mostly cancellous bone. Metaphysis also means “change next to the physis” because this is where the wider bone is actively remodeled to become the narrower shaft. This area of bone remodeling is called the cut‐back zone and appears in radiographs as a part of the cortex with a less distinct margin. The cut‐back zone sometimes is mistaken for pathology; however, there is no soft tissue swelling or pain with normal bone growth. The epiphysis is located “upon the physis.” It is the rounded end of a long bone that supports the articular cartilage. The epiphysis is mostly cancellous bone with a thin layer of dense subchondral bone. The subchondral bone appears in radiographs as a thin line of increased opacity at the articular border. An apophysis is a non‐articular epiphysis. It forms a bony prominence that serves as an attachment site for a tendon, ligament, or joint capsule. Examples include the greater trochanter, greater tubercle, and tibial tuberosity. An apophysis sometimes is called a traction epiphysis because the structures that attach here exert a pulling force, whereas the structures at an epiphysis exert compressive forces due to weight bearing. The parts of growing bones are not visible in radiographs until they ossify. Ossification centers become visible at predictable times in dogs and cats, and the secondary centers are expected to fuse with the parent bone within certain time intervals. These are listed in Table 5.1 and illustrated in Figures 5.7–5.13. Figure 5.7 Normal shoulder epiphysis. Lateral view (A) and craniocaudal view (B) of an immature dog scapulohumeral joint depicting the humeral head (H), supraglenoid tubercle (ST), and caudal glenoid accessory ossification center (CG). Figure 5.8 Normal elbow epiphyses. Lateral view (A), flexed lateral view (B), and craniocaudal view (C) of an immature dog elbow depicting the distal humeral condyle (H), medial epicondyle (ME), olecranon tuber (O), anconeal process (A), and radial head (R). Figure 5.9 Normal carpal epiphyses. Lateral view (A) and dorsopalmar view (B) of an immature dog carpus depicting the distal radial epiphysis (R), distal ulnar epiphysis (U), and accessory carpal bone (Acc). Figure 5.10 Normal pelvic epiphyses. VD view of an immature dog pelvis depicting the femoral head (FH), greater trochanter (GT), lesser trochanter (LT), and ischiatic tuberosity (IT). Figure 5.11 Normal stifle epiphyses. Lateral view (A) and Caudocranial view (B) of an immature dog stifle depicting the femoral condyle (FC), tibial plateau (T), tibial tuberosity (TT), and fibular head (F). Figure 5.12 Normal tarsal epiphyses. Lateral view (A) and dorsoplantar view (B) of an immature dog tarsus depicting the distal fibular epiphysis (F, also called the lateral malleolus), the distal tibial epiphysis (T, also called the medial malleolus), and the calcaneal tuberosity (C, also called the tuber calcis). Figure 5.13 Normal digit epiphyses. Lateral view (A) of an immature dog pes and dorsopalmar view (B) of an immature dog manus. Depicted are the epiphyses in the metatarsal/metacarpal bones (M) and in the phalanges (P). Notice that there are only distal metacarpal and metatarsal epiphyses and only proximal phalangeal epiphyses. In the DP view, the first digit phalangeal epiphysis is labeled. Bone growth ceases when cartilage growth stops. After the cartilage stops growing, the metaphyses and epiphyses gradually blend together and the physes become thinner and thinner until the physes “close.” A “closed” physis appears in radiographs as a thin sclerotic line which is called a physeal scar. The physeal scar slowly fades as the animal matures and eventually disappears. Nearly all bone surfaces are covered with a thin membrane called the periosteum. The articular surfaces and most of the sesamoid bones are not covered with periosteum. The periosteum consists of two layers, a tough fibrous outer layer and an inner layer that produces bone. The outer layer provides protection and connection, the latter because it blends continuously with tendons and ligaments at their attachment sites. The inner layer of the periosteum, called the cambium layer, is attached to the bone cortex by Sharpey’s fibers. The periosteum contributes to the circumferential growth of long bones and aids in bone healing. Bone responds to disease with either an increase in mineral or a decrease in mineral, and often it is both. At least 30%–50% of the mineral content must change before a bone lesion can be seen in a radiograph. This amount of change typically requires at least one to two weeks to occur. Bone production is a non‐specific periosteal response to bone damage. The formation of new bone is an attempt to heal, not a “reaction” to any particular disease. Whenever the periosteum is separated from the cortex and maintains its blood supply, it will produce mineral to fill the space between the lifted periosteum and the cortex of the bone. Mineral is produced by the cambium layer and deposited along the fibrous and vascular tissues that remain connected to the cortex. The formation of new bone, therefore, is perpendicular to the periosteum. The further the periosteum is separated from the cortex, the larger and more irregular the new bone production because there is more space to fill. The stages of a periosteal response are illustrated in Table 5.2. Table 5.2 Stages of periosteal response When you see a periosteal response, the first thing to determine is whether it is active or inactive. If it is active, you must deal with the bone disease now. If it is inactive, you can observe the lesion to see whether it remains inactive (i.e., a benign process). It is important to make sure an apparently benign lesion remains inactive before abandoning diagnostics or treatment. To determine whether new bone production is active or inactive, look at its margin. The less distinct the margin, the more active the periosteal response. The simplest way to evaluate a bony margin is to trace it with an imaginary pencil or stylus. If it is easy to draw a line along the edge of the new bone, it is well‐defined and not very active. The more difficult it is to trace the margin, the more active the disease process. Active disease processes continue to push the periosteum away from the cortex. The subperiosteal space may fill with blood, tumor cells, edema, or purulent material, any of which will prevent complete mineralization of the subperiosteal space. As long as the disease process is active, the edges of the new bone being produced will remain indistinct. A well‐defined margin can only be established once the disease process has stopped and there is time for mineral deposits to fill the space. The better defined the margin, the less active the process. It is essential to realize that the definition or sharpness of the new bone margin has nothing to do with its size or shape. New bone with an “irregular” border does not mean the margin is ill‐defined, and new bone with a “smooth” border does not mean the margin is well‐defined. Some periosteal responses are quite extensive, producing large amounts of new bone with irregular or uneven borders, but this does not tell us whether the disease is active or inactive. It may give us some idea about the severity of the bone damage, but we must evaluate the margin to determine whether further diagnostics or treatment are immediately needed. The aggressiveness of a bone lesion is reflected by the degree of osteolysis. Osteolysis is the pathologic destruction of bone. The less distinct the margin around an area of osteolysis, the more aggressive the disease process. In addition to tracing the margin to determine its sharpness, look at the zone of transition between the abnormal bone and bone that appears normal. The longer and less defined the zone between abnormal and normal, the more aggressive the disease process. The aggressiveness of a bone lesion is also assessed by its rate of change in serial radiographs. The more rapid the change, the more aggressive the disease. Very aggressive bone diseases can produce visible osteolysis in 5–7 days. Bone production, however, usually takes at least 7 to 10 days to become visible in a radiograph, regardless of the cause of the periosteal response. Occasionally, periosteal new bone production is visible in less than 7 days in a young, rapidly growing animal. Note: a periosteal response does not tell us how aggressive the disease is, only how active. The radiographic appearance of periosteal new bone also tells us something about its chronicity. New bone production initially is more opaque near the displaced periosteum because this is where it is being formed. As healing progresses and more mineral is deposited, the new bone increases in opacity and becomes more homogeneous. New bone gradually matures over the following weeks, becoming more organized and trabeculated. During the following months and years, normal stresses gradually remodel the bone until it eventually resembles the original size and shape. The change from unorganized mineral deposits to organized bone reveals the chronicity of the lesion. Knowing how long a lesion has been present is valuable for patient management and may provide clues to its etiology. With many bone diseases, it is a race between the pathologic process and the healing response. Serial radiographs help monitor the progression of disease and the patient’s response to therapy. Patterns of bone production and bone destruction have been used over the years in an attempt to narrow the list of differential diagnoses. Most patterns, however, are not diagnostic of any specific disease. Patterns sometimes are helpful to describe radiographic findings, and some of the classic patterns are discussed on the following pages. However, any aggressive disease process, regardless of etiology (e.g., malignant tumor, bacterial infection, severe trauma) can produce radiographic signs of aggressive bone disease. Likewise, any benign disease process, regardless of etiology (e.g., bone bruise, bone cyst, tumor) can produce radiographic signs of benign bone disease. The value of radiography is to help determine the activity, aggressiveness, chronicity, and extent of bone disease. Bone lesions may be solitary or multifocal, monostotic or polyostotic, and may arise in the diaphyseal, metaphyseal, or epiphyseal region of a bone. It is important to remember that radiographs are snapshots in time. They depict the appearance of a lesion only at the moment the images were made. Serial radiographs often are needed to determine the true nature of a disease process. In addition, some diseases may be obscured by a pre‐existing condition, such as degenerative joint disease masking a tumor. Patterns of bone production tend to emphasize the shape of the new bone rather than its margination. Remember, the shape is not the same as the margination. In all of the patterns discussed below, the margins of the new bone may be well‐defined or ill‐defined, depending on how active the disease process was at the time of radiography. Note: it is the bony margin at the edge of the periosteal response, away from the underlying cortex, that must be evaluated to determine lesion activity. Lesion aggressiveness (i.e., osteolysis) is determined by evaluating the cortex and underlying bone. Smooth bordered new bone production generally occurs when the periosteum was not separated very far from the cortex. This may occur with a slow‐growing disease process or early in the course of a more aggressive disease. When the periosteum is only mildly elevated from the cortex, mineral can quickly fill the subperiosteal space to produce new bone with a smooth, even border. Again, the margin may be well‐defined or ill‐defined, depending on the activity of the disease process. Uneven or irregular‐shaped new bone production occurs when at some point, the periosteum was separated a considerable distance from the cortex (Figure 5.14). Figure 5.14 Irregular periosteal response. Lateral view of a section of a long bone depicting a large amount of homogeneous new bone production. The periosteum was separated a relatively long distance from the cortex. The new bone margin is irregular, but well‐defined, indicating this is an inactive process. Multilayered new bone production occurs with diseases that grow intermittently, each time elevating the periosteum. New bone is produced in layers between growth episodes of the disease. The radiographic appearance has been described as lamellar, laminated, or onion skin. The shape of the new bone tends to be even and smooth‐bordered (Figure 5.15). Again, the margin of the outer‐most layer may be well‐defined or ill‐defined, depending on the current activity of the disease. Figure 5.15 Lamellated periosteal response. Lateral view of a section of a long bone depicting layers of periosteal new bone production. The new bone is organized, indicating this is a chronic process. The outer margin is smooth and well‐defined, indicating it is currently inactive. Brush border new bone production occurs when the periosteum is elevated along a broad expanse of cortex. Mineralization is perpendicular to the periosteum, creating an appearance which has been described as resembling the bristles of brush or “hairs‐standing‐on‐end” (Figure 5.16). If the separation between the periosteum and the cortex is larger and more chronic, the new bone may be more organized and appear “columnar” or “palisading.” Figure 5.16 Brush border periosteal response. Lateral view of a section of a long bone depicting periosteal new bone that is perpendicular to the periosteum and cortex. The margin is indistinct indicating this is an active bone lesion. The sunburst pattern of new bone production occurs when the periosteum is separated from the cortex in all directions. This typically is caused by a rapidly growing lesion that originates at a single site. Periosteal mineral is produced perpendicular to the elevated and rounded periosteum and extends toward the cortex. The mineralization is not perpendicular to the cortex, rather it appears to radiate from the center of the lesion as numerous bony spicules that resemble a “sunburst” (Figure 5.17). A sunburst periosteal response most often is attributed to neoplasia, but it can occur with severe osteomyelitis or trauma. Figure 5.17 Sunburst periosteal response. Lateral view of a section of a long bone depicting spicules of mineralization appearing to radiate from a localized area in the diaphyseal region (arrow). The margins of the periosteal response are hazy and ill‐defined and there is cortical destruction, indicating an active and aggressive bone lesion. Amorphous new bone production occurs with very rapidly growing disease processes that actually break through the periosteum. In these cases, the elevated periosteum is ruptured and discontinuous. Parts of the periosteum that retain a blood supply produce perpendicular mineralization which appears in radiographs as multiple mineral opacity foci without any form or organization (Figure 5.22). Amorphous new bone tends to develop further away from the cortex than most other periosteal responses. It most often is seen with aggressive tumors or severe trauma. Codman’s triangle is another finding that occurs with very rapidly growing disease processes and frequently is attributed to neoplasia. It is caused by continued growth of the disease that elevates the periosteum again, lifting the newly formed bone away from the cortex. Codman’s triangle appears as a small, well‐defined, wedge‐shaped area of mineralization at the periphery of the lesion (Figure 5.18). The triangular‐shaped mineralization may be separated from the cortex by soft tissue opacity material (e.g., fluid, cells) or the mineralization may extend to the cortex. Because the margin associated with Codman’s triangle is well‐defined, the disease process may be erroneously diagnosed as inactive. But the triangle occurs at the periphery of the actual lesion and does not represent the true character of the disease. Figure 5.18 Codman’s triangle. Lateral views of a section of a long bone depicting an aggressive lesion. Codman’s triangle is visible at the periphery of the lesion (arrows) and may appear as elevated mineralization with soft tissue opacity underneath, as shown in A, or as mineralization that extends to the cortex, as shown in B. Patterns of bone destruction generally emphasize the sizes of the contiguous osteolytic lesions. However, it is the sharpness of the margins that is the key to determine aggressiveness. There are three classic patterns of osteolysis. From least aggressive to most aggressive, they are: geographic, moth‐eaten, and permeative. In many bone lesions, there is more than one pattern present. The most aggressive pattern determines the true nature of the disease process. A geographic pattern of osteolysis refers to a larger area of bone loss, over 10 mm in size (Figure 5.19). It often is caused by a benign condition but can result from aggressive disease. Benign is differentiated from aggressive by the sharpness of the margin and the zone of transition between abnormal and normal bone. A well‐defined osteolytic margin suggests a slow‐growing lesion (e.g., bone cyst, benign tumor, pressure remodeling). In these cases, the area of bone loss may be surrounded by osteosclerosis, indicating that the body actually is “reacting” to the disease. A slow‐growing lesion may cause thinning or outward expansion of the adjacent cortex, but rarely will the cortex be disrupted. Geographic osteolysis with an ill‐defined margin and a longer zone of transition generally results from the coalescence of moth‐eaten and permeative osteolysis. Figure 5.19 Geographic osteolysis. Lateral view of a dog shoulder depicting a large, solitary area of osteolysis in the proximal diaphyseal region of the humerus (arrow). The margins are well‐defined with a short zone of transition between abnormal and normal bone. A thin rim of osteosclerosis surrounds the lesion. The adjacent cortex is thinned and expanded outward, but remains intact. There is no soft tissue swelling. This is a benign lesion, possibly a bone cyst. A moth‐eaten pattern of osteolysis appears as multiple smaller areas of bone loss, each about 3–10 mm in size. The margins usually are indistinct with a longer zone of transition from abnormal bone to normal bone (Figure 5.20). A moth‐eaten pattern tends to occur with more aggressive disease processes (e.g., neoplasia, osteomyelitis). The adjacent cortex may be thin and irregular in shape, sometimes with a disrupted border. Figure 5.20 Moth‐eaten osteolysis. Lateral view of a dog shoulder depicting multiple small areas of osteolysis in the metaphyseal region of the humerus (arrow). The margins are indistinct, and the adjacent cortex is thin and less opaque. There are soft tissues swelling. This is an aggressive lesion, possibly due to neoplasia or osteomyelitis. A permeative pattern of osteolysis appears as numerous, tiny areas of bone loss, 1–2 mm in size, with no clear distinction between normal and abnormal bone (Figure 5.21). These pinpoint lesions are caused by a very aggressive disease process (e.g., malignant neoplasia, severe osteomyelitis). They may be difficult to detect until a larger area of osteolysis has developed. The adjacent cortex often is thin and less distinct, or it may not be visible at all due to severe disruption by the disease. Figure 5.21 Permeative osteolysis. Lateral view of a dog shoulder depicting numerous, tiny foci of osteolysis in the proximal humerus (arrow). These foci are difficult to distinguish from normal bone until they coalesce to become larger osteolytic areas. The adjacent cortex is indistinct and diminished in opacity. The adjacent soft tissues are swollen. This is a very aggressive bone lesion due to malignant neoplasia or severe osteomyelitis. Mixed patterns of osteolysis are common, especially with aggressive diseases (Figure 5.22). In many cases, osteolysis is a continuum of patterns, with permeative progressing to moth‐eaten and then to geographic bone loss. In the race between pathology and healing, one pattern may change to another as the disease process waxes and wanes over time. As long as the disease remains unchecked, bone destruction will continue, and margins will remain ill‐defined with a longer zone of transition. The formation of new bone may be associated with growth, healing, or metabolic disease. In radiographs, new bone production appears as increased opacity. Bone growth and development have been discussed. Bone healing generally involves a periosteal response, which also has been discussed. It is worth mentioning that bone healing includes an endosteal response, which is similar to a periosteal response but occurs along the inner surface of the cortex. Endosteal bone production tends to be less evident in radiographs than periosteal bone production. A frequently encountered type of bone production is an exostosis. An exostosis is any abnormal mineralized structure that extends outward from the surface of a bone. The term is not specific to any condition or disease; however, exostoses are important to recognize, and they must be differentiated from normal prominences. The most common exostoses are osteophytes and enthesophytes, which are discussed in greater detail with joint disease. Osteosclerosis is new bone production within the bony matrix. It leads to thicker trabeculae and smaller spaces between them, both of which increase bone opacity (Figure 5.23). Osteosclerosis is caused by an increase in mineral deposit, a reduction in the resorption of mineral, or both. It may be a localized attempt to heal a lesion, as the body tries to wall‐off or repair the damaged part of the bone, or it may be diffuse due to abnormal bone metabolism (i.e., osteopetrosis). In radiographs, localized osteosclerosis may appear as a rim of increased opacity adjacent to a bone lesion. It sometimes is seen in subchondral bone near a damaged joint. The presence of osteosclerosis can help differentiate osteomyelitis from osseous neoplasia because the body is much more likely to wall off an infectious agent than a tumor. Histopathology, however, usually is necessary for definitive diagnosis. Osteopetrosis is a form of osteosclerosis that affects the entire skeleton. It results from decreased resorption of bone due to abnormal osteoclastic activity. In radiographs, the bones are relatively normal in length and profile but increased in opacity. The appearance has been described as “ivory,” “marble,” or “chalky” bones (Figure 5.24). The cortices are thickened and the medullary cavities are narrowed. The corticomedullary contrast is less distinct. In cancellous bone, the trabeculae become thickened and the spaces obscured by the increased mineral. Osteopetrosis is a rare congenital disease in dogs and cats. It results in brittle bones that lead to pathologic fractures and it causes anemia due to reduced bone marrow function. Lead poisoning in young animals can produce thin, transverse lines of osteosclerosis in the metaphyseal regions of long bones. The lines form parallel to the physes and most often are seen in the distal radius and ulna. They also have been reported in the vertebrae. Chronic lead poisoning (plumbism) can lead to osteopenia. Growth arrest lines are thin, transverse lines of osteosclerosis in the diaphyseal regions of long bones (Figure 5.25). They are caused by changes in the rate of growth in an immature bone, which may result from a systemic illness, dietary change, or other stressor. The lines are sharply‐defined and seen most often in the femurs, usually bilaterally. There is no soft tissue swelling, periosteal response, or evidence of active bone remodeling. The importance of growth arrest lines is their potential to mimic pathology, such as a fracture, bone infarct, or panosteitis. Bone infarcts appear in radiographs as distinct foci of osteosclerosis that are irregular in shape with well‐defined margins (Figure 5.26). They most often are seen in the medullary region of a long bone, generally distal to the elbow or stifle. Bone infarcts usually are multiple and may be monostotic or polyostotic. They result from a localized loss of blood supply, typically caused by embolic disease (e.g., neoplasia, trauma, vasculitis, recent orthopedic surgery). Most infarcts are asymptomatic. The loss of mineral from bone may be due to increased resorption, reduced deposition, or active bone destruction. Sometimes it is a combination of these. The term osteopenia means “too little bone” and is used to describe diminished bone opacity. Osteopenia may result from osteolysis, osteoporosis, or osteomalacia. Osteolysis was discussed earlier as it pertains to the aggressiveness of bone lesions. Specifically, it is the pathologic destruction of bone that results in loss of both mineral and matrix. Causes of osteolysis include neoplasia, infection, and pressure necrosis. Osteoporosis means “porous bone” and refers to the loss of bone mineral due to an imbalance between bone formation and bone resorption. Osteoporosis that affects the entire skeleton and is caused by a systemic disease, such as hyperparathyroidism. Osteoporosis in a single limb is caused by disuse (e.g., immobilization, pain, paralysis). Generalized osteoporosis tends to initially be more evident in the vertebrae, followed by the mandible and then the long bones. Osteoporosis in a single limb initially is more severe in the distal bones (e.g., distal epiphyses, cuboidal bones). Osteoporosis sometimes is called “bone atrophy” and leads to thin, weak bones that are prone to pathologic fractures (Figure 5.23). Osteomalacia means “soft bone” and refers to the loss of bone mineral due to faulty ossification. The most common cause is a dietary deficiency (e.g., Vitamin D, calcium, phosphorous). Osteomalacic bones are soft, but many affected dogs and cats do not exhibit orthopedic signs of disease. In immature animals, osteomalacia is called rickets. Osteoporosis and osteomalacia rarely can be differentiated in radiographs. The term osteopenia applies to both. Osteopenic bones are less opaque and there is less contrast between bone and soft tissues (Figure 5.27). The cortices become thin and faint, sometimes developing a “double cortical line” due to intracortical resorption of bone. Cancellous bone becomes more porous in appearance due to the larger spaces and thinner trabeculae, commonly described as a “coarse trabecular pattern.” Corticomedullary contrast is diminished. A fracture is a break or a discontinuity in a bone. It is caused by a physical force that exceeds the bone’s structural capacity. Fractures are more likely in bone that has been weakened by disease. Soft tissue swelling usually accompanies a fracture, especially in the acute stages, which is helpful to distinguish pathology from normal anatomy or an imaging artifact. Knowledge of sesamoid bones, accessory ossification centers, nutrient foramina, growth plates, and the normal shapes of bones is essential to avoid a misdiagnosis. Non‐displaced fractures may not be evident in initial radiographs but may be detected in a follow‐up study when a periosteal response develops or when the fracture line widens during healing or as a result of displacement. The radiographic description of a fracture should include the type of fracture, the parts of the bones involved, the degree of displacement and rotation, whether the fracture is articular, and an assessment of the adjacent soft tissues (Box 5.1). Most fracture lines are less opaque than the adjacent bone. The fracture sometimes is more opaque than adjacent bone due to summation of overlying fracture fragments (e.g., compression fracture, folding fracture). Incomplete fractures are those in which the cortex is broken on only one side and the bone is not in complete discontinuity. When the cortex is broken on the convex side, it is called a greenstick or hairline fracture (Figure 5.28). If the cortex is broken on the concave side, it is a folding, torus, or buckling fracture (Figure 5.29). An incomplete fracture in a fracture fragment is called a fissure fracture (Figure 5.31), which is important to identify because a fissure can become a complete fracture during reduction and repair. Stress or fatigue fractures are caused by repetitive cycling injuries that damage the bone at a rate faster than it can heal. An incomplete fracture may not be visible in radiographs unless the x‐ray beam is aligned with the fracture line. Complete fractures are those in which the fracture line extends through the entire bone. A simple complete fracture consists of one fracture line and two fragments (Figure 5.30). In a complex complete fracture, there are multiple, non‐continuous fracture lines and more than two fragments (Figure 5.31). The fracture is comminuted if all of the fracture lines communicate at a single point. Comminuted fractures consist of three or more fragments. More than five fragments is considered highly comminuted. Fragments that are wedge‐shaped commonly are called “butterfly” fragments. In a segmental fracture, the fracture lines do not communicate and the result is one or more isolated segments of bone (Figure 5.32). Fractures in paired long bones sometimes are accompanied by a luxation in the adjacent joint. A Monteggia fracture, for example, is a fracture in the proximal 1/3 of the ulna with a concurrent luxation of the radial head. It is important to identify the luxation prior to treating the fracture. Closed fractures are those in which the overlying skin and soft tissues are intact. Open fractures occur when the overlying tissues are perforated (also called compound fractures). Open fractures usually are accompanied by subcutaneous emphysema and are at increased risk for infection (Figure 5.33). T or Y fractures describe fractures in which the bone splits both longitudinally and transversely (Figure 5.34). Avulsion fractures occur at attachment sites for ligaments, tendons, or joint capsules. A piece of bone is pulled away from main bone (Figure 5.35). The origin or “bed” of an avulsed fragment may be visible as a similar‐sized defect in the main bone. Compression fractures are those in which the ends of the bone fragments are jammed into each other resulting in a shortened or collapsed bone. The fracture line may or may not be visible in radiographs. When visible, the line may be less opaque or more opaque than the adjacent bone. Compression or impaction fractures most often are seen in the vertebral bodies (Figure 5.210) and in the short bones in the carpus or tarsus. Chip fracture is a descriptive term that means “knocking off a corner.” A small fragment is displaced from the main bone. Chip fractures occur most often in a short bone in the carpus or tarsus. They usually are articular. The site of origin or bed for the fracture fragment may or may not be identified. Slab fracture is a descriptive term that means “knocking off a chunk.” As with chip fractures, they occur most often in a short bone in a tarsus or carpus. Slab fractures involve the articular surfaces on both ends of the bone. If the fracture involves only one articular surface, it is a chip fracture. Shearing fractures are caused by severe friction or a glancing trauma, such as an animal being dragged on pavement. Also called abrasion fractures, these are open fractures due to loss of overlying soft tissue. Pathologic fractures are caused by normal stresses on abnormal bone. They occur in bone that has been weakened by an underlying disease or a developmental defect. Disease may be systemic (e.g., osteoporosis) or localized (e.g., tumor, cyst). In radiographs, the bone opacity at or near the fracture site usually is diminished and a periosteal response may be present, earlier than expected for normal fracture healing (Figure 5.42). Pathologic fractures tend to occur at the periphery of the abnormal part of the bone. Physeal fractures occur in immature bones. They are classified based on the degree of physeal, metaphyseal, and epiphyseal involvement using the popular method proposed by Salter and Harris (Table 5.3). The Salter–Harris classification ranks physeal fractures according to the increasing probability (from less likely to more likely) of a growth deformity during fracture healing. Table 5.3 Salter–Harris classification of fractures Fracture healing may be primary or secondary. Primary healing occurs when the fragments are in close apposition and the cortex is reestablished without the formation of a callus. This requires rigid stabilization and excellent anatomic alignment. Secondary fracture healing is more common and involves callus formation and subsequent remodeling (Table 5.4). Table 5.4 Stages of unncomplicated fracture healing After a fracture occurs, bleeding from ruptured blood vessels results in a hematoma at the fracture site. The hematoma provides a temporary framework for subsequent healing. Within the framework, granulation tissue develops and a fibrocartilaginous callus forms, bridging the fracture fragments. This callus is soft tissue opacity and not visible in radiographs. It gradually becomes visible as mineral deposits are added from the periosteum and endosteum. The ends of the fracture fragments often become less distinct during early fracture healing due to active bone remodeling. The fracture line initially widens as bone is resorbed and then begins to narrow as bone is produced. As the fracture fills with mineral, it gradually disappears over the next 3–4 weeks. During this time, the callus continues to mineralize, increasing in opacity and making it better defined. Over the next few months, the new bone matures and develops trabeculation. The cortical margins become more distinct and the medullary cavity gradually is reestablished. The bone eventually resembles its normal appearance. Soft tissue swelling and emphysema that were present at the time of injury or introduced during open reduction and stabilization usually resolve during the first 5–10 days, unless there is an infection. A fracture may be considered radiographically healed when the fracture line is no longer visible, the cortex is continuous and uninterrupted, and an ossified callus completely bridges the fracture fragments. Removal of fixation devices may be considered when there is radiographic evidence of a bridging bony callus. Multiple factors affect fracture healing, including the blood supply, the alignment and stability of the fracture fragments, and the overall health of the patient. An adequate blood supply is important to callus formation and periosteal/endosteal bone production. A loss of blood supply may be due to severe tissue damage or removal of the hematoma. Intramedullary implants can disrupt endosteal blood supply and delay healing. Prolonged disuse of a fractured limb can lead to reduced blood flow and delayed healing. The apposition of the fracture fragments is important to healing. In general, at least 50% contact between bone fragments is needed for efficient healing. Spiral and oblique fractures tend to heal faster than transverse fractures due to the increased area of contact. The smaller the gap between fragments and the less movement at the fracture site, the quicker the bone will heal. Some bones naturally heal more slowly than others (e.g., distal radius, ulna, tibia). Delayed union is fracture healing that takes longer than expected, but the bone eventually heals, both clinically and radiographically. Although no definite timetable exists, healing generally is considered delayed if bridging callus is not evident within 6–8 weeks (Figure 5.36). Nonunion occurs when fracture healing stops before there is complete union. A fracture generally is considered a nonunion when there is no progression of callus formation after 6 months, and no further healing is expected unless intervention occurs. In radiographs, there is no evidence of active bone remodeling for several weeks; the fracture margins remain sharp and well‐defined (Figure 5.37). A hypertrophic nonunion results from chronic instability at the fracture site that prevents callus from bridging the fragments. Organized but inactive new bone builds up at the ends of the fracture fragments. The new bone tends to flair out from the cortical ends. In some cases, the ends of the fragments appear to “fit together” because of motion (e.g., one end becomes convex and the other concave) but there is a gap separating the fragments. An atrophic nonunion occurs when the fracture fragments are chronically separated. There is no callus formation. The ends of the fragments appear thin and tapered due to bone resorption. Atrophic nonunions occur more often in small breed dogs and usually are accompanied by disuse osteopenia in the affected limb. A dystrophic nonunion is caused by loss of blood supply to one or both fragments. The end of the affected fragment remains sharp, well‐defined, and pointed due to a lack of bone remodeling. If the blood supply is only diminished and not completely lost, some remodeling may occur and the fracture ends may appear more rounded and sclerotic (called a nonviable nonunion). Complete loss of blood supply is called a necrotic nonunion. Malunion fractures are those that are healed but with abnormal bone geometry (Figure 5.38). Abnormal geometry can lead to increased stress at the proximal or/and distal joint and may impair limb function. The healed bone fragments may be angled, rotated, shortened, or otherwise malaligned. Comparison radiographs of the contralateral limb may be useful to assess the degree of limb deformity. A sequestrum is a fragment of bone that is no longer viable due to loss of its blood supply. It appears as a sharply‐defined, sclerotic piece of bone that is not incorporated in the callus and exhibits little or no remodeling (Figure 5.39). A sequestrum may be located within its bone of origin or separate from it. Fragments that remain in the bone sometimes are located in a lacuna, which is a pocket of necrotic soft tissue. The lacuna often is surrounded by a rim of sclerotic bone called an involucrum. In some cases, a draining tract extends from the involucrum to the skin surface where it opens as a cloaca. The tract may be demonstrated in radiographs using fistulography (Figure 2.96). Sequestra are more likely to occur as a complication of osteomyelitis or in a necrotic nonunion, and rarely with neoplasia (i.e., osteosarcoma). A pseudoarthrosis or “false joint” sometimes develops at a nonunion fracture site. It occurs when the fibrocartilage between the fracture fragments forms into a capsule that fills with serum. The pseudoarthrosis may allow the patient some use of the limb. Occasionally a bone fragment will fuse to an adjacent bone. This is called a synostosis and can result in loss of movement. Any physis in any bone can be injured and may lead to a growth deformity in the bone. The degree of the deformity depends on the age of the animal at the time of injury, which physis was affected, and the severity of the damage. Physeal injuries that lead to limb deformities may be unilateral or bilateral. Bilateral conditions may or may not be symmetrical. Damage to a physis most often is due to trauma. The traumatic incident may or may not be known at the time of diagnosis. The younger the patient when the damage occurs, the greater the potential for limb deformity. In many cases, the severity of physeal damage is not evident until bone growth stops (i.e., all the physes are closed). Therefore, the earlier a damaged physis is detected, the greater the possibility for successful treatment. Physeal damage affects the rate of bone growth. If the entire physis is damaged, growth may either be delayed or stopped completely. If only part of a physis is damaged, that side may grow more slowly than the other. Abnormal rates of growth are most significant when paired bones are involved (i.e., radius and ulna, tibia and fibula). It is important to realize that complete cessation of long bone growth is not necessary to produce a limb deformity, merely a differential in the rate of growth between the two bones. Asynchronous growth can lead to abnormal shortening, bowing, and angulation of one or both bones. Limb deformities can lead to abnormal joint stresses, subluxation, and degenerative joint disease. Clinical signs tend to be more significant in young, large, and giant breed dogs due to their greater and faster bone growth. Certain dog breeds are deliberately bred to exhibit asynchronous growth as a desired trait (e.g., Bassett Hound, Dachshund). In radiographs, the damaged part of a physis may or may not be visible. When visible, the damaged physis typically appears thinner and more opaque than the other physes, a finding commonly referred to as “premature closure” of the physis. Note: be cautious when interpreting subtle signs of a thinner, more opaque than expected physis because the normal physes can vary in appearance, even when comparing contralateral limbs. Experience is needed to recognize normal physeal variations and avoid overdiagnosis. Serial radiographs sometimes are needed. The important point is to carefully inspect the physes in a deformed limb to determine whether physeal damage and asynchronous growth might be the cause of the deformity. Asynchronous growth is discussed in greater detail in this chapter with Congenital and Developmental Abnormalities. If a physis is not visible at all, it likely is “closed,” and no further growth should be expected from that site. Inflammation of bone tissue is called osteitis. If inflammation includes the medullary cavity, it is called osteomyelitis. Inflammation sometimes starts in the medullary cavity, especially if the cause is hematogenous in origin. If due to a penetrating wound, inflammation can start anywhere from the skin to the medullary cavity. Osteomyelitis most often is caused by an infection, either bacterial, mycotic, or protozoal. Infection may be monostotic (all lesions in one bone), regional (all lesions in one limb), or polyostotic (lesions in multiple bones and multiple limbs). The earliest radiographic sign of osteomyelitis is soft tissue swelling. Bone lesions may become visible after about 1–2 weeks, sometimes sooner in very young animals. Bone lesions caused by active inflammation typically appear as an ill‐defined periosteal response. Osteolysis may develop if inflammation remains active and unchecked by either body defenses or appropriate therapy. Bone weakened by osteolysis is susceptible to pathologic fracture. In chronic cases, osteosclerosis surrounds the lytic areas as the body attempts to wall‐off the infection. Osteomyelitis, whether due to a bacterial or mycotic infection, rarely extends into the joints. Bacterial osteomyelitis tends to stimulate a greater periosteal response than a mycotic infection or a tumor. Elevation of the periosteum is caused by subperiosteal accumulation of fluid (e.g., purulent, hemorrhagic, serous). In radiographs, the subperiosteal fluid may separate the periosteal new bone from the underlying cortex. Bacterial infections tend to result in a more rapid accumulation of subperiosteal fluid which can spread proximally and distally along the diaphyses of the long bones (Figure 5.40). In some cases, the entire diaphysis will be involved. Bacterial osteomyelitis typically affects a single limb, but multiple bones in that limb may be involved (Figure 5.41). Bacterial infection can result from a penetrating wound, extension of an infection in the adjacent tissues, or hematogenous spread. Polyostotic bacterial infections are less common, typically resulting from a systemic infection. Lesions can occur in any part of any bone, but they are more likely in areas with a rich blood flow, such as the metaphyses in immature animals and the vertebrae and ribs in older animals. Occasionally a bone abscess develops, appearing as a focal, sharply marginated area of decreased opacity. Chronic infections can lead to development of a fistulous tract. Mycotic osteomyelitis usually is hematogenous in origin and polyostotic in distribution. Mycosis in a single bone is uncommon, but when it occurs the ends of the bone (metaphyseal or epiphyseal region) are more likely to be affected. Mycotic infection at the end of a bone often is indistinguishable from a bone tumor, although mycoses tend to more osteoproductive and neoplasms more osteolytic. Mycotic osteomyelitis tends to produce a smaller and better defined periosteal response that bacterial infections and rarely involves the entire diaphysis, as often is seen with bacterial infections. Fungal infections typically grow more slowly and periosteal elevation is more gradual. Mycotic osteomyelitis is more common in endemic regions. It is reported more often in dogs than in cats, particularly in young adult, large‐breed dogs used for hunting. Animals that are immunocompromised are at increased risk. Protozoal osteomyelitis is uncommon in dogs and cats. Parasites that may infect bone include hepatozoonosis, leishmaniasis, and neosporosis. Protozoa may enter bone via hematogenous spread or from the adjacent soft tissues. Lesions usually are polyostotic and aggressive. Any bone may be affected, but the long bones most often are involved. Periosteal new bone may be ill‐defined (active process) or well‐defined (inactive disease) and varies in appearance from smooth and laminar to very irregular and proliferative. Neoplasia can involve any part of any bone. Most bone tumors are both osteolytic and osteoproductive, but they tend to be more lytic and less productive than bone infections. Unlike with an infection, the body does not react to a neoplasm (Table 5.5). No defense is mounted against a bone tumor. The body only responds to the separation of the periosteum, which is caused by the tumor physically elevating it. That said, differentiating osseous neoplasia from osteomyelitis often is difficult using radiographs alone. It has been taught traditionally that bone tumors do not cross a joint and infections can cross a joint. This may be true much of the time, but not always. In radiographs of any particular patient, you cannot be sure whether the lesion you are seeing is a tumor that did cross the joint or an infection that did not. Histopathology usually is needed for definitive diagnosis. Serial radiographs are useful to assess the rate of disease progression, especially when the initial findings are equivocal. Table 5.5 General considerations comparing neoplasia and osteomyelitis* * A primary difference between a bone tumor and a bone infection is that the body does not react to a tumor, it only responds to the elevation of the periosteum and the destructive forces of the tumor. The body does, however, react to infection by mounting a defense against the etiologic agent. It is sometimes difficult for the body to curtail bacterial infections and they can spread up and down the diaphysis of the bone(s). Mycotic infections are slower growing, giving the body more time to limit their spread and confine them to a more focal area of bone. Tumors also are focal, but tumors tend to be more osteolytic than mycoses; mycoses tend to be more osteoproductive. Primary bone tumors originate in bone. They rarely extend directly into an adjacent bone. Larger, advanced tumors can irritate a nearby bone and cause a periosteal response, but the response is much less severe than at the primary site and there is no cortical destruction. Primary tumors in the axial skeleton may be purely osteolytic, purely osteoproductive, or a combination of both. They most often are reported in the skull, followed by the ribs, pelvis, and then the vertebrae. Osteosarcoma is the most common malignant bone tumor in dogs and cats. Most osteosarcomas arise as a solitary lesion, typically in the metaphyseal region of a long bone; rarely are they diaphyseal or epiphyseal. Most osteosarcomas present with an aggressive pattern of osteolysis, an irregular and ill‐defined periosteal response, and variable degrees of soft tissue swelling (Figure 5.22). In some cases, osteolysis is the only radiographic finding; the periosteal response may be amorphous or not evident in the images. The aggressive nature of osteosarcoma leads to a wide and indistinct zone of transition between abnormal and normal bone. Cortical destruction is a frequent finding and Codman’s triangle often is present. Osteosarcoma may be associated with bone infarcts. A classic “sunburst” pattern occurs in approximately one‐third of osteosarcomas. Osteosarcoma most often is reported in older, large, and giant breed dogs. Common site predilections are “away from the elbow” and “toward the knee,” which includes the proximal humerus, the distal radius and ulna, the distal femur, and the proximal tibia. Clinical signs of pain and lameness may be acute or their onset may be gradual and progressive. Pathologic fractures are common (Figure 5.42). Figure 5.22 Mixed pattern of osteolysis and periosteal response. Lateral view of a dog shoulder depicting an active and aggressive bone disease in the humerus. Periosteal new bone production is amorphous and ill‐defined (black arrows). There is a mixed pattern of osteolysis, including permeative, moth‐eaten, and geographic areas of bone loss (white arrow) and extensive cortical destruction. This is a very aggressive disease process, most likely a malignant bone tumor or possibly severe osteomyelitis. Figure 5.23 Osteoporosis and osteosclerosis. VD views of a dog proximal femur illustrating the appearance of (A) osteoporotic bone, (B) normal bone, and (C) osteosclerotic bone. Osteoporotic bone (A) is less opaque than normal with thin trabeculae, larger intertrabecular spaces, thin cortices, and less bone‐to‐soft tissue contrast. In osteosclerotic bone (C), the trabeculae are thicker, the intertrabecular spaces are smaller, the cortices are thickened, and the medullary cavity is smaller. Figure 5.24 Osteopetrosis. Lateral view (A) and VD view (B) of a cat thorax depicting increased opacity and thickened cortices in the axial and appendicular skeleton (e.g., vertebrae, sternebrae, ribs, humeri). Figure 5.25 Growth arrest lines. Lateral view of a dog stifle depicting several thin, well‐defined, transverse lines of osteosclerosis in the femur (arrow). Other names include stress lines and growth retardation lines. Figure 5.26 Bone infarcts. Lateral view of a dog crus depicting multiple, well‐defined foci of mineral opacity in the medullary cavity of the tibia (arrow). The radiograph was made to evaluate a cranial cruciate ligament injury (note the cranial displacement of the tibia in relation to the distal femur) and the infarcts were an incidental finding. Figure 5.27 Osteopenic bones. Lateral view of a dog stifle depicting disuse osteoporosis. The bone opacity is diminished, the cortices are thin, there is less bone‐to‐soft tissue contrast, and there is a coarse trabecular pattern. A double cortical line is visible along the cranial border of the femur due to intracortical resorption of bone (arrows). Figure 5.28 Greenstick fracture. Lateral view of a dog proximal humerus depicting an incomplete fracture on the convex side of the bone. Figure 5.29 Torus fracture. Lateral view of a dog proximal humerus depicting an incomplete fracture on the concave side of the bone. Figure 5.30 Simple c omplete fractures. Lateral views of a dog femur illustrating mid‐diaphyseal fractures that are transverse (A), short oblique (B), and long oblique (C). The soft tissues are swollen. Figure 5.31 Comminuted fracture. A. Lateral view of a dog femur depicting a mid‐diaphyseal fracture with multiple fragments. B. Enlarged image of the fracture in radiograph A highlighting a butterfly fragment (white arrow) and fissure fractures in the larger fragments (black arrows). Figure 5.32 Spiral and segmental fractures. Lateral view (A) and craniocaudal view (B) of a dog crus depicting a spiral oblique fracture in the distal tibial diaphysis (black arrow) and a segmental fracture in the proximal fibular diaphysis (white arrow). In the lateral view, the tibial fracture line is more opaque than the adjacent bone due to summation of the ends of the fragments. Displacement of the fragments is not evident in the lateral view and the fracture may be missed without the orthogonal view. Figure 5.33 Open fracture. Lateral view (A) and craniocaudal view (B) of an immature cat pelvic limb depicting an open transverse fracture in the right femoral distal physis with caudal and medial displacement and override of the fracture fragments. There is moderate soft tissue swelling at the fracture site and gas in the soft tissues (arrows). Figure 5.34 Condylar fractures. Craniocaudal views of dog stifles depicting a medial condylar fracture in the femur (A) and a “T” or “Y” fracture (B) that is both supracondylar and intercondylar. Figure 5.35 Avulsion fractures. A. Lateral view of a dog shoulder depicting an avulsion fracture in the scapular tuberosity. B. Lateral view of a dog shoulder depicting an avulsion fracture in the humeral greater tubercle with adjacent soft tissue swelling. C. Caudocranial view of a dog shoulder depicting an avulsion fracture in the spine of the scapula with adjacent soft tissue swelling. Figure 5.36 Delayed union fracture. VD view of a cat pelvis depicting a delayed union of a left femoral diaphyseal fracture. Callus is visible but less than expected. The fracture line remains distinct. Figure 5.37 Nonunion fracture. VD view of a cat pelvis depicting a nonunion fracture in the left femoral diaphyseal region. A build‐up of smooth, well‐defined new bone is visible at each fragment end, but the new bone does not bridge the fracture gap. Fracture healing has ceased, bony margins are well‐defined. Figure 5.38 Malunion fracture. VD view of a cat pelvis depicting a malunion fracture in the left femoral diaphysis. The femur is healed, but it is abnormally angulated. Figure 5.39 Bone sequestrum. Craniocaudal view of a long bone depicting a sharply marginated, sclerotic fragment of bone (white arrow) surrounded by sclerotic bone called an involucrum (black arrow). The sequestrum is surrounded by soft tissue opacity necrotic tissue. The periosteal new bone is well‐defined (yellow arrows) consistent with a chronic and not very active lesion. Figure 5.40 Bacterial osteomyelitis. Lateral views of the same dog antebrachium depicting progression of osteomyelitis. A. Normal radius and ulna. B. Soft tissue swelling is visible at the distal ulna (arrow). C. A periosteal response has developed at the distal ulnar metaphysis (arrow). D. The periosteal new bone has extended along the ulnar diaphysis (arrows). Figure 5.41 Osteomyelitis. Dorsoplantar views of a dog’s right and left tarsus. There is soft tissue swelling in the right tarsus and a periosteal response along the lateral margins of the right distal calcaneus, the fourth tarsal, and the proximal fifth metatarsal bones (arrows). The left tarsus is normal (the left tarsal radiograph is oriented to match the right for ease of comparison). Figure 5.42 Pathologic fracture. Lateral view of a dog humerus depicting a diaphyseal fracture (white arrow) at the proximal aspect of an area of geographic osteolysis (black arrow). A periosteal response is present, sooner than expected for fracture repair, indicating that a disease process was present before the fracture. Figure 5.43 Metastatic osseous neoplasia. Lateral view of a dog stifle depicting multiple focal areas of osteolysis in the femur and tibia due to multiple myeloma. Figure 5.44 Bone cyst. Lateral view of a dog shoulder depicting a well‐defined area of geographic osteolysis with a septated center in the proximal humerus (arrow). The adjacent cortex is thin and slightly expanded outward. Figure 5.45 Enchondroma. Lateral view of a dog stifle depicting a well‐defined, expansile area of soft tissue opacity in the proximal fibula (arrow). No periosteal response nor soft tissue swelling is evident. Figure 5.46 Osteochondroma. Lateral view (A) and caudocranial view (B) of a dog femur depicting a spur‐shaped growth on the caudal border of the right femur (arrow). Figure 5.47 Synovial osteochondromatosis. Lateral view of a cat stifle depicting mineral opacity structures in the femorotibial joint (arrows). Figure 5.48 Ectrodactyly. DP views of a dog right manus (A) and left manus (B) depicting absence of the left third metacarpal bone and its associated phalanges. Figure 5.49 Ectrodactyly. Dorsoplantar view of a dog pes depicting absence of a metatarsal bone. The extra digit at the fifth metatarsal bone makes the ectrodactyly less evident at first glance. Figure 5.50 Hemimelia. Craniocaudal oblique view of a dog antebrachium depicting absence of the radius. The ulna is larger than normal and the carpus is luxated. Figure 5.51 Polydactyly. Dorsopalmar view of a cat right and left manus depicting partial development of extra digits in the medial aspect of each foot (arrows). Figure 5.52 Epiphyseal dysplasia. Lateral views of a puppy thoracic limb (A), pelvic limb (B), and lumbar spine (C) depicting delayed epiphyseal ossification. The visible epiphyses appear mottled and irregular. The distal ulnar and femoral epiphyses should be visible, but are not. Figure 5.53 Rickets. Dorsopalmar oblique view of an immature cat thoracic limbs depicting widened physes with flared, concave metaphyses (arrows). Figure 5.54 Asynchronous growth. A. Lateral view of an immature dog’s left antebrachium. The limb is normal. The articular margin of the ulnar trochlear notch is continuous with the radial head, forming a smooth articulation with the humeral head (black arrow). B. Lateral view of the same dog’s right antebrachium. There is a disproportionate rate of growth between the radius and the ulna. The distal ulnar physis is closed, which stops further growth. The radial physis is still open and the radius continues to lengthen. The abnormally shortened ulna leads to cranial bowing of the radius (white arrow) and humeroulnar subluxation (black arrow). Notice the “stair step” between the proximal radius and ulna compared to the same area in the normal left limb. C. Postoperative radiograph made following a distal ulnar ostectomy. The humeroulnar luxation is reduced. Figure 5.55 Asynchronous growth. A. Craniocaudal view of the left antebrachium of the same dog as in Figure 5.54. The limb is normal. The ulnar medial coronoid process is even with the radial head, forming a continuous articulation with the humeral condyle (black arrow). B. Craniocaudal view of the same dog right antebrachium. Premature closure of the distal ulnar physis results in an abnormally shortened ulna. Continued growth at the radial physis leads to lateral bowing of the radius (white arrow), lateral deviation of the carpus (yellow arrow), and humeroulnar subluxation (black arrow). Again, notice the “stair step” between the proximal radius and ulna compared to the same area in the normal left limb. C. Postoperative view made after a distal ulnar ostectomy. The humeroulnar luxation is reduced and the carpus is less deviated. Figure 5.57 Asynchronous growth. Lateral view (A) and craniocaudal view (B) of a dog antebrachium with severe disproportionate growth of the radius and ulna. Depicted in these radiographs is an abnormally shortened ulna that lead to radius curvus, carpal valgus, lateral luxation of the radial head (arrow indicates direction of displacement), and humeroulnar subluxation. Figure 5.58 Asynchronous growth. Lateral view (A) and craniocaudal view (B) of a dog antebrachium depicting an abnormally shortened radius that resulted in widening of the humeroradial and radiocarpal joint spaces. The distal radial physis is closed; no further growth will occur here. The ulnar physis is still open so the ulna continues to lengthen normally, leading to the humeroradial and radiocarpal subluxations. Figure 5.59 Asynchronous growth. Craniocaudal views of an immature dog right antebrachium (A) and left antebrachium (B) depicting abnormal growth in the right distal radius due to partial damage of the physis. There is less or slower growth at the lateral aspect of the damaged physis than at the medial aspect. Continued growth at the medial aspect leads to carpal valgus and humeroradial subluxation. The left limb is normal. Figure 5.56 Elbow subluxation. A. Illustration showing the normal alignment along the radioulnar articular border. B. Subluxation caused by asynchronous growth with an abnormally shortened ulna. There is a misalignment or “stair‐step” along the radioulnar border with the ulnar medial coronoid process distal to the radial head. C. Subluxation caused by asynchronous growth with an abnormally shortened radius There is a “stair‐step” because the medial coronoid is proximal to the radial head. Figure 5.60 Asynchronous growth due to synostosis. A. Lateral view of a dog antebrachium depicting a diaphyseal fusion between the radius and ulna (black arrow). The fusion restricted lengthening of the ulna and resulted in elbow subluxation (yellow arrow). B. Craniocaudal view of the same dog elbow depicting the elbow subluxation (arrow). Figure 5.61 Hypertrophic osteodystrophy. A. Craniocaudal view of a dog distal antebrachium depicting lines of decreased opacity in the metaphysis, parallel to the physes (arrows). This is sometimes called a “double physis” sign. B. Lateral view of the same dog 2 days later with wider soft tissue opacity lines (arrows), more soft tissue swelling, and a minimal amount of mineralized subperiosteal hemorrhage. C. Lateral view of the distal crus (same dog a few days later) depicting mineralization of subperiosteal hemorrhage (arrows) and soft tissue swelling. The “double physis” sign is less distinct, but still present. D. Lateral view of the same dog later in the disease depicting larger cuffs of new bone around the metaphysis (arrows). The “double physis” sign is no longer evident (typically it only lasts a couple of weeks). E. Lateral view of a dog distal antebrachium depicting periosteal new bone filling the space between the elevated periosteum and the cortex (arrow). The new bone is smooth, well‐defined, and beginning to organize and blend with the mineralized subperiosteal hemorrhage. F. This image depicts superimposition of a pathology specimen on a radiograph of the distal antebrachium to illustrate the appearance of hemorrhage near the physis (white arrows) and under the periosteum (yellow arrows). Figure 5.62 Hypertrophic osteodystrophy. Lateral views of a dog forelimb (A) and hindlimb (B) depicting the distibution of HOD lesions at the ends of the long bones. Notice the increased opacity in the metaphyseal regions due to summation of periosteal new bone. Figure 5.63 Hypertrophic osteopathy. Dorsopalmar view of a dog carpus (A) and dorsoplantar view of the same dog tarsus (B) depicting an irregular, well‐defined, periosteal response along the distal ulna, the metacarpal and metatarsal bones, and the phalanges (arrows). There is diffuse, mild soft tissue swelling. Notice the medullary regions of the long bone diaphyses appear increased in opacity due to summation of periosteal new bone (may be mistaken for panosteitis). Figure 5.64 Chronic hypertrophic osteopathy. Lateral view of a dog femur (A) and tarsus (B) depicting a smooth‐bordered, well‐defined, periosteal response along the cranial border of the femur and the dorsal and plantar borders of the metatarsal bones (arrows). Figure 5.65 Retained cartilage core. Lateral view (A) and craniocaudal view (B) of an immature dog antebrachium depicting a cone‐shaped soft tissue opacity area in the distal ulnar metaphysis, extending into the diaphysis (arrows). The physes and epiphyses are normal. Figure 5.66 Panosteitis. Lateral views of a dog elbow (A) and stifle (B) depicting increased medullary opacity in the distal humerus, proximal ulna, femur, and proximal tibia (arrows). Figure 5.67 Villonodular synovitis. Caudocranial view of a dog stifle depicting a focal area of decreased opacity at the edge of the proximal tibia (arrow) caused by pressure remodeling due to synovial thickening. Figure 5.68 Osteophytes and enthesophytes. Illustration of a synovial joint with DJD. Osteophytes form at the periphery of the articular cartilage, in the non‐weight‐bearing areas. Enthesophytes form at attachment sites (in this case, at the joint capsule attachments). Compare this illustration with the normal synovial joint in Table 5.6. Figure 5.69 Osteophytes. Photograph of a dog humeral head specimen depicting periarticular osteophytes (arrows). Figure 5.70 Erosive arthritis. Lateral view (A), dorsopalmar views (B and C) of a cat manus depicting extensive subchondral osteolysis in the metacarpophalangeal and interphalangeal joints with joint swelling, collapse of the joint spaces, and weakening of the supportive ligaments. Figure 5.71 Synovial cell sarcoma. Lateral view (A) and caudocranial view (B) of a dog stifle depicting geographic and permeative osteolysis in the distal femur and proximal tibia. There is a concave defect along the cranial cortex of the distal femur due to pressure remodeling caused by the tumor (arrow). Figure 5.72 Osteochondrosis. Lateral view of a dog shoulder depicting a flattened, osteosclerotic area in the caudal aspect of the humeral head (arrow). Figure 5.73 Normal shoulder. Lateral view of a dog scapulohumeral joint depicting normal anatomy. Figure 5.74 Normal shoulder. Craniocaudal view of a dog scapulohumeral joint depicting normal anatomy. Figure 5.75 Normal shoulder. Skyline view of a dog humerus depicting normal anatomy. Figure 5.76 Normal shoulder. Lateral view of a dog scapulohumeral joint depicting the attachment sites for the joint capsule and the intra‐articular ligaments, the latter including the biceps tendon and the medial and lateral glenohumeral ligaments. Figure 5.77 Normal shoulder. Lateral view of a dog scapulohumeral joint depicting the attachment sites for muscles and their tendons. Figure 5.78 Normal immature canine shoulder. Lateral view depicting a puppy scapulohumeral joint. Figure 5.79 Normal clavicle. Cat: lateral view (A) and caudocranial view (B) of a cat shoulder depicting the relatively large and curvilinear feline clavicles (arrows). Dog: lateral view (C) and caudocranial view (D) of a dog shoulder depicting the relatively small and incompletely mineralized canine clavicle (arrows). Figure 5.80 Accessory caudal glenoid ossification center. Lateral view of a dog shoulder depicting a focal mineral opacity at the caudal margin of scapular glenoid (arrow). Figure 5.81 Shoulder DJD. Lateral view (A) and skyline view (B) of a dog shoulder depicting osteophytes on the caudal articular margins of the humeral head and scapular glenoid and along the bicipital groove (arrows). Figure 5.82 Scapulohumeral luxation. Caudocranial view of the shoulders of a dog depicting chronic luxation of the left scapulohumeral joint with remodeling of the humeral head and distal scapula (arrow). The left scapulohumeral joint is normal. Figure 5.83 Osteochondrosis. Lateral view of an immature dog shoulder depicting flattening of the caudal humeral head with subchondral osteosclerosis and a thin flap of mineralized cartilage (yellow arrow). A mineralized fragment is visible in the caudal joint pouch (white arrow). The deep circumflex humeral artery is seen end‐on (red arrow), visible because it is surrounded by fat. Figure 5.84 Mineralized intra‐articular structures. Lateral view (A) and caudocranial view (B) of a dog shoulder depicting well‐defined, mineralized fragments in the caudal joint pouch (arrows). Figure 5.85 Shoulder mineralization. Lateral view of a dog scapulohumeral joint depicting foci of mineralization in the area of the greater tubercle (arrow). Mineralization in this area may represent osteophytes along the bicipital groove or it may be associated with the supraspinatus, infraspinatus, or biceps muscle tendon or joint capsule. Occasionally, sesamoid bones are present in this area. Note: mineralization occurs over time and may or may not be associated with current lameness. Figure 5.86 Supraspinatus tendinopathy. Lateral view of a dog shoulder depicting mineralization along the cranial border of the greater tubercle (arrow), in the area of insertion of the supraspinatus muscle tendon. Figure 5.87 Normal elbow. Lateral view of a dog elbow depicting normal anatomy. Figure 5.88 Normal elbow. Flexed lateral view of a dog elbow depicting normal anatomy. The trochlear notch of the ulna is also called the semilunar notch. Figure 5.89 Normal elbow. Craniocaudal view of a dog elbow depicting normal anatomy. Figure 5.90 Supracondylar foramen. Craniocaudal oblique view of a cat elbow depicting the normal foramen on the lateral aspect of the distal humerus (arrow). Figure 5.91 Normal elbow. Lateral view of a dog elbow depicting the attachment sites for ligaments and the joint capsule. The black arrow points to enthesopathy at the attachment of the interosseous ligament. This is a common and usually asymptomatic finding. There is no osteolysis and the amount of interosseous mineralization can vary considerably between individuals. The white dotted oval indicates the normally less opaque part of the humeral medullary cavity, located distal to the nutrient foramen (white arrow). This part of the distal humerus is a common area for panosteitis lesions. If this area is not relatively less opaque, consider panosteitis as the cause. Figure 5.92 Normal elbow. Lateral view of a dog elbow depicting the attachment sites for muscles and their tendons. Figure 5.93 Normal elbow. Craniocaudal view of a dog elbow depicting attachment sites for the collateral ligaments and the origins of the flexor and extensor muscle tendons. Figure 5.94 Normal immature canine elbow. Lateral view (A) and craniocaudal view (B) depicting a puppy elbow. Figure 5.95 Chondrodystrophic forelimb. Lateral view of the thoracic limb of an adult Corgi with no evidence of lameness. Figure 5.96 Elbow DJD. Lateral view (A), flexed lateral view (B), and craniocaudal view (C) of a dog elbow depicting osteophytes on the radial head (R), medial coronoid process (MCP), along the ulnar trochlear notch (U), and at the anconeal process (AP) as indicated by the yellow arrows. The black arrows point to enthesophytes on the medial epicondyle (ME). The bone spurs on the medial epicondyle and anconeal process are not well visualized in the lateral view (A) due to summation. The lesions are readily identified in the flexed lateral view (B). Figure 5.97 Elbow enthesophyte. Lateral view of a dog elbow depicting a bone spur on the medial epicondyle (arrow). Figure 5.98 Fragmented medial coronoid process. A. Craniocaudal view of a dog elbow depicting the normal appearance of the medial coronoid process (arrow). B. Craniocaudal view of a different dog elbow depicting a blunted, rounded medial coronoid process (arrow). The process is not well visualized due to superimposition of the ulna. C. Craniocaudal oblique view of the same dog as in (B). Rotation of the elbow eliminates superimposition of the ulna to improve visualization of the blunted medial coronoid process (yellow arrow) and reveals a focal area of osteolysis in the distal humerus (a “kissing lesion”, black arrow). Figure 5.99 Ulnar articular surfaces. A. Flexed lateral view of a dog elbow illustrating the medial coronoid process (MCP), anconeal process (AP), and olecranon (O). B. Same specimen as A, but the ulna is highlighted and the other bones faded to show the articular surfaces of the ulnar coronoid process (medial and lateral projections) and trochlear notch. C. An actual radiograph with the same ulnar articular surfaces highlighted (arrows). Figure 5.100 Ulnar trochlear notch. Photographs of two different canine bone specimens that demonstrate a normal variation in the appearance of the ulnar trochlear notch (arrows). The articular edges in A extend further proximally than in B. Figure 5.101 Ulnar trochlear notch. A. Photograph of the same ulna as in Figure 5.100A, but from a different angle. The articular border of the trochlear notch is visible above (proximal to) the anconeal process (arrow). B. Lateral radiograph of an ulna in which the articular border of the trochlear notch projects proximal to the anconeal process (arrow). C. Lateral radiograph of a different ulna in which the articular border of the trochlea is not visible proximal to the anconeal process. Figure 5.102 Ulnar degenerative changes. A. Photograph of an ulna bone specimen with osteophytes along the trochlear notch and anconeal process (arrows). B. Lateral radiograph of the ulna bone specimen (arrows point to osteophytes). Compare with the normal ulna bone specimen and radiographs in Figure 5.100. Figure 5.103 Elbow “bump”. Flexed lateral view of a dog elbow depicting a smooth, well‐defined bony margin extending proximal to the anconeal process (arrow). The dog is asymptomatic and there are no other signs of degenerative joint disease, no soft tissue swelling, or other signs of an elbow abnormality in this radiograph. The elbow, including the medial coronoid process, was normal in the orthogonal view, suggesting that this elbow “bump” likely is a normal anatomic variation caused by the articular border of the ulnar trochlear notch. Figure 5.104 Ununited anconeal process. Lateral view of a dog elbow depicting a line of soft tissue opacity at the base of the anconeal process (arrow). Figure 5.105 Normal elbow. A. Lateral view of an immature dog elbow depicting superimposition of the physis of the medial humeral epicondyle (arrow) on the anconeal process. Superimposition of the physis may be mistaken for an ununited anconeal process. B. Flexed lateral view of the same dog, eliminating superimposition of the physis (yellow arrow) and revealing a normal anconeal process (black arrow). The physis of the medial epicondyle generally remains visible until about 6 months of age. Figure 5.106 Elbow incongruity. Lateral view of a dog elbow (A) and an illustration of a dog elbow (B), both depicting abnormal fit between the humeral condyle and the ulnar trochlear notch. The trochlear notch is too small (arrow). Figure 5.107 Osteochondrosis. Craniocaudal oblique view of a dog elbow depicting a focal area of decreased opacity on the medial articular margin of the humeral condyle (arrow). This subchondral defect is consistent with an OCD lesion, however, a similar finding may be seen due to focal osteolysis adjacent to a fragmented medial coronoid process (i.e., “kissing lesion”). Figure 5.108 Ununited medial humeral epicondyle. Craniocaudal view of a dog elbow depicting a mineral opacity structure in the soft tissues medial to the humeral medial epicondyle (yellow arrow). There is no soft tissue swelling or evidence of active bone remodeling. The margin of the medial epicondyle is slightly irregular, but well defined. An osteophyte is visible on the medial coronoid process (black arrow). A normal sesamoid bone is present near the radial head (red arrow). Figure 5.109 Incomplete ossification of humeral condyle. A. Craniocaudal (CrCa) view of an immature dog depicting the normal medial and lateral ossification centers (M and L) that fuse to form the humeral condyle. B. CrCa view of a different immature dog depicting a soft tissue opacity line in the humeral condyle (arrow) due to incomplete mineralization of the cartilage between the ossification centers. C. CrCa view of a dog elbow depicting a laterally displaced lateral humeral condylar fracture. D. CrCa view of a normal dog elbow depicting an artifact in the humeral condyle caused by summation of the ulna (arrow). This artifact may be mistaken for incomplete ossification of the humeral condyle. Figure 5.110 Elbow luxation. Craniocaudal view (A) and lateral view (B) of a dog elbow depicting a lateral luxation of the humeroantebrachial joint. The luxation could easily be missed in the lateral view alone. Figure 5.111 Elbow subluxation. A. Craniocaudal view of a dog antebrachium depicting very subtle, slight widening of the humeroulnar joint space (black arrow) and a minimally displaced oblique fracture in the distal ulna (yellow arrow). B. Stress view crca view of the same dog depicting more severe widening of the joint (black arrow) due to application of a lateral‐to‐medial force at the elbow, documenting abnormal elbow joint laxity. Notice that the distal ulnar fracture is also widened in this view. Figure 5.112 RUIN. Lateral view (A) and craniocaudal view (B) of a dog antebrachium depicting focal osteolysis and a periosteal response in the interosseous space between the radius and ulna (arrows). Figure 5.113 Normal carpus. Lateral view of a dog carpus depicting normal anatomy. The fifth metacarpal bone (MC5) is identified because it is positioned immediately distal to the ulnar carpal bone. The other metacarpal bones, as well as the distal row of carpal bones, are summated in this view and not individually recognized. Figure 5.114 Normal carpus. Dorsopalmar view of a dog carpus depicting normal anatomy. The accessory carpal bone is superimposed on the distal radius The distal row of carpal bones are numbered 1–4 and the metacarpal bones are numbered I–V. A fifth carpal bone may be present in some dogs. Figure 5.115 Carpal luxation. Lateral view (A) and dorsopalmar view (B) of a dog carpus depicting a medial luxation of the antebrachiocarpal joint (arrows). Bandaging material is visible peripheral to the skin in the lateral view. Figure 5.116 Stenosing tenosynovitis. Dorsopalmar view of a dog carpus depicting localized soft tissue swelling along the medial carpus and an enthesophyte on the medial aspect of the distal radius (arrow). Figure 5.117 Normal foot. Lateral view of a dog manus depicting normal anatomy. The digits are separated by using gentle compression applied with a plastic paddle. The first digit or “dewclaw” is shorter than the others and includes the first metacarpal bone (MC1), a proximal phalanx (P1), and a distal phalanx (P3). The second digit is identified because it is adjacent to the first and includes MC2, a proximal phalanx (P1), a middle phalanx (P2), and a distal phalanx (P3). The dorsal border of the fifth metacarpal bone (MC5) is identified because digit 5 is shorter than digits 3 and 4 (digit 2 also is shorter than digits 3 and 4). Figure 5.118 Normal foot. Dorsopalmar view of a dog manus depicting normal anatomy. The medial carpal sesamoid bone is located in the tendon of the abductor pollicis longus muscle. Digits 1 through 5 are labeled D1‐D5. The palmar sesamoid bones are numbered from medial to lateral 1‐8. The metacarpal foot pad is superimposed on the digits. Figure 5.119 Normal immature canine manus. Lateral view (A), lateral oblique view (B), and dorsopalmar view (C) of a puppy carpus and digits. The yellow arrows point to bunching of the skin caused by wrapping a tie around the limb to aid in positioning. The black arrow points to the cut back zone in the radial metaphysis. Figure 5.120 Normal immature feline forelimb. Lateral view (A) and craniocaudal view (B) depicting a kitten thoracic limb. The arrow points to the supracarpal foot pad which appears as a soft tissue opacity nodule in the caudomedial aspect of the distal antebrachium. It sometimes is mistaken for a lesion. Notice that in cats, the distal ulna is relatively larger than in dogs and the distal ulnar physis is transverse as opposed to conical in shape. Figure 5.121 Digital neoplasia. Dorsopalmar view of a dog foot depicting soft tissue swelling and osteolysis associated with the distal phalanx in the second digit (arrow). There is no visible periosteal response and no evidence that the lesion involves the joint or any other bone. Figure 5.122 Digital lesion. Lateral view (A) and dorsopalmar view (B) of a dog manus depicting an aggressive, expansile, osteolytic lytic lesion in the proximal phalanx of the second digit. The final diagnosis was osteosarcoma. Figure 5.123 Normal pelvis. VD view of a dog pelvis depicting normal anatomy and superimposition artifacts; the latter includes the prepuce, tail folds, and inguinal skin folds. Figure 5.124 Normal pelvis. VD view of a dog pelvis depicting normal anatomy (same radiograph as Figure 5.123, but additional structures are labeled). Notice the triangle shaped bone structure at the caudal aspect of the pubic symphysis (it should not be mistaken for a fracture). Figure 5.125 Normal coxofemoral joint. Close‐up VD view of a dog pelvis depicting normal anatomy. The “joint space” arrow points to the cranial third of the acetabulum, which is the part of the joint space that should be evaluated for evidence of subluxation. Don’t evaluate the middle third because of the fovea capitis and don’t evaluate the caudal third because of the acetabular notch. Note: the acetabular notch is a non‐weight bearing part of the coxofemoral joint and therefore a common site of osteophyte formation in cases of DJD. Figure 5.126 Normal feline pelvis. VD view of a cat pelvis. Compared to dogs, the feline pelvis is more rectangular in shape with relatively longer ischii. Figure 5.127 Normal pelvis. Lateral view of a dog pelvis depicting normal anatomy. The radiograph is slightly obliqued to eliminate superimposition of the hemipelves and coxofemoral joints. The iliac crests may never completely fuse to the ilial wings. Figure 5.128 Normal immature canine pelvis. Lateral view (A), illustrated lateral view (B) and VD view (C) depicting a normal puppy pelvis. The triangular‐shaped acetabular bone normally fuses with the ilium and ischium by about 8 weeks of age. In male dogs, the prepuce frequently is seen superimposed over the caudal abdomen in a VD/DV view and may be mistaken for a lesion. Figure 5.129 Pelvic accessory ossification centers. VD view of a dog pelvis depicting (1) mineralization in the gluteal muscle tendon; (2) an ossicle on the cranial acetabular rim; (3) sesamoid bone in the psoas minor muscle tendon, located near the iliopectineal eminence; (4) sesamoid bone in the iliopsoas muscle tendon. Figure 5.130 Avascular necrosis of femoral head. VD view of a dog pelvis depicting a misshapen right femoral head (arrow). The femoral head is diminished in opacity and there is adjacent osteosclerosis in the femoral neck. Figure 5.131 Hip DJD. VD view of a dog pelvis depicting bilateral osteophyte formation on the acetabular rims (yellow arrows point to osteophytes on the cranial, dorsal, and caudal aspects of the right acetabular rim), along the femoral necks (red arrow), and at the acetabular notch (blue arrow). Enthesophytes are present at the attachment sites of the joint capsule (black arrow). Figure 5.132 Femoral head and neck “lines”. VD view of a dog pelvis depicting mineral opacity lines that often are seen on the proximal femur. The physeal scar is intra‐articular. Osteophytes form at the edges of the articular cartilage. Enthesophytes form at the attachment of the joint capsule. Figure 5.133 Mach line. A. VD view of a dog pelvis depicting a line of increased opacity along the femoral neck (arrow). This line is an artifact caused by the Mach phenomenon (Figure A38 in Chapter 2, Artifacts). B. In this image, a pathology specimen is superimposed on the radiograph to illustrate the tight “undercut” curve at the junction of the femoral head and neck (arrow). This curve is responsible for the positive Mach line. Notice that the Mach line does not extend outside or beyond the femoral neck, whereas osteophytes often do. Sometimes making additional views with the limbs in slightly different positions helps make the distinction. Figure 5.134 Extended VD view of the pelvis. VD view of a dog pelvis depicting the proper patient positioning for evaluation of hip dysplasia. Figure 5.135 Coxofemoral joint “fit”. This illustration depicts a normal coxofemoral joint on the left and a “loose” joint on the right. On the left, the cranial joint space is even (shaded area). On the right, the cranial joint space is wedged because the femoral head is subluxated (the head has moved away from the acetabular fossa). The concave area on the femoral head represents the normal fovea capitus. Figure 5.136 Distraction. A. VD view of a dog pelvis depicting a normal appearing coxofemoral joint. B. VD view of the same dog pelvis with distraction. The femoral head is displaced laterally (subluxated) indicating joint laxity. Figure 5.137 Half axial views. A. VD extended view of a dog pelvis with normal appearing coxofemoral joints. B. VD half‐axial view of the same dog, but both hips are now subluxated, as indicated by the cranial wedging of the coxofemoral joints. Lateral distraction of the femurs reveals th hip laxity in this dog. C. VD half‐axial view of a different dog pelvis for comparison. This dog has no evidence of hip laxity; the femoral heads remain well‐seated in the acetabular fossae during distraction. D. An illustration of the distraction device that was placed between the patient’s thighs to make the half‐axial views. This device acts as a fulcrum to distract the hips when the tarsi are moved together (see also Figure 2.37). Figure 5.138 Rotated pelvis. VD view (A) and lateral view (B) of a dog pelvis. In the VD view, the left ilial wing appears wider than the right ilial wing (red double‐headed arrows). Also in the VD view, the left obturator foramen appears smaller than the right obturator foramen. These findings indicate that the pelvis is slightly rotated. Pelvic rotation alters the apparent coverage of the femoral heads by the dorsal acetabular rims (black arrows). Notice that there appears to be less coverage of the left femoral head than the right. This is a positioning artifact that may be mistaken for a shallow left acetabular fossa. The acetabulum on the side with the wider ilial wing may appear abnormally shallow (memory tip: “wider = worse”) and the acetabulum on the side with the bigger obturator foramen may appear deeper than actual size (memory tip: “bigger = better”). Notice in the VD view that there is a well‐defined area of less opacity in the left ischium (yellow arrow). This is an artifact caused by superimposition of anal gland gas, as can be seen in the lateral view (arrow). Figure 5.139 “Flattening” of the femoral heads. VD view of a dog pelvis depicting bilateral hip dysplasia with coxofemoral joint subluxation and advanced degenerative joint disease. The apparent “flattening” of the femoral heads, particularly in the left hip, is due to the extensive buildup of new bone on the femoral necks (arrows). Figure 5.140 Angle of femoral neck. These photographs of the same bone specimen illustrate the different appearances of the femur depending on the point of view. Notice that the angle between the femoral neck and the femoral shaft (dotted lines) appears to vary as the femur is rotated. On the left, the angle appears increased (coxa valga); in the middle the angle is normal (the femur is viewed en face); and on the right, the angle appears decreased (coxa vara). Figure 5.141 Grades of hip dysplasia. A. VD view of a dog pelvis depicting normal hip joint conformation. Notice the amount of coverage of the femoral head by the dorsal acetabular rim (white arrow) and the uniformly even coxofemoral joint space (black arrow). Remember to evaluate the cranial part of the joint space. B. VD view depicting mild hip dysplasia with slight cranial wedging of the coxofemoral joint space and less than 50% coverage of the femoral head by the dorsal acetabular rim. C. VD view depicting moderate hip dysplasia with more severe subluxation, less coverage of the femoral head, and osteophytes on the femoral neck. D. VD view depicting severe hip dysplasia with subluxation, a flattened acetabular fossa, and a large amount of osteophytosis. Figure 5.142 PennHIP. VD view of a dog made during distraction and illustrating the measurements used to calculate the Distraction Index (DI). DI is the distance (D) between the femoral head center (F) and the acetabular center (A) divided by the radius of the femoral head (R). Figure 5.143 Norberg angle. VD view of a dog pelvis illustrating the Norberg measurement technique. The Norberg angle is a numerical assessment of hip joint laxity. To make the measurement, you first locate the center of each femoral head (black dots). Second, draw a line between the centers (yellow line). Third, draw a line from each center to the cranial acetabular rim (white lines). The intersection of a white line with the yellow line determines the Norberg angle for that hip. An angle greater than 105° is considered normal, an angle between 90° and 105° is borderline abnormal, and an angle less than 90° is abnormal. Figure 5.144 Apple‐core lesion. Flexed VD view of a dog pelvis depicting a narrowed left femoral neck with an irregular, indistinct margin (arrow). Figure 5.145 Feline capital physeal dysplasia syndrome. VD view of a cat pelvis depicting a displaced fracture in the right femoral proximal physis (arrow). Figure 5.146 Coxofemoral luxation. A. Lateral view of a dog pelvis depicting dorsal displacement of the left femur (arrow). B. VD view of the same dog; the coxofemoral luxation is not as evident because the femoral head is superimposed on the acetabular fossa. The arrow points to a small chip fracture just caudal to the left femoral head. C. VD view of a different dog pelvis depicting cranial luxation of the left hip. Figure 5.147 Sacroiliac luxation. A. VD view of a normal dog pelvis. The arch formed by the inner borders of each ilium and the sacrum is continuous and even (arrows). B. VD view of a sacroiliac luxation. There is a discontinuity or “stair‐steps” in the arch (arrows) due to cranial displacement of the ilia. Figure 5.148 Pelvic neoplasia. VD view of a dog pelvis depicting aggressive osteolysis with an amorphous periosteal response in the left ischium (arrow). Figure 5.149 Normal stifle. Lateral view of a dog stifle depicting normal anatomy. Notice that the cranial portion of the meniscus is visible as the soft tissue opacity triangle caudal to the infrapatellar fat pad. Figure 5.150 Normal stifle. Caudocranial view of a dog stifle depicting normal anatomy. The intercondylar eminence consists of lateral and medial tubercles that project into the intercondylar fossa of the femur. The femoral trochlea is also called the patellar groove. Notice that the lateral portion of the meniscus is visible as a soft tissue opacity triangle at the lateral aspect of the femorotibial joint space. The medial portion is thin and seldom visible. Figure 5.151 Normal stifle. Skyline view of a cat stifle depicting normal anatomy. Figure 5.152 Normal stifle. Lateral view of a dog stifle illustrating the attachment sites of the joint capsule, ligaments, and tendons at the stifle. Figure 5.153 Normal stifle. Lateral view of a dog stifle illustrating the attachment sites of the cruciate ligaments. Figure 5.154 Normal immature canine stifle. Lateral view (A) and caudocranial view (B) depicting a puppy stifle. Figure 5.155 Stifle DJD. Lateral view (A) and caudocranial view (B) of a dog stifle illustrating the sites where osteophytes may form. Look for osteophytes in the peripheral areas of non‐weight bearing articular cartilage, including the femoral trochlear ridges (patellar groove) and the tibial intercondylar eminence. Figure 5.156 Stifle DJD. Lateral view of a dog stifle depicting osteophytes on the trochlear ridge of the femur, distal patella, extensor fossa, tibial plateau, and fabellae (arrows). Figure 5.157 Cruciate ligament injuries. Lateral views of dog stifles. The black dots in each radiograph designate the center of the femoral condyles and the center of each tibial intercondylar eminence. The black arrows point to the infrapatellar fat pads. The yellow arrows point to a fat opacity fascial plane. A. Normal stifle with good alignment between the proximal tibia and the distal femur, a normal fat pad, and a normal fascial plane. B. The proximal tibia is cranially displaced in relation to the distal femur due to a rupture of the cranial cruciate ligament. Joint effusion distends the femorotibial joint capsule, partially effacing the fat pad and pushing the fascial plane caudally. C. The proximal tibia is caudally displaced in relation to the distal femur due to a caudal cruciate ligament rupture. Joint effusion again effaces the fad pad, reducing its apparent size, and pushes the fascial plane caudally. Figure 5.158 Cranial cruciate ligament rupture. Lateral view of a dog stifle depicting cranial displacement of the proximal tibia in relation to the distal femur. Joint effusion causes the joint capsule to distend, partially effacing the infrapatellar fat pad and pushing the caudal fascial plane further caudally (yellow arrows). The popliteal sesamoid bone is displaced caudally and distally (black arrow). The well‐defined, smooth bordered bone along the caudal border of the distal femur is a normal attachment site for the origin of the gastrocnemius muscle tendons (red arrow). The size and appearance of this attachment site is variable between animals. Figure 5.159 Gastrocnemius tendon avulsion. Lateral view (A) and caudocranial view (B) of a cat stifle depicting caudal and distal displacement of the lateral fabella (white arrow) due to tearing at the origin of the lateral branch of the gastrocnemius muscle tendon. The smaller medial fabella is normal in position (black arrow). The focal mineral opacity structure in the femoropatellar joint is likely associated with the meniscus (yellow arrow). These “meniscal ossicles” are a frequent, asymptomatic finding in cats. The mottled background pattern in these radiographs is caused by the canvas stretcher on which the cat was lying during radiography. Figure 5.160 Avulsion of long digital extensor tendon. Lateral view of a dog stifle depicting an avulsed bone fragment that is displaced distally from the extensor fossa (arrow). There is joint effusion. Osteophytes and enthesophytes are visible, particularly on the patella and patellar groove. Figure 5.161 Avulsion fracture of tibial tuberosity. A. Lateral view of an immature dog stifle depicting proximal displacement and clockwise rotation of the tibial tuberosity fragment (yellow arrow) and moderate soft tissue swelling. B. Lateral view of a different immature dog stifle depicting less severe avulsion of the tibial tuberosity (yellow arrow) and a fracture in the proximal tibial physis, the latter resulting in caudal displacement of the proximal epiphysis (black arrow). Figure 5.162 Ruptured patellar ligament. Lateral view of a cat stifle depicting soft tissue swelling associated with the patellar ligament (arrow) and proximal displacement of the patella. Soft tissue swelling blends with the patellar ligament because they are the same opacity with no different opacity between them. Figure 5.163 Damaged proximal tibial physis. VD view of the pelvis (A), lateral view of the left pelvic limb (B), and lateral view of the right pelvic limb (C) of the same dog depicting an abnormally shortened right tibia (yellow arrow) with a bowed right fibula (black arrow). The right tibial plateau slopes caudodistally and the right fibular head is luxated. The left leg is normal. Figure 5.164 Medial patellar luxation. Craniocaudal view (A), lateral views (B and C), and skyline views (D and E) of an immature dog’s right and left stifles depicting medial luxation of the right patella (arrow in A). The right patella partially overlies the femur in the lateral view (C). The left stifle is normal. Figure 5.165 Effects of stifle positioning. These photographs of the same bone model illustrate the appearance of the distal femur and proximal tibia due to stifle rotation and different points of view. Figure 5.166 Tibial cartilage rest. A. Lateral view of a normal immature dog stifle depicting the cartilage junction between the physes in the proximal tibia and the tibial tuberosity (arrow). B. Lateral view of a normal adult dog stifle depicting a cartilage rest (arrow); a focal area of soft tissue opacity between the tibial plateau and the tibial tuberosity. Figure 5.167 Incidental findings. Lateral view of a dog tibia depicting an enthesophyte in the cranial tibial muscle tendon, extending distally from the tibial tuberosity (yellow arrow). There is no soft tissue swelling or active bone remodeling. The black arrow points to decreased opacity in the proximal tibia due to cartilage rests (cartilage that did not completely ossify during bone development). Figure 5.168 Stifle osteochondrosis. Lateral view (A) and caudocranial view (B) of an immature dog stifle depicting a flattened area on the articular border of the medial femoral condyle (yellow arrows). The rounded defect in the condyle is surrounded by osteosclerosis. The black arrows point to the normal extensor fossa which may be mistaken for a lesion. Figure 5.169 Bipartite patella. Lateral view of a cat stifle depicting two well‐defined mineral opacity structures in the area of the patella (arrow). There is no soft tissue swelling. This finding is consistent with a bipartite patella; however, a chronic, non‐union fracture of the patella may be similar in appearance. Figure 5.170 Multipartite fabella. Lateral view (A) and caudocranial view (B) of a dog stifle depicting multiple mineral opacity structures in the area of the lateral fabella (arrows). This is an incidental finding. There is, however, cranial displacement of the proximal tibia relative to the distal femur and moderate joint effusion indicating cranial cruciate ligament damage. Figure 5.171 Summation artifacts. A. Craniocaudal view of a dog tibia depicting superimposition of the Achilles tendon on the medullary region of the distal tibia (arrow). The artifact may be mistaken for a fracture line. B. Craniocaudal view of the same tibia, but slightly obliqued to eliminate superimposition of the Achilles tendon. C. Lateral view of the same tibia depicting superimposition of the fibula, which also may be mistaken for a fracture line (yellow arrow). In addition, there appears to be a line of decreased opacity where the fibula crosses the caudal cortex of the tibia (red arrow). This is an optical illusion called a negative Mach line, another artifact which may be mistaken for a fracture or a nutrient foramen. The black arrow points to the saphenous vein. Figure 5.172 Normal tarsus. Lateral view of a dog tarsus depicting normal anatomy. The fibula is superimposed on the tibia (arrow points to the caudal edge of the fibula). The appearance of the first digit is quite variable. The central tarsal bone is identified because it is located immediately distal to the talus (arrow points to the dorsal border of the central tarsal bone). Figure 5.173 Normal tarsus. Dorsoplantar view of a dog tarsus depicting normal anatomy. The base of the fifth metatarsal bone (MT5 or V) is larger laterally, which is a useful landmark to determine the lateral side of the foot. The fourth tarsal bone spans the distal intertarsal joint space. The first tarsal and metatarsal bones are quite variable in appearance and are not present in all dogs. Sesamoid bones may be present on the medial and lateral aspects of the tarsus as shown. What we are calling a lateral sesamoid bone may in some dogs be a fifth tarsal bone. In cats, both the base of MT5 and the lateral malleolus tend to be relatively larger than in dogs. Figure 5.174 Normal immature canine tarsus. Lateral view (A) and dorsoplantar view (B) depicting a puppy tarsus. Figure 5.175 Common calcanean tendon injury. Lateral view of a dog tarsus depicting soft tissue swelling in the distal Achilles tendon (black arrow) and an irregular, but well‐defined margin on the calcanean tuber (yellow arrow), the latter suggestive of a chronic condition. Figure 5.176 Tarsal trauma. Lateral view of a dog tarsus made while applying a dorsal‐to‐plantar stress at the proximal metatarsus (white dashed arrow). The radiograph depicts tarsometatarsal laxity and subluxation and tiny chip fractures at the joint. An avulsion fracture fragment is visible at the tuber calcis (yellow arrow). Figure 5.177 Degenerative joint disease in the tarsus. Lateral view (A) and DP view (B) of a dog tarsus depicting osteophytes on the distal tibia, fibula, talus, and calcaneus (arrows). There is moderate joint effusion. Figure 5.178 Fractured medial malleolus. Lateral view (A) and dorsoplantar view (B) of a dog tarsus depicting a distally displaced, short oblique fracture in the tibial medial malleolus (yellow arrow). In the DP view, the tibiotarsal joint space is widened medially due to ligamentous damage and subluxation. Figure 5.179 Tarsometatarsal luxation. Lateral oblique view (A) and dorsoplantar view (B) of a dog tarsus depiciting widening and malalignment at the tarsometatarsal joint, which is made evident by the application of a stress during radiography (arrows). The radiograph was made to investigate the proximal soft tissue swelling. Figure 5.180 Tarsal osteochondrosis. A. Lateral view of a dog’s right tarsus depicting the normal area of less opacity between the distal tibia and the calcaneus (arrow). B. Lateral view of the same dog’s left tarsus depicting tibiotarsal joint effusion (arrows). C. Dorsoplantar (DP) view of the dog’s right tarsus depicting the normal medial trochlear ridge of the talus (arrow). D. DP view of the dog’s left tarsus depicting an osteochondrosis lesion on the talus (black arrow) and soft tissue swelling due to joint effusion (yellow arrows). The articular border of the medial trochlear ridge of the talus is flattened and the joint space widened. Note: radiographs C and D are oriented the same way for ease of comparison. Figure 5.191 Normal vertebra. Cross‐sectional CT image of a vertebra illustrating the spinal cord surrounded by fat, the nucleus pulposus, and the annulus fibrosis. Figure 5.192 Lumbar intumescence. Lateral view of a dog lumbar spine illustrating the normal widening of the spinal canal between L3 and L5 (highlighted in red). Figure 5.193 Cross‐section of the spinal cord. The anatomy of the spinal cord meninges is illustrated. The pia mater covers the spinal cord; it is thin and delicate but impermeable to fluids. The arachnoid mater, named for its spiderweb like appearance, surrounds the pia mater and spinal cord, forming a loose sac. The subarachnoid space contains the cerebrospinal fluid. The dura mater is a tough outer layer. The subdural space is a potential space located between the dura and arachnoid. It can enlarge with fluid following trauma. The epidural space surrounds the dura mater and spinal nerve roots. Lymphatics, blood vessels, and fatty tissue are located in the epidural space. Figure 5.194 Positioning for lateral view of the spine. A. With the patient in lateral recumbency, the cervical and lumbar spinal regions normally sag, which can cause distortion and superimposition artifacts in the radiograph. B. Placing a foam wedge under the patients nose and small towels under the cervical and lumbar regions helps support the spine to better align the vertebrae. Figure 5.195 Rotation of the spine in a lateral view. These illustrations depict a patient in right lateral recumbency. The viewpoint is from the caudal aspect of the patient. The dotted lines represent the horizontal and vertical planes of the lumbar vertebrae. A. Without supporting the pelvic limbs, the lumbar planes naturally rotate as shown. B. Adding support, such as a rolled towel, between the pelvic limbs helps correct the rotation. Figure 5.181 Normal cervical vertebrae. Lateral view of a dog cervical spine depicting normal anatomy. Figure 5.182 Normal cervical vertebrae. VD view of a dog cervical spine depicting normal anatomy. Figure 5.183 Normal thoracic vertebrae. Lateral view of a dog thoracic spine depicting normal anatomy. Figure 5.184 Normal lumbar vertebrae. Lateral view of a dog lumbar spine depicting normal anatomy. Figure 5.186 Normal lumbar vertebrae. Lateral view of a dog lumbar spine depicting normal anatomy. The spinous processes are highlighted for illustration purposes. Accessory processes begin at about T11 and get progressively larger caudal to L6. Because they overlie the intervertebral foramen, they may be mistaken for herniated disc material. Figure 5.185 Normal lumbar vertebrae. VD view of a dog lumbar spine depicting normal anatomy. Figure 5.187 Normal thoracic vertebrae. Lateral view of a cat thoracic spine depicting normal anatomy. The black arrow points to the relatively narrower intervertebral disc space at the anticlinal junction. Figure 5.188 Normal lumbar vertebrae. Lateral view of a cat lumbar spine depicting normal anatomy (compare with Figure 5.184). The lumbar vertebrae in cats are relatively longer than in dogs. Figure 5.189 Normal caudal vertebrae. Lateral view of a cat pelvis and cranial tail depicting normal anatomy. The arrow points to a hemal arch. Figure 5.190 Normal immature dog spine. Lateral view of a 3‐month‐old puppy lumbar spine depicting the normal appearance of the vertebrae. The vertebral endplates have not yet fused with the vertebral bodies; the physes are still open. Figure 5.196 Dural ossification. Lateral view of a dog lumbar spine depicting thin mineral opacity lines along the ventral and dorsal spinal canal (arrows). Figure 5.197 Spinal DJD. Lateral view of a dog lumbar spine depicting osteophytosis at the intervertebral joints (arrows). Figure 5.198 Spondylosis deformans. A. Lateral view of a dog lumbar spine depicting well‐defined, smooth‐bordered new bone production along the ventral aspects of multiple vertebral bodies (arrows). B. VD view of the same dog depicting well‐defined exostoses along the lateral aspects of the lumbar vertebral bodies (arrows). C. Lateral view of a dog lumbar vertebrae depicting interdigitation of the exostoses along the ventral vertebral bodies, bridging the intervertebral disc spaces. Spondylosis does not involve the vertebral body endplates. However, it does begin very near the endplates at the attachments of the ventral longitudinal ligament. D. Lateral view of a dog lumbar spine depicting severe spondylosis with DJD (arrows point to osteophytes at the vertebral articulations). Figure 5.199 DISH. Lateral view of a dog thoracolumbar spine depicting extensive new bone production along the ventral and dorsal aspects of multiple vertebrae with pseudoarthrosis along the dorsal spinous processes. Figure 5.200 Vertebral neoplasia. Lateral view (A) and VD view (B) of a dog cervical spine depicting a moth‐eaten pattern of osteolysis in the sixth cervical vertebra (arrow). Figure 5.201 Vertebral tumor. Lateral view (A) and VD view (B) of a dog lumbar spine depicting marked osteolysis in the dorsal part of the sixth lumbar vertebra with an amorphous periosteal response (arrow). The vertebral body is increased in opacity, which sometimes is an early but non‐specific radiographic finding with vertebral neoplasia. Figure 5.202 Multiple myeloma. Lateral view of a dog spine depicting multiple foci of osteolysis in multiple vertebrae, most severe in L4 and L5 (arrows). Figure 5.203 Hemivertebrae. Lateral view (A) and VD view (B) of a dog thoracic spine depicting scoliosis due to multiple hemivertebrae. Arrows point to a ventrally wedged vertebra in the lateral view and a “butterfly‐shaped” vertebra in the VD view. Figure 5.204 Transitional vertebra. Lateral view (A) and VD view (B) of a dog thoracolumbar spine depicting “lumbarization” of T13. There is a partially formed rib on the right side (yellow arrow) and a transverse spinous process on the left (black arrow). Figure 5.205 Transitional vertebra. VD view of a dog pelvis depicting “sacralization” of the seventh lumbar vertebra. The left side of L7 is partially fused with the left ilium to form part of a sacroiliac joint (yellow arrow). On the right side of L7 is a transverse process (black arrow). Figure 5.206 Block vertebra. Lateral view of a dog cervical spine depicting congenital fusion of the third and fourth cervical vertebrae (arrows). There is no intervertebral disc space between them. A myelogram had been performed on this dog and is normal. Figure 5.207 Atlantoaxial subluxation. Lateral view (A) and VD view (B) of a dog cervical region depicting dorsal displacement of C2 in relation to C1. There is widening of the space between C1 and C2 (yellow arrow in A). The dens is absent (yellow arrow in B). The red arrows point to the endotracheal tube. Figure 5.208 Spina bifida. Close‐up VD view of a dog cervicothoracic spine depicting an incompletely fused dorsal spinous process on T1 (arrow). Figure 5.209 Overriding fractures. Lateral view of an immature dog lumbosacral spine depicting a fracture through the physis of the cranial endplate of L7 (yellow arrow) and through the second caudal vertebra (black arrow). The fractures are dorsally and cranially displaced and overriding. Figure 5.210 Compression fracture. Lateral view of a dog thoracolumbar spine depicting a compression fracture in the second lumbar vertebra (arrow). Figure 5.211 Osteochondrosis. Lateral view of a dog lumbosacral junction depicting a focal defect and a small mineralized fragment at the craniodorsal aspect of the first sacral vertebra (arrow). Figure 5.212 Intervertebral disk herniation. Lateral view (A) and VD view (B) of a dog thoracolumbar spine depicting a narrowed intervertebral disc space at T12‐13 (yellow arrow). There is increased opacity in the intervertebral foramen at this site. Multiple in situ mineralized intervertebral discs are visible (black arrowheads). A myelogram was performed on this dog and is shown in Figure 5.213). Figure 5.213 Intervertebral disk herniation. Lateral view (A) and VD view (B) of the same dog as in Figure 5.212. Contrast medium outlines the spinal cord except at T12–13, where there is little to no filling of the subarachnoid space (arrow). In this area, the spinal cord is swollen and/or compressed by herniated intervertebral disc material. Figure 5.214 Intervertebral disc herniation. Lateral view of a cat lumbar spine depicting a narrowed intervertebral disc space at L4–5 (arrow). Notice the increased opacity in the intervertebral foramen at this site. Figure 5.215 Spine positioning. Two lateral views of the same dog lumbar spine depicting the appearance of the vertebrae when the spinal column sags (A) and when it is supported (B). Compare the appearances of the L2–4 intervertebral disc spaces and foramina (arrows) in A and B. Figure 5.216 Intervertebral disc herniation. Cross‐sectional CT images of a lumbar vertebra illustrating normal anatomy (A), an acute intervertebral disc rupture and herniation (B), and a chronic disc herniation (C). Figure 5.217 Lumbosacral instability. A. A standard lateral view of a dog lumbosacral junction depicts normal alignment of the sacrum and last lumbar vertebra and a uniform spinal canal (dotted lines). B. An extended lateral view of the same dog depicts slight ventral displacement of the sacrum in relation to the last lumbar vertebra, resulting in a “stair‐step” narrowing of the spinal canal and dorsal wedging of the intervertebral disk space. Figure 5.218 Discospondylitis. Lateral view of a dog lumbar spine depicting multiple sites of osteolysis in the vertebral body endplates with adjacent osteosclerosis and widening of the intervertebral disk spaces (arrows). Figure 5.219 Schmorl’s node. Lateral view of a dog lumbar spine depicting focal concave defects centrally located in the opposing endplates of L4 and L5 (arrow) due to herniation of disc material into the adjacent vertebrae. Figure 5.220 Cervical spondylomyelopathy. Lateral view of a dog cervical spine depicting subluxation at C5–6. The cranial end of C6 is dorsally displaced in relation to C5 (arrow), creating a “stair‐step” along the ventral spinal canal. Figure 5.221 Cervical spondylopathy. A. Extended lateral view of a dog cervical spine. C6 is identified by its large ventral lamina. The cranioventral border of C7 is flattened (arrow). B. Flexed lateral view of the same dog. C6 is dorsally displaced in relation to C5 due to subluxation at C5–6 (yellow arrow). The black arrow points to the flattened area of C7. Figure 5.222 Cervical spondylomyelopathy. Lateral view of a dog cervical spine myelogram depicting dorsal compression of the spinal cord at C5–6 (arrow) caused by a malformation of C6 that narrows the spinal canal. Figure 5.223 Cervical spondylomyelopathy. Lateral view of a dog cervical spine depicting a narrowed intervertebral disc space and degenerative changes at C6–7 (arrow) due to chronic vertebral instability and intervertebral disc disease. Figure 5.224 Normal dog skull. Lateral view of a dog head depicting normal anatomy. An endotracheal tube is present. Figure 5.225 Normal dog skull. DV view of a dog head depicting normal anatomy. The vomer bone is the visible bony part of the nasal septum. Figure 5.226 Normal cat skull. Lateral view of a cat head depicting normal anatomy. The ethmoid turbinates are in the nasal cavities surrounded by the maxillary bone. The very opaque bone at the dorsal aspect of the tympanic bullae is the petrous temporal bone. Figure 5.227 Normal skull. DV view of a cat skull depicting normal anatomy. The orbits are located just medial to zygomatic arches. Figure 5.228 Normal dog maxilla. Open‐mouth VD view of a dog head depicting normal anatomy. Figure 5.229 Normal immature dog skull. Lateral view of a 2‐month‐old puppy head. Figure 5.232 Modified Triadan numbering system. DV views of a normal dog head illustrating the maxillary teeth (A) and the mandibular teeth (B). Each tooth is identified by a three‐digit number. The first digit indicates the quadrant: 1 is right maxillary, 2 is left maxillary, 3 is left mandibular, and 4 is right mandibular. The second and third digits denote the tooth position in that quadrant. Note: the numbering always begins on midline. Figure 5.233 Modified Triadan numbering system. DV views of a normal cat skull illustrating the maxillary dental arcade (A) and the mandibular dental arcade (B). Each tooth is identified by a three‐digit number as described in Figure 5.230. Figure 5.230 Normal tooth. Lateral view of a dog mandible depicting normal anatomy of the first molar tooth. The periodontal ligament fills the periodontal space. Figure 5.231 Normal teeth. Intraoral view of a dog rostral maxilla depicting normal incisor teeth. Figure 5.234 Normal pharynx and larynx. Lateral view of a dog cervical region depicting normal anatomy. The larynx includes the epiglottis, arytenoid cartilage, cricoid cartilage, and thyroid cartilage. The tip of the epiglottis lies just dorsal to the soft palate. The lateral ventricles are superimposed on the thyroid cartilage. The paired hyoid bones are labeled s = stylohyoid, e = epihyoid, c = ceratohyoid, and t = thyrohyoid. The basihyoid is unpaired and in the lateral view appears quite opaque because it is viewed end‐on resulting in summation along the length of the bone. There is gas in the cranial esophagus; the gas outlines the caudal part of the cranial esophageal sphincter. Figure 5.235 Retropharyngeal space. Lateral view of a dog cervical region illustrating the normal size of the retropharyngeal space. In general, with the head in a neutral position (about 135° with the neck), the width of the retropharyngeal space (yellow double‐headed arrow) should not exceed the length of C3 (black double headed arrow). The width is measured from the dorsal edge of the cricoid cartilage (cc) to the ventral plane of C2. Figure 5.236 Craniomandibular osteopathy. Lateral view (A) and DV view (B) of a dog head depicting prolific new bone production along the right and left hemimandibles (arrows). Periosteal new bone is irregular but well‐defined. Figure 5.237 Skull fracture. Lateral oblique view (A) and skyline view (B) of a brachycephalic dog depicting a comminuted fracture in the right frontal bone (arrows) with mild soft tissue swelling and subcutaneous emphysema. Figure 5.238 Skull fracture. DV view of a dog head depicting a medially displaced segmental fracture in the right zygomatic bone (arrows) and mild soft tissue swelling. Figure 5.239 Skull fracture. Lateral oblique view of an immature dog head. The radiograph was made tangential to an area of soft tissue swelling to evaluate that part of the skull. The arrow points to a depressed fracture along the dosolateral calvarium. Figure 5.240 Hydrocephalus. Lateral view (A) and DV view (B) of a puppy with an expanded cranial vault and thinning of the calvarium. The cranial vault is homogeneous in appearance. Figure 5.241 Chronic otitis. Lateral view (A), lateral oblique view (B), DV view (C) and open mouth rostrocaudal view (D) of a cat head depicting increased opacity in the right tympanic bulla and thickening of the right bulla wall (yellow arrows). The left bulla is normal. In the oblique view, the left bulla (black arrow) is positioned dorsal to the right. In the DV view, gas is absent from the right external auditory canal due to soft tissue swelling and there is faint linear mineralization in the canal. Figure 5.242 Nasopharyngeal mass. Lateral view of a cat head depicting soft tissue opacity in the rostral half of the nasopharynx. The arrow points to the caudal border of a soft tissue mass. Figure 5.243 Rhinitis. Open‐mouth VD view of a dog head depicting increased opacity in both nasal cavities. The turbinates are effaced, but still visible, without evidence of osteolysis at this time. Figure 5.244 Aggressive lesion. Open mouth VD view of a dog head depicting geographic osteolysis that involves both nasal cavities. The arrow points to destruction of the vomer bone (nasal septum). Figure 5.245 Nasal cavity lesions. VD view (A) and open‐mouth VD view (B) of the same dog head depicting unilateral increased opacity in the left nasal cavity. The full extent of the is easier to see without superimposition of the mandible. Figure 5.246 Rhinitis. Open‐mouth VD view of a dog head depicting decreased opacity and osteolysis in the rostral portion of the left nasal cavity and increased opacity in the caudal portion. The rostral fine bony structures associated with the nasal turbinates are lost. The ethmoid turbinates are visible through the increased opacity and appear intact. The radiographic appearance is typical for a fungal infection but not diagnostic of a mycosis. Neoplasia or bacterial infection can produce similar findings. Laboratory testing is necessary for diagnosis. Figure 5.247 Nasal neoplasia. A. Open‐mouth VD view of a dog head depicting increased opacity throughout the right nasal cavity with an indistinct nasal septum (arrow) and loss of fine bony structures. B. Open‐mouth VD view of a dog head depicting a mass in the right nasal cavity resulting in osteolysis and left lateral deviation of the nasal septum (arrow). Figure 5.248 Frontal sinus mucocele. Rostrocaudal view of a dog head depicting increased opacity in the left frontal sinus without evidence of osteolysis. A similar appearance may result from neoplasia or inflammation in the sinus. Figure 5.249 Normal temporomandibular joint. A. Lateral view of a dog head made with the nose tilted to image the left TMJ (yellow arrow). The right TMJ (black arrow) is not aligned with the x‐ray beam and is not well visualized. B. Close‐up of the left TMJ depicting the normal anatomy. Figure 5.250 Temporomandibular joint luxation. DV view (A), open mouth lateral view (B), and standard lateral view (C) of a cat head depicting rostral luxation of the right TMJ (black arrow). The left TMJ (yellow arrow) is normal. The standard lateral view (C) was made following reduction of the TMJ luxation. Figure 5.251 Dental disease. Lateral view of a mandible depicting widening of the periodontal space due to osteolysis (black arrow) and defects (cavities) in the tooth crown (yellow arrow) and tooth root (white arrow). The pulp cavity in the cranial root of the affected tooth is enlarged due to tooth decay. Figure 5.252 Dental disease. Lateral view of a mandible depicting a tooth root abscess (black arrow) and resorption of alveolar crest (white arrow). Figure 5.253 Dental disease. Lateral view of a mandible depicting a retained tooth root (yellow arrow) and periodontal disease (black arrow). Figure 5.254 Tooth root abscess. Intraoral oblique view of a dog rostral mandible depicting osteolysis at the apical foramen of a canine tooth root (black arrow) and enlargement of the pulp cavity (yellow arrow). Figure 5.255 Hyperparathyroidism. Lateral view (A) and VD view (B) of a dog head and a lateral view (C) of the lumbar spine depicting severe demineralization of bone due to nutritional secondary hyperparathyroidism. The teeth appear to “float” in the soft tissue opacity mandible and maxilla. Figure 5.256 Dentigerous cyst. Lateral oblique view (A) and DV view (B) of a dog head depicting a well‐defined expansile osteolytic lesion in the right rostral hemimandible (yellow arrows). Figure 5.257 Salivary glands. Lateral view of a dog head illustrating the salivary glands. Figure 5.258 Retropharyngeal swelling. Lateral view of a dog cervical region depicting ventral displacement of the dorsal pharyngeal wall (yellow arrow) resulting in narrowing of the pharynx. There is incidental mineralization in the epiglottis (black arrow) and the soft palate is thickened. Parosteal osteosarcomas arise from the surface of cortical bone as opposed to most osteosarcomas which arise in cancellous bone. They typically appear as a smooth‐bordered, lobulated, mineral opacity mass extending outward from the border of a long bone. The bony mass usually is sclerotic with a well‐defined margin. There often is little or no osteolysis. Over time, the mass may eventually invade the cortex and extend into the medullary cavity. Parosteal osteosarcomas, or juxtacortical osteosarcomas, are uncommon in dogs and cats. They tend to be slower growing and carry a better prognosis than typical osteosarcomas, but they can metastasize. In cats, parosteal osteosarcomas tend to arise in the humerus or femur, whereas in dogs, they are more likely to occur near the stifle. Although more common in long bones, they have been reported in a frontal bone and a mandibular ramus. Giant cell tumors are rare in dogs and cats. They most often are reported in the metaphyseal or epiphyseal region of a long bone, particularly the distal ulna. They are slow growing and usually benign. In radiographs, giant cell tumors typically produce an expansile area of geographic osteolysis with a septated or multiloculated center and a well‐defined margin. In most cases, there is no visible periosteal response. If a response is present, rule out osteosarcoma. A giant cell tumor may resemble a bone cyst, but the tumor tends to grow, which often is evident in serial radiographs. Secondary bone tumors originate elsewhere in the body and spread to bone. They are uncommon in dogs and cats. However, any malignant tumor can involve bone by direct extension or metastasis. Examples of soft tissue tumors that can invade bone include a squamous cell carcinoma that invades the mandible or the digits and a synovial cell sarcoma that invades the adjacent bones. Soft tissue tumors also can cause pressure remodeling or a periosteal response in an adjacent bone without directly invading it. Malignant tumors that arise in bone, lung, prostate gland, and mammary glands tend to metastasize to bone more often than those arising in other tissues. Metastasis usually is polyostotic and often involves the diaphyseal and metaphyseal regions of long bones. Lesions typically are aggressive with some degree of cortical destruction. The initial periosteal response often is small, smooth bordered, and well‐defined, particularly in the ribs, but can become much more active. Multiple myeloma and lymphoma tend to produce multiple osteolytic foci with little or no periosteal response (Figure 5.43). These often occur at sites not typical for primary neoplasia. Lymphoma may produce medullary osteosclerosis. Digital tumors may originate in bone or in the adjacent soft tissue. The typical radiographic findings are osteolysis in the affected digit, adjacent soft tissue swelling, and little to no visible periosteal response (Figure 5.121). Most digital tumors are subungual and confined to the third phalanx. They rarely cross the joint, but they can. Infection in a digit that leads to osteomyelitis is more likely to cross a joint, but not always. Again, histopathology usually is needed for diagnosis. Subungual tumors are reported most often in older, large‐breed dogs, particularly those with black hair coats. They are uncommon in cats. Usually, only a single digit is involved, but tumors can arise in multiple digits and in multiple limbs. Benign bone diseases change slowly over time and rarely involve other tissues. They are uncommon in dogs and cats, more often reported in young, large breed dogs. Any suspected benign lesion must be proven to be benign with serial radiographs. A malignant bone tumor is much more likely that a bone cyst or a benign tumor. Most benign bone diseases are difficult to differentiate with radiographs. Some will grow and may eventually interfere with limb or joint function, but most remain asymptomatic. Larger lesions can impinge on an adjacent structure or weaken the bone and predispose it to a pathologic fracture. Occasionally a skeletal deformity is present at the site of a benign lesion. A bone cyst is a cavity within a bone. The cavity often is filled with fluid. In radiographs, a bone cyst appears as an area of geographic osteolysis with a well‐defined margin (Figure 5.44). The center often is septated. The adjacent cortex may be thinned or displaced, but it remains intact. If the cortex becomes too thin, however, a pathologic fracture may occur. A periosteal response is rare and soft tissue swelling often is minimal or absent. Most bone cysts are monostotic and develop in the diaphyseal or metaphyseal region of a long bone. They more often are reported in the distal radius or ulna, but can affect any bone. In the spine, bone cysts are more likely to be located in a dorsal spinous process than in a vertebral body. The etiology of most bone cysts is unknown. A simple or solitary bone cyst (unicameral cyst) sometimes develops from a hematoma that formed following a traumatic event. An aneurysmal bone cyst may result from the arteriovenous shunting of blood that causes expansile remodeling in the bone. An odontogenic bone cyst is associated with teeth that are devitalized, malformed, impacted, or unerupted. Enchondromas and osteochondromas are benign cartilaginous tumors that develop from displaced growth cartilage. The cartilage continues to grow in the abnormal location and functions similar to a physis. These tumors are typically soft tissue opacity due to their cartilage content. Some may contain mineralized tissue, often in the form of a thin rim of bone. Differentiating enchondroma from osteochondroma using radiographs often is difficult and may be clinically unimportant. An enchondroma is derived from the actively proliferating cartilaginous tissue of the physis. Its typical location is the endosteal surface of the bone where it causes an expansile lesion that thins the adjacent cortex (Figure 5.45). Usually, there is no periosteal response or significant soft tissue swelling. The center of the lesion may contain bony trabeculae. Enchondromas typically form in the metaphyseal or diaphyseal region of one or more long bones, most often in a distal limb. Multiple sites of enchondroma is called enchondromatosis. An osteochondroma is derived from aberrant physeal cartilage that separates from the edge of a normal growth plate. As the patient grows, the separated piece of cartilage also grows, but in an abnormal location. Osteochondromas can arise in any bone that develops from cartilage. They appear as well‐defined expansile lesions without evidence of osteolysis, soft tissue swelling, or an active periosteal response. In the early stages, an osteochondroma may appear separate from the underlying cortex, sometimes resembling calcinosis circumscripta. Osteochondromas may be solitary or multiple, monostotic or polyostotic. The presence of multiple osteochondromas is called osteochondromatosis or multiple cartilaginous exostosis. In dogs and cats, osteochondromas may be found anywhere but most often in two specific locations: the distal radius and the femoral shaft. In the distal radius, they typically appear as a broad‐based growth, sometimes interfering with the ulna to cause pain and lameness. In the femur, they typically appear as a spur‐shaped growth extending from the caudal border of the midshaft (Figure 5.46). Osteochondromas usually stop growing at skeletal maturity. Rarely, a symmetrical or annular osteochondroma at the physis will lead to limb shortening or deformity. Although uncommon, osteochondromas can develop in the trachea, in joints, and sometimes in flat or irregular bones. They have been reported in the pelvis, spine, and skull, particularly in cats, but tend to be less organized in these areas than in long bones. Spinal osteochondromas more often occur in the cervical or thoracic spine, usually near a spinous process. Osteomas are benign, slow‐growing tumors that arise from the surface of a bone. They may be difficult to differentiate from parosteal osteosarcomas. The typical appearance of an osteoma is a very opaque bony mass extending away from the cortex. The margins of the mass tend to be smooth and well‐defined without evidence of osteolysis and with little to no soft tissue swelling. Osteomas more often involve the cranial vault, a mandible, or a sinus. Other than a firm swelling, most affected animals are asymptomatic. Chondromas are benign, slow‐growing tumors of cartilage. They arise in flat bones, such as the ribs, more often than long bones. In radiographs, a chondroma appears as a well‐defined, expansile, soft tissue opacity mass in the bone. Any periosteal response usually is minimal with smooth, well‐defined margins and little soft tissue swelling. Chondromas typically are less mineralized than osteochondromas. Synovial osteochondromas appear as one or more well‐defined, mineral opacity structures in a joint (Figure 5.47). They may be round or irregular in shape and often occur bilaterally (radiographs of the contralateral joint are recommended). The etiology of synovial osteochondromas often is unknown. They are seen more often in cats than in dogs, particularly in mature animals. Osteochondromas nourished by synovial fluid can continue to grow. In cats, the joints most often involved are the stifles, elbows, shoulders, and digits. In dogs, it is the hips, stifles, and elbows. In dogs, synovial osteochondromas are more common in larger breeds and with chronic osteochondritis dissecans. When multiple joints are affected, the condition is called synovial chondromatosis. Congenital absence of bones is uncommon in dogs and cats. Supernumerary bones are more common, particularly in the digits. Some conditions may be heritable. Ectrodactyly is the abnormal development of bones in a limb. It most often occurs in the metatarsus or metacarpus where one or more of the central digits is absent or reduced in size (Figures 5.48 and 5.49). The remaining digits may be further separated than normal or fused together, commonly called split hand deformity or lobster‐claw syndrome. The condition usually is unilateral. The ipsilateral elbow may be subluxated or luxated. Hemimelia is the partial or complete absence of a normally paired bone (i.e., radius/ulna or tibia/fibula). Most often, it is the radius or tibia that is affected. The existing paired bone tends to be larger in diameter with thicker cortices than normal due to stress remodeling (Figure 5.50). The joints proximal and distal may be subluxated or luxated. Polydactyly is an increase in the number of digits on one or more limbs. It is a common inherited trait in cats and certain dog breeds (e.g., Great Pyrenees). More than one extra digits may be present (Figure 5.51). The development of the extra digit(s) may be partial or complete and may also include extra metacarpal or metatarsal bones or just extra phalanges. Syndactyly is the bony or soft tissue fusion of two or more adjacent digits. The bones may either blend together as a bony union or they may remain separate but in close apposition with one another due to a fibrous union. Commonly called dwarfism, chondrodysplasia is a skeletal deformity caused by abnormal endochondral ossification. It may be proportionate, affecting all bones in the skeleton equally, or it may be disproportionate, leading to abnormalities in some bones but not in others. Chondrodysplasia is deliberately bred into certain breeds of dogs and cats (e.g., Dachshund, Basset Hound, Bulldog, Pug, Pekinese, Lhasa Apso, Welsh Corgi, Munchkin cats). Proportionate dwarfism is usually caused by inadequate growth hormone, which often is a pituitary problem. Affected animals are diminutive in stature and slow to grow. They tend to retain their juvenile hair coats, though symmetrical alopecia and hyperpigmentation often develop later in life. In radiographs, all bones are smaller than normal but proportionate in size. Formation of ossification centers and physeal closure times tend to be similar or slightly delayed compared to non‐chondrodystrophic animals. Disproportionate dwarfism can result in numerous skeletal abnormalities, including stunted bone growth, asynchronous growth of paired bones, varus or valgus limb deformities, hemivertebrae, joint subluxations, and others. Many affected animals also suffer stenotic nares and elongated soft palates. In radiographs of immature animals, the epiphyses may appear mottled due to delayed ossification, a condition called epiphyseal dysplasia (Figure 5.52). Epiphyseal dysplasia may be visible up to about 4 months of age, after which ossification usually has progressed to the point that the mottling is no longer evident. Mottling tends to be more evident in the humeral condyles, carpi, and tarsi, but the femurs, metacarpi/metatarsi, and vertebrae usually are also involved. Closure of the physes frequently is delayed leading to wider and more irregular growth plates. The metaphyses may be widened or “flared” and increased in opacity. In the appendicular skeleton, disproportionate dwarfism causes abnormal shortening of the bones in the extremities. The thoracic limbs tend to be more severely affected than the pelvic limbs. Asynchronous growth of the radius and ulna is common, leading to radius curvus and pes valgus deformities. Endochondral cartilage sometimes is retained, most often in the distal ulnar metaphysis. Development of the coronoid process, medial humeral condyle, and anconeal process may be delayed or incomplete due to chondrodysplasia. The carpal and tarsal bones may be misshapen. With maturity, the epiphyseal, physeal and metaphyseal abnormalities often regress; however, the long bone deformities are permanent. Secondary degenerative joint disease is common in affected animals, as is hip dysplasia. In the axial skeleton, abnormalities due to chondrodysplasia are less common. The vertebrae may be shortened, which affects body length, and fusion of the vertebral endplates to the vertebral bodies may be delayed. The ventral vertebral margins may appear irregular, sometimes described as “lipping” or “pleating.” The ends of the ribs may be flared and concave or “cupped” at the costochondral junctions. Congenital hypothyroidism or cretinsim can result in disproportionate dwarfism. Low levels of thyroid hormone during growth may be due to hypoplasia or aplasia of the thyroid gland, defective thyroid hormone synthesis, or an iodine deficiency. If hypothyroidism occurs after cessation of bone growth, no dwarfism or skeletal changes develop. Congenital hypothyroidism most often is reported in Boxers and sometimes in other breeds. Affected dogs present with short, bowed limbs and long bodies. Radiographic findings include epiphyseal dysplasia, delayed physeal closures, and delayed ossification. Many of the long bones are short and bowed with thick cortices, particularly the proximal tibia, distal femur, and distal humerus. The medullary canals may be increased in opacity. Secondary degenerative joint disease is common. The vertebrae frequently are abnormal in shape and shortened due to endplate dysplasia, often leading to spinal kyphosis. The skull usually is shorter and broader than normal due to delayed closure of the fontanelles and shortened facial bones. Dental eruption usually is delayed. Concurrent hydrocephalus may be present. The abnormal, excessive production of parathyroid hormone leads to loss of calcium from the skeleton and generalized osteoporosis. In radiographs, bone is less opaque, there is less bone‐to‐soft tissue contrast, bone cortices become thin, and there is a coarse trabecular pattern. Osteoporosis commonly leads to pathologic fractures. Long bones may be deformed and in various stages of fracture healing. Malunion fractures frequently are present. One of the earliest radiographic signs of hyperparathyroidism often is loss of the dental lamina dura. In advanced cases of osteoporosis, fibrous osteodystrophy develops and the teeth may appear to “float” in soft tissue due to the severe loss of bone opacity. There are three types of hyperparathyroidism: primary, secondary, and pseudo‐hyperparathyroidism. Primary hyperparathyroidism is caused by disease in one or more of the parathyroid glands, often a tumor. Secondary hyperparathyroidism is caused by an imbalance in the body’s calcium to phosphorous ratio; usually due to either excess dietary phosphorous or abnormal retention of phosphorous due to chronic renal disease. Both of these conditions can result in the overproduction of parathormone. Pseudo‐hyperparathyroidism is associated with a malignant tumor that causes hypercalcemia as part of a paraneoplastic syndrome. It has been reported with lymphoma, multiple myeloma, and others. The parathyroid glands are normal but overactive. Osteogenesis imperfecta presents as generalized osteoporosis due to a rare defect in collagen production. It may be confused with hyperparathyroidism. Growth deformities can occur in young animals, and pathologic fractures are common. Affected animals may exhibit some degree of dwarfism. The rib cage typically is small and may compress the intrathoracic viscera. Rickets is a disease of growing bones caused by a dietary deficiency, usually a lack of vitamin D. It is rare in dogs and cats. Rickets, sometimes called juvenile osteomalacia, results in abnormal ossification that leads to softening and weakening of the bones. In radiographs, the physes appear wider and more irregular than normal (Figure 5.53). The metaphyses often are widened or flared and concave or “cupped” with more pointed edges. The epiphyses, however, are normal, which helps differentiate rickets from other causes of skeletal dysplasia. There is little or no soft tissue swelling. Rickets affects all bones, but abnormalities tend to be more severe in the distal radius, ulna, and tibia. Untreated cases can progress to long bone deformities. This is a group of heritable diseases characterized by a buildup of mucopolysaccharides (MPS), also called glycosaminoglycans. MPS are long‐chain sugar molecules that must be degraded by lysosomal enzymes for normal growth to occur. If the enzymes are abnormal, MPS can accumulate in the body and damage the musculoskeletal, circulatory, and neurologic systems. The type of damage varies with the type of enzyme deficiency but usually progresses as the animal ages. Mucopolysaccharidosis can cause disproportionate dwarfism, which usually is evident by 8 weeks of age. Skeletal abnormalities include epiphyseal dysplasia, flared metaphyses, and short, deformed limbs. The diaphysis often are enlarged. Fascial dysmorphism is common with a large skull, short maxillary, incisive, and mandibular bones, small or absent frontal sinuses, and abnormal teeth. The vertebrae may be fused, shortened, and/or misshapen, sometimes cuboid in appearance, especially along the cervical and lumbar spine. The odontoid process (dens) may be hypoplastic or fragmented. The vertebral body endplates frequently are sclerotic. Degenerative changes may be present along the dorsal vertebral articulations. The clavicles and distal ends of the ribs may be widened. Pectus excavatum often is present. Many affected animals have hip dysplasia and other appendicular joints may be deformed. Degenerative joint disease is common. Generalized osteoporosis usually is present, varying from mild to severe. Mucopolysaccharidosis is uncommon in dogs and cats, most often reported in Siamese cats. In addition to skeletal deformities, the disease may lead to cardiac anomalies, hydrocephalus, paresis or paraplegia, and ocular abnormalities. CLAD is rare inherited condition that impairs the function of granulocytes. Affected animals exhibit stunted growth and often suffer recurrent bacterial infections. Bony abnormalities include a moderate to severe periosteal response along the mandible (may resemble craniomandibular osteopathy), increased opacity in the frontal sinuses, and widening or flaring of the metaphyses. Metaphyseal bone may appear heterogenous, sometimes described as stippled. Osteomyelitis often is present. CLAD has been reported in several dog breeds, including Irish Setter, Irish Red and White Setter, Doberman Pinscher, and Weimaraner. The disease is invariably fatal, with most animals dying at a few months of age. As mentioned earlier, the physes are susceptible to injury and the distal ulnar physis in dogs is particularly vulnerable due to its unique conical shape. Damage to a physis can lead to abnormal bone growth and a limb deformity, especially when it results in disproportionate or asynchronous growth between paired bones (e.g., radius and ulna, tibia and fibula). The greater the damage and the younger the animal, the more severe the limb deformity. Early diagnosis is crucial to successful treatment, which can minimize future problems with the leg. Serial and comparison radiographs often are useful for diagnosis. When investigating a thoracic limb deformity, make the following evaluations: Damage to the distal ulnar physis that results in asynchronous growth with the radius leads to radius curvus, elbow subluxation, and carpal valgus. The abnormally shortened ulna causes the radial diaphysis to curve cranially and medially. The abnormal curvature is called “bowing” due to the “bowstring” effect of the ulna, which is fixed at the carpus and humerus. The radial cortex thickens along the concave side due to stress remodeling. Shortening of the ulna also can lead to widening of the humeroulnar joint space, making the space more wedge‐shaped (Figures 5.54 and 5.55). The ulnar medial coronoid process moves distal to the radial head, creating a “stair step” along the radioulnar articular border (Figure 5.56). The normal radioulnar border is smooth. Greater than 2 mm displacement is significant. At the carpus, the shortened ulna causes the manus to deviate laterally because the ulnar styloid process is further proximal than normal. Severe disproportionate growth may lead to a very short ulna and luxation of the humeroradial joint with lateral displacement of the radial head (Figure 5.57). Abnormal stress on the anconeal process may prevent fusion or deform or fracture the process. A very short ulna also can lead to luxation of the radiocarpal joint. Damage to the distal radial physis that results in asynchronous growth often leads to elbow subluxation and carpal varus. The abnormally shortened radius can cause widening of the humeroradial and radiocarpal joint spaces (Figure 5.58). The manus may deviate laterally because the ulnar styloid process is further distal than normal. Damage that affects only part of the distal radial physis can result in one side of the physis growing slower than the other side (Figure 5.59). In this situation, the manus deviates toward the damaged side and the radial diaphysis may curve or bow toward the normal side (concave side of diaphysis toward the damaged side of the physis). The humeroradial joint space may be widened. Damage to both the distal radial and distal ulnar physes that results in abnormal growth can lead to shortening of both the radius and ulna. The elbow or carpus may be subluxated depending on the degree of damage to each physis. Damage to the proximal radial physis that results in abnormal growth leads to shortening of the radius and findings similar to those seen with damage to the distal radial physis, but usually not as severe. Similar to physeal damage, fusion of the radius and ulna in an immature animal can result in asynchronous growth (Figure 5.60). Hypertrophic osteodystrophy is a systemic disease that affects the metaphyses of immature long bones (HOD sometimes is called metaphyseal osteopathy). Any metaphysis in any long bone can be affected, but lesions tend to be most severe in the distal radius and ulna. Lesions usually are bilateral and relatively symmetrical. The long bones distal to the carpus and tarsus often are spared. Rarely, HOD can affect the mandible, cranium, or ribs. The etiology of HOD is unknown. The initial radiographic sign of HOD is a thin line of soft tissue opacity near the physis (Figure 5.61), sometimes called a “double physis” sign. The line is caused by bleeding in the metaphyseal zone of provisional calcification that prevents mineralization in a thin band of trabecular bone. Bleeding also extends under the periosteum, separating it from the cortex. Typically, swelling develops in the adjacent soft tissues. A periosteal response produces mineral in the subperiosteal space, appearing in radiographs as a “cuff” or “sleeve” of new bone around the metaphysis. The subperiosteal space continues to fill with mineral over the next week or two, enlarging the “cuff” and making it more homogeneous. The metaphysis may appear more opaque due to summation of periosteal new bone (Figure 5.62). In chronic cases, the periosteal cuffing can extend to the physis and may bridge the physis to restrict bone growth and possibly lead to a limb deformity. HOD most often is reported in young, rapidly growing, large, and giant breed dogs. It often begins around 4 months of age. Affected animals typically present with swollen, painful metaphyses, which may be mistaken for joint swelling. Factors that may contribute to the development of HOD include vitamin C deficiency (HOD sometimes is called skeletal scurvy) and infection with canine distemper virus. HOD usually is a self‐limiting disease. Most cases resolve completely after a few weeks. Pain management sometimes is difficult. During resolution, the soft tissue swelling subsides and the metaphyseal “cuffs” gradually remodel and become smaller. Very severe cases of HOD occasionally are accompanied by septicemia and pneumonia, which rarely can be fatal. As we’ve discussed, HOD lesions occur in the area of the metaphyseal cutback zone. The normally irregular and indistinct margins in this area may be mistaken for pathology. Note: there is no soft tissue swelling or pain with normal growth. Hypertrophic osteopathy is a periosteal response that occurs secondary to a mass‐effect in the thoracic or abdominal cavity. The most common cause of HO is intra‐thoracic disease, particularly large lung tumors. HO has also been seen with infection in the thoracic or abdominal cavity, severe cardiomegaly, and secondary to certain tumors and large masses in the abdomen. The mechanism of HO is unknown, but periosteal new bone forms along the diaphyses of multiple long bones. Usually, all four limbs are involved. HO typically begins in the digits and progresses proximally. New bone formation tends to be bilateral, symmetrical, and progressive, typically with a palisading appearance (Figure 5.63). The margins of the new bone generally are ill‐defined during active production and become better defined over time. Margins usually are well‐defined in chronic cases (Figure 5.64). Soft tissue swelling is present in most cases, more severe during active bone production. The long bone cortices remain intact without evidence of osteolysis. If disease is unchecked, all long bones in all legs eventually will be affected. Rarely, other bones are involved (e.g., carpi, tarsi, vertebrae, pelvis). HO most often is reported in middle‐aged to older dogs but can occur in any age, breed, or gender. If the primary lesion is removed or otherwise resolved, the bone lesions gradually regress. Endochondral cartilage sometimes is retained in the metaphysis of a long bone due to failure of ossification. This occurs most often in the distal ulna, but it has been reported in the distal radius and in the lateral femoral condyle. In radiographs, the retained cartilage appears as a triangular or cone‐shaped area of soft tissue opacity in the metaphysis (Figure 5.65). The triangle or cone is widest at the physis and tapers toward the diaphysis. The cartilagenous core may be surrounded by a rim of osteosclerosis. Retention of cartilage sometimes is bilateral and radiographs of the contralateral limb are recommended. Retained cartilage cores most often are reported in young, rapidly growing, large and giant breed dogs (e.g., Great Dane, Saint Bernard, Setters). The retained cores in many cases are temporary, but some are permanent. Retained cartilage sometimes is seen in long bones that are slow growing and may or may not be the cause of the abnormal growth rate. Most retained cartilages are asymptomatic and may even be a variation of normal. Panosteitis is a painful bone disease of unknown etiology. Lesions due to panosteitis appear in radiographs as patchy areas of increased medullary opacity in one or more long bones (Figure 5.66). Lesions may resolve in one bone and manifest in another, appearing to “move” from bone to bone. More than one lesion may be present in a bone. Panosteitis usually is bilateral but not symmetrical. It tends to predominantly affect the larger long bones (e.g., humerus, femur, radius, ulna, tibia), but sometimes will involve the metacarpal and metatarsal bones. The areas of increased medullary opacity generally are irregular in shape with ill‐defined margins. They usually begin near a nutrient foramen. At the lesion sites, the bony trabeculae often appear hazy and sclerotic, and there is less corticomedullary contrast. In severe cases, lesions may occupy the majority of the diaphyseal region. A thin, smooth‐bordered periosteal response sometimes is present, typically with an ill‐defined margin during the active stages of panosteitis and a well‐defined margin when disease is inactive. Soft tissue swelling usually is minimal or absent. Panosteitis most often is reported in young, large and giant breed dogs (German Shepherds and Bassett Hounds are overrepresented). It occasionally is seen in older animals. Males more often are affected than females. Panosteitis is a self‐limiting disease characterized by a sudden onset of shifting leg lameness. Lameness may be protracted over several months and may involve a single limb or multiple limbs, either simultaneously or sequentially. The severity of the radiographic findings may not correlate with the severity of the clinical signs. During recovery from panosteitis, lesions regress and the bones eventually resume a normal appearance. In some cases, sclerotic lines may persist in the diaphyses. These narrow, transverse lines may resemble growth arrest lines. Another cause of medullary osteosclerosis that may mimic panosteitis is a bone infarct. Bone infarcts, however, are more focal and the margins tend to be sharper (Figure 5.26). Summation of periosteal new bone may be mistaken for increased medullary opacity, particularly in patients with HO or HOD. Determining the distribution of the lesions is helpful to differentiate panosteitis from HO and HOD (Box 5.2).
5
Musculoskeleton
Introduction to musculoskeletal radiography
Procedure for making musculoskeletal radiographs
Standard views of musculoskeletal structures
Supplemental views of musculoskeletal structures
Soft tissues
Site
Appearance
Fusion (Dogs)
Fusion (Cats)
Scapula
Supraglenoid tubercle
7–9 weeks
4–7 months
3–4 months
Humerus
Greater tubercle
2–3 weeks
4 months (to humeral head)
Proximal epiphysis (humeral head)
2 weeks
10–13 months
18–24 months
Distal epiphysis (humeral condyles)
(See condyles)
6–8 months (to diaphysis)
Lateral condyle
2–3 weeks
6 weeks (to medial condyle)
3–4 months
Medial condyle
3–6 weeks
6 weeks (to lateral condyle)
3–4 months
Medial epicondyle
7–9 weeks
6 months (to medial condyle)
3–5 months
Radius
Proximal epiphysis
3–5 weeks
5–11 months
6–7 months
Distal epiphysis
2–4 weeks
6–12 months
13–21 months
Ulna
Olecranon (tuberosity of olecranon)
7–9 weeks
6–10 months
8–12 months
Anconeal process
12 weeks
4–5 months
Distal epiphysis
7–8 weeks
7–12 months
13–24 months
Carpus
Radial carpal bone
3–4 weeks
Ulnar carpal bone
4–5 weeks
Central carpal bone
4–5 weeks
Intermediate carpal bone
2–4 weeks
Accessory carpal bone (body)
2 weeks
3–5 months
Accessory carpal bone (epiphysis)
7 weeks
3–6 months
First–fourth carpal bones
3–4 weeks
Sesamoid bone
16 weeks
Metacarpus/metatarsus
Distal epiphysis (MC I)
5–7 weeks
6–7 months
Distal epiphysis (MC II–V)
4–5 weeks
5–7 months
6–10 months
Phalanges
Proximal epiphysis
6–8 weeks
4–6 months
Dorsal sesamoids
4–5 months
4–5 months
Volar sesamoids
3–4 months
Pelvis
Ileum
Birth
4–6 months
Ischium
Birth
4–6 months
Acetabulum
7–11 weeks
4–6 months
Tuber ischii
3–5 months
8–10 months
Symphysis pubis
5–12 months
60 months (5 years)
Ischial arch
6 months
12 months
Iliac crest
16 weeks
1–2 years (may remain open permanently)
Femur
Proximal epiphysis (femoral head)
2–4 weeks
8–12 months
7–10 months
Greater trochanter (major)
5–8 weeks
6–11 months
7–9 months
Lesser trochanter (minor)
5–10 weeks
8–13 months
8–10 months
Distal epiphysis
2–3 weeks
8–11 months (to diaphysis)
12–18 months
Medial and lateral condyles
2–3 weeks
3 months (medial to lateral condyle)
Patella
7–9 weeks
Tibia
Lateral and medial condyles
2–3 weeks
6 weeks (medial to lateral condyle)
Tibial tuberosity
7–9 weeks
6–8 months (to condyle)
12–18 months
8–12 months (to diaphysis)
Proximal epiphysis
3–8 weeks
8–12 months
12–18 months
Distal epiphysis
2–4 weeks
8–11 months (to diaphysis)
9–12 months
Medial malleolus
11–13 weeks
5 months
Fibula
Proximal epiphysis
7–10 weeks
8–12 months
13–17 months
Distal epiphysis
4–7 weeks
7–12 months
9–13 months
Tarsus
Calcaneus
1 week
Talus
1 week
Intertarsal bones (central, I–IV)
3–4 weeks
Tuber calcis (calcanean tuber)
6–8 weeks
3–8 months
7–12 months
Tarsal bones (central, I–IV)
2–4 weeks
Sesamoids
Patella
7–9 weeks
Fabellae (stifle)
12 weeks
2–5 months
Popliteal (stifle)
12 weeks
4–5 months
Dorsal digits
20 weeks
Plantar digits
8 weeks
Phalanges
Proximal epiphysis (II–V)
6–7 months
Distal epiphysis
Orthopedic anatomic considerations
Types of bone
Shapes of bones
Bone development
Bone response to disease or injury
Patterns of bone remodeling
Patterns of periosteal response
Patterns of osteolysis
Bone production
Bone loss
Fractures
Dorsopalmar view of a normal immature dog carpus for comparison.
Type I physeal fracture involves only the physis; commonly called a “slipped physis.”
Type II physeal fracture involves the physis and the metaphysis.
Type III physeal fracture involves the physis and the epiphysis; the fracture is articular.
Type IV physeal fracture involves the physis, metaphysis, and epiphysis; the fracture is articular.
Type V physeal fracture is a compression fracture involving the entire physis.
Type VI physeal fracture is a compression fracture involving only part of the physis; growth on the damaged side may slow or stop, while the other side grows normally.
Bony bridging on one side of the physis (arrow) can restrict or stop growth on that side, similar to the Type VI physeal fracture described above.
Fracture healing
0–5 days
5–10 days
10–20 days
20–30 days
40 days (6 weeks)
Fracture complications
Physeal injuries and limb deformities
Osteomyelitis
Osseous neoplasia
Characteristic
Neoplasia
Bacterial Osteomyelitis
Mycotic Osteomyelitis
Signalment
Older, larger animals
Younger animals
Younger animals
Location
Usually metaphyseal
Often diaphyseal
Metaphyseal or epiphyseal
Distribution
Usually monostotic
Often polyostotic
Polyostotic or monostotic
Lesion size
More focal
More diffuse
More focal
Lytic or productive
Mostly osteolytic
Mostly osteoproductive
Mostly osteoproductive
Periosteal response
More irregular and less distinct
Larger, better defined
Chronic, more organized, sharper margins
Cortical destruction
May be severe
Less common
Uncommon
Codman’s triangle
More likely
Less likely
Unlikely
Rate of change in serial radiographs
More rapid change (days)
Less change (weeks)
Little change (weeks to months)
Sequestrum
Unlikely
More likely
Unlikely
Body defense
No defense
Strong defense
Variable defense
Benign conditions of bone
Congenital and developmental abnormalities
Abnormal number of bones
Chondrodysplasia
Hyperparathyroidism
Osteogenesis imperfecta
Rickets
Mucopolysaccharidosis
Canine leukocyte adhesion disorder (CLAD)
Asynchronous growth and limb deformity
Hypertrophic osteodystrophy (HOD)
Hypertrophic osteopathy (HO)
Retained cartilage core (distal ulna)
Panosteitis
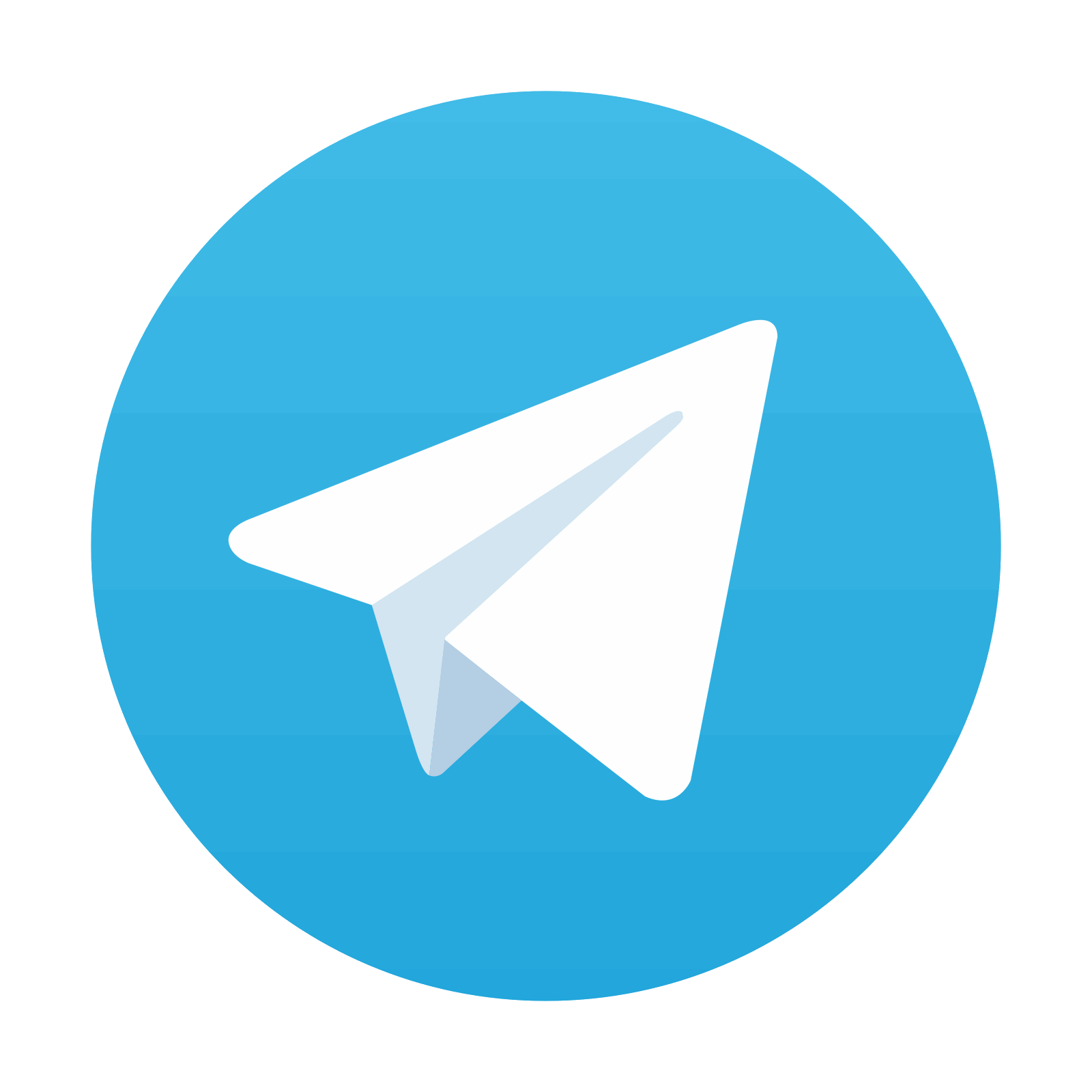
Stay updated, free articles. Join our Telegram channel

Full access? Get Clinical Tree
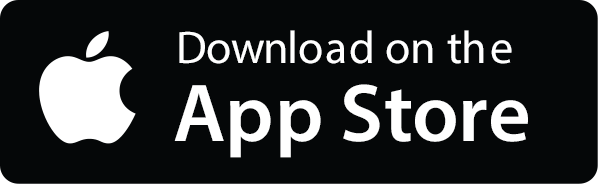
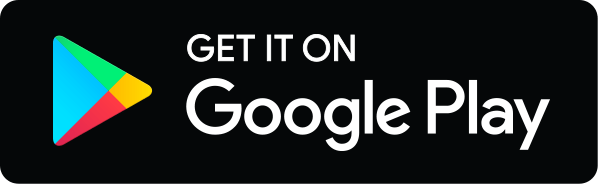