13.1 Background and development
The mouse musculoskeletal system is fundamentally similar to that of other mammals with variations of the skeleton to accommodate rodent-specific features such as the head adaptations to the continuously growing incisors and the large olfactory system. Musculoskeletal tissues arise from mesoderm, with the bone and tendon arising from the lateral plate mesoderm and the muscles from the somite mesoderm. Mineralization of bones occurs by ossification of a cartilage template in limb bones (endochondral) or direct ossification of a fibrous stroma (intramembranous) in the flat bones of the skull. Ossification starts around embryonic day 14 in the forelimb long bones and continues into postnatal life (Patton and Kaufman 1995) with most bones having ossification centres by postnatal day 7, with some exceptions such as the patella.
13.2 Sampling techniques
Routine screening of mice should include examination of the musculoskeletal system. A range of nonhistological techniques including micro- CT, X-ray analysis, DEXA analysis of bone mineral density, biochemical analysis for mineral metabolism, breaking strength testing and muscle biomarkers can be used to screen for, or investigate, suspected musculoskeletal phenotypes (Bassett et al. 2012). Differential staining of whole foetuses with Alizarin red for bone and Alcian blue for cartilage can be used to map alterations in early bone development (McLeod 1980). Behavioural observations such as gait analysis and grip strength may also be made, particularly when muscle phenotypes are suspected.
Where no musculoskeletal phenotype is expected, standardized sections of a selected long bone including a joint and an example of skeletal muscle may be sufficient for a phenotypic histopathological screen. The femur and stifle joint along with hind limb muscles, for example the quadriceps, biceps femoris or gastrocnemius are commonly sampled in routine protocols.
Bone and skeletal muscle may also be evaluated incidentally in vertebral sections if the spinal cord is processed within the spinal column and in sections of sternum, which may be taken primarily for examination of bone marrow.
Formalin or paraformaldehyde are adequate fixatives for routine examination of bone and muscle. For investigations of muscle fibre typing, using histochemical analysis or muscle diameter morphometry, frozen tissue is usually required (Wang and Kernell 2001; Briguet et al. 2004). Samples containing bone generally require decalcification prior to processing and embedding in paraffin wax. Decalcification can be performed in mice using EDTA or formic acid for 14 or 2–3 days respectively. In some circumstances, for example to evaluate mineral density, it may be helpful to examine nondecalcified bone. Nondecalcified bone can be examined using frozen sections or sections made from tissue embedded in resin. Both approaches may be technically demanding and require nonroutine histological equipment (Fuchs et al. 2006).
A longitudinal section through the femur, stifle joint and proximal tibia is commonly used for evaluation of bone, growth plate, articular cartilage and bone marrow (Morawietz et al. 2004). Coronal sections through the stifle joint where the patella is embedded face down may be useful for a more detailed assessment and scoring of damage to cartilage in the stifle joint (van Valburg et al. 1996). Scoring systems for damage to the articular surface have been developed for mice (Glasson et al. 2010; McNulty et al. 2011) adapted from the Mankin system widely used to assess human joint tissue (Mankin et al. 1971).
For routine evaluation of skeletal muscle, standardized sections of the biceps femoris muscle may be trimmed in longitudinal and or transverse orientation. Longitudinal sections are useful to assess striation but transverse sections are generally more useful for observation of fibre diameter, variation in staining, vacuolation and location of nuclei (Morawietz et al. 2004). Other muscles may be examined for specific purposes, for example, diaphragm and intercostal muscles are in constant motion and may be particularly sensitive to myotoxic agents. Other muscles consist of predominantly fast twitch fibres e.g. tibialis anterior or slow twitch fibre e.g. soleus and so may be examined if specific effects are expected in different fibre types (Augusto et al. 2004).
13.3 Anatomy and histology
13.3.1 Bone
The histology of the standard section of femur and stifle joint and skeletal muscle will be described with specific features relevant to standard sections of sternum and vertebrae. Descriptions of variations for individual bones or muscles are beyond the scope of this text. Long bones in mice, as in other species, consist of the epiphysis at the ends separated by the metaphysis from the shaft or diaphysis.
Examination of the bones involves an awareness of the normal features of the articular cartilage, cortical and medullary bone and growth plates of mice. The cortical bone in mature animals consists of compact bone, which is composed of mature bone cells (osteocytes) embedded in a mineralized, collagen matrix (Figure 13.1). The collagen fibres in mature compact (lamellar) bone are arranged in organized parallel pattern whereas in developing or repairing bone (woven) the collagen fibres are arranged haphazardly, which can be seen using polarizing light illumination (Figure 13.2). Osteoblasts, which are involved in the formation of bone, are plump cells with basophilic cytoplasm, which can be seen lining the medullary surface of the bone (Figure 13.3) and large, multinucleated osteoclasts, which are responsible for remodelling and removing bone, can be found lining trabeculae and are particularly numerous where the primary spongiosa is being remodelled (Figure 13.3).
Figure 13.1 Mature compact bone from the femur showing osteocytes embedded in collagen matrix. Also shown is a nutrient artery (arrow) and bone marrow (top right).

The thickness of the articular cartilage in mice varies depending on the joint, but is generally 10 to 15 cells thick. The cartilage consists of four distinct layers of chondrocytes in lacunae embedded in an homogenous, pale eosinophilic collagen and proteoglycan matrix (Hughes et al. 2005). The surface or articular layer is two to three cells thick and the nuclei are flattened and parallel with the articular surface. In the intermediate or transitional layer the cells are rounder, often in pairs, and align at an angle to the articular surface (Figure 13.4). The deep or radial layer is the thickest layer of the mouse articular cartilage, the cells are larger and may be present in small clumps aligned perpendicular to the surface. In the deepest layer adjacent to the subchondral bone, the matrix becomes calcified. Haematoxylin and eosin is an adequate stain for routine assessment and will allow identification of most changes e.g. cartilage necrosis and fibrillation. Cationic stains such as Toluidine Blue and Safranine-O that bind to proteoglycans can be useful in assessing more subtle damage in cartilage. Safranin-O, usually used with a Fast Green counterstain, stains glycosaminoglycans red and Toluidine Blue stains them dark blue or purple (Figure 13.5). Loss of this staining reaction indicates a decrease in glycosaminoglycans.
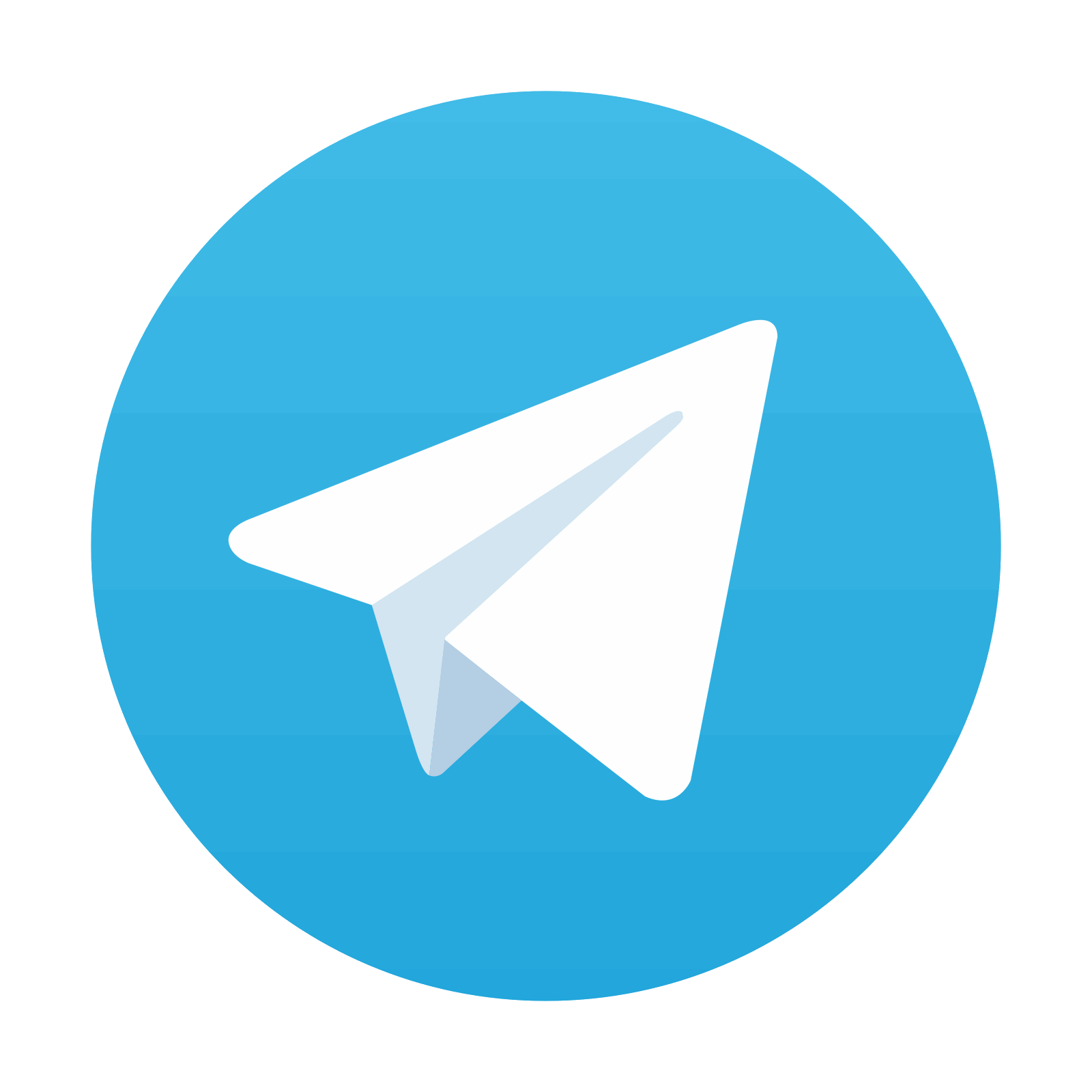
Stay updated, free articles. Join our Telegram channel

Full access? Get Clinical Tree
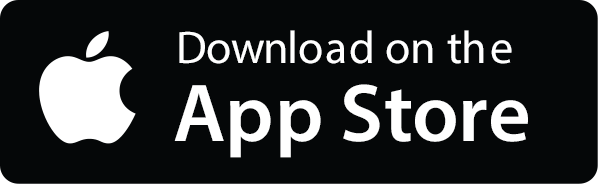
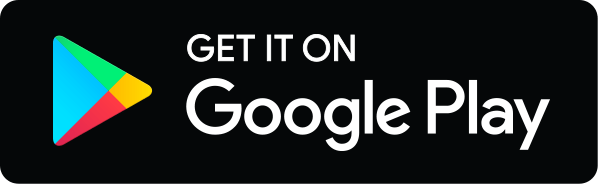