, Monika Hassel2 and Maura Grealy3
(1)
Centre of Organismal Studies, University of Heidelberg, Heidelberg, Germany
(2)
Spezielle Zoologie, Universität Marburg FB Biologie, Marburg, Germany
(3)
Pharmacology and Therapeutics, National University of Ireland Galway, Galway, Ireland
14.1 Active Cell Movement and Migration
14.1.1 Unlike in Plants Active Cell Movement Plays an Important Role in the Development of Animals
As a result of cell division and cell differentiation a large variety of cells with different shapes and divergent molecular constitutions and makeup appear. These cells in turn create associations of cells serving a common function. Such morphological and functional units are called a tissue if they are composed of one or a few cell types; they are called an organ if they are composed of diverse cell types and their functional role in the commission of the whole organism occupies centre stage. In cell associations, the number, size and shape of individual cells will eventually determine the shape of the whole association. In contrast to the development of plants, in animals active shaping through intracellular contractile filaments and intercellular forces of cohesion and adhesion are observed, and extensive displacement and migration of cells take place.
Active deformation is brought about by
intracellular, in their length variable elements of the cytoskeleton (microtubules, intermediate filaments, actin filaments),
intracellular motor proteins such as actin/myosin, kinesin, dynein and dynamin, and by
self-regulated, variable forces of cohesion and adhesion which occur between the cells and their environment.
Furthermore in animal development extensive migration of mobile cells is common.
14.1.2 Displacement and Migration of Cells Enable Construction of Tissues and Organs at Distant Locations: A First Overview
Gastrulation, a most important event in animal development, consists of the displacement of cohorts of cells into the interior of the embryo where they will give rise to the inner organs. In the amphibian gastrula displacement is brought about by the combined effect of two processes, in the initial curving in of the outer cell sheet to form the blastopore and in the subsequent streaming of cell cohorts through the blastopore. When isolated by excision cells around the blastopore crawl on a suitable substrate like a caravan of migrating Dictyostelium amoebae. Moreover, cells can sort themselves out from their association and acquire the freedom of individual mobility. Active movement of cells into the interior is the main modus of gastrulation in fish, birds, mammals, but also in several invertebrates.
In sea urchins gastrulation begins at the vegetative pole with the immigration of cells into the cavity of the blastocoel. Descendants of the micromeres untie the bonds to their neighbours, pull out of the society of the epithelial blastoderm (dissociate), and immigrate into the blastocoel. The descendants of the micromeres reaggregate at certain places and collectively manufacture the larval skeleton (see Fig. 4.2). In the gastrula of sea urchins and in the amphibian gastrula at the tip of the archenteron, there are cells that retain contact to epithelial sheets but extend pseudopodia and take on the function of pathfinders. In amphibians these cells are known as bottle cells (Fig. 14.1). Guided by these bottle cells in the course of gastrulation the roof of the archenteron slides along the overlying ectoderm (inducing it to form the central nervous system). A layer of fibronectin covering the inner surface of the ectoderm serves as lubricant.
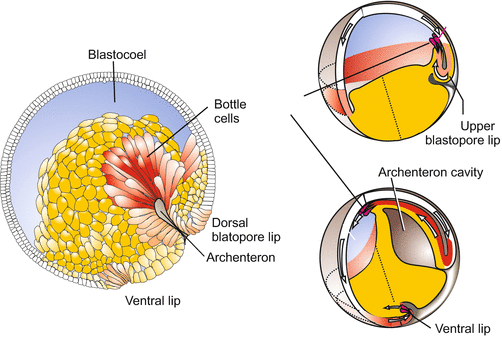
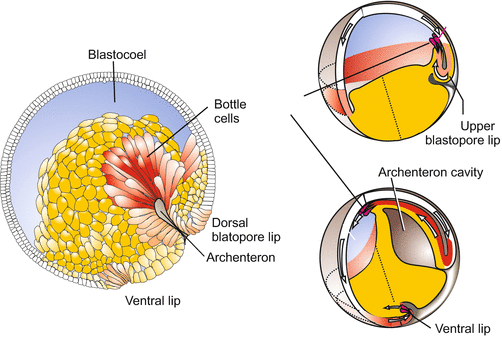
Fig. 14.1
Amoeboid bottle cells in the amphibian gastrula
In translucent embryos many freely wandering cells can be seen, such as the deep cells in fish embryos (see Fig. 5.22). Ameboid behaviour is also displayed by cells in the blastodisc of birds (Fig. 5.25) or mammals (Figs. 5.29, 6.8) that invade through the primitive groove into the depths and spread in the blastocoel (subgerminal cavity) to form the axial mesoderm (notochord and somites).
14.2 Sliding and Sorting by Virtue of Cell Adhesion
14.2.1 Convergent Extension: Stretching and Elongation of a Cell Association on the Zipper Model
When in the amphibian gastrula the future mesoderm (marginal zone) rolls around the lip of the blastopore it stretches itself, and continues elongating while gliding along the inside of the ectoderm in an anterior direction. The analysis of each single cell movement revealed an unexpected mechanism of stretching and elongation. Imagine the following situation: Two roads converge and join forming a single major traffic pathway. In addition two roads converge from higher levels, one road entering from the left, the other from the right. Of course, at the site of convergence a traffic jam happens. How is the problem solved in such a way that everybody gets a chance to line up, without traffic lights? The solution is the zipper mode, one car from the left, the next car from the right and alternating so forth.
The multilayered cell association acts in a similar way. The cells line up coming in alternate order from right or left, one by one, according to the zipper mode, so that a single layered, narrow association is formed (Fig. 14.2). In the terminology of developmental biologists the procedure is known as convergent extension. This mode of stretching is used in other processes as well. The archenteron of the sea urchin comprises initially 20–30 cells in its circumference, after its stretching it merely consists of 6–8 cells. In the process of intercalation changing forces of cell adhesion are of decisive significance.
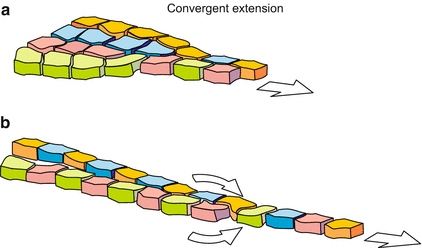
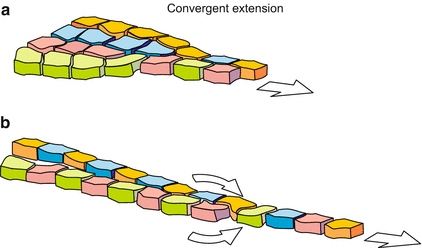
Fig. 14.2
Convergent extension and zipper procedure in the gastrulating Xenopus embryo
14.2.2 Forces of Adhesion Can Shift the Relative Positions of Cells
A drop of oil contracts on a hydrophilic substrate to form a sphere, while it spreads on a hydrophobic (lipophilic) surface to form an extended film. A drop of water displays the opposite behaviour. Similarly, adhesion or repulsion of cells is influenced by physical forces such as tension of wetting, which is the integral result of several surface energies. In addition, electrostatic and ion exchange properties influence the strength of adhesion or repulsion. Cells can alter the degree of hydrophobic or hydrophilic interactions with neighbouring cells, and the strength of electrostatic attraction or repulsion. They can do this, for example, by inserting membrane proteins and by glycosylating or deglycosylating surface proteins. Physical forces acting at interfaces are of significance when in the course of gastrulation sheets of endoderm, mesoderm and ectoderm slide along each other. Even if cells do not actively move, cell sheets can slide and spread over other sheets by sheer physical forces of adhesion and cohesion, thereby minimizing the strength of surface tensions. Minimizing surface energies also accounts for, or contributes to, the process of sorting out of cells of different origin in aggregates (Fig. 14.3).
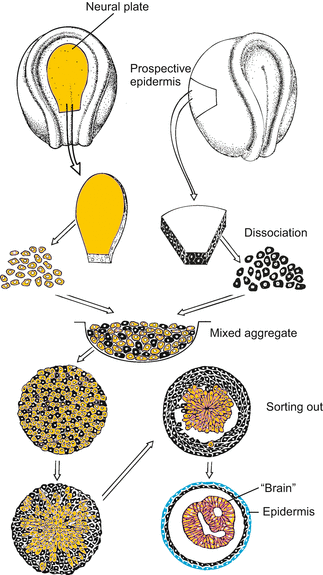
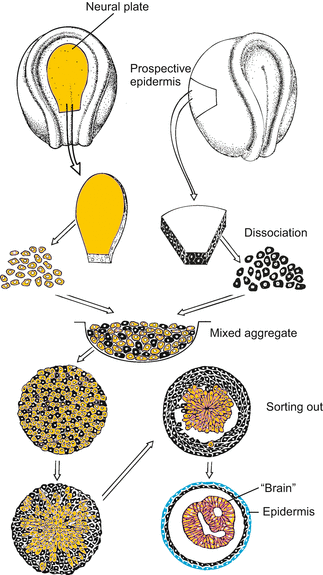
Fig. 14.3
Sorting out of cells of different origin and fate. After segregation, prospective epidermal cells form an outer epithelial layer, and the cells derived from the neural plate form hollow bodies reminiscent of neural tube or brain, respectively (after Townes and Holtfreter 1955)
During embryonic development of the vertebrates, coherent groups of cells stream to their destinations by liquid-like spreading movements. According to the “differential adhesion hypothesis”, these movements are driven and guided by cell-adhesion-generated tissue surface tensions, operating in the same manner as surface tensions do in the mutual spreading behaviour of immiscible liquids, among which the liquid of lower surface tension is always the one that spreads over its partner. Miniature devices have been designed to measure surface tensions of cell groups. The measured values correlate with the behaviour of the cells in aggregates. Cells displaying high tensions are enveloped by cells displaying lower surface tensions. For example, limb bud mesoderm (tension 20.1 dyne/cm) is enveloped by liver (4.6 dyne/cm) which, in turn, is enveloped by neural retina (1.6 dyne/cm).
In addition cells can segregate by differential mobility. For example, in an aggregate of Hydra cells (Fig. 4.14) endodermal cells migrate actively and rapidly into the interior of the cell clump, while ectodermal cells hover almost immobile at the surface and eventually reorganize an outer epithelium.
In animal embryos the differential spreading of one cell population over the surface of another is a common means of embryonic morphogenesis.
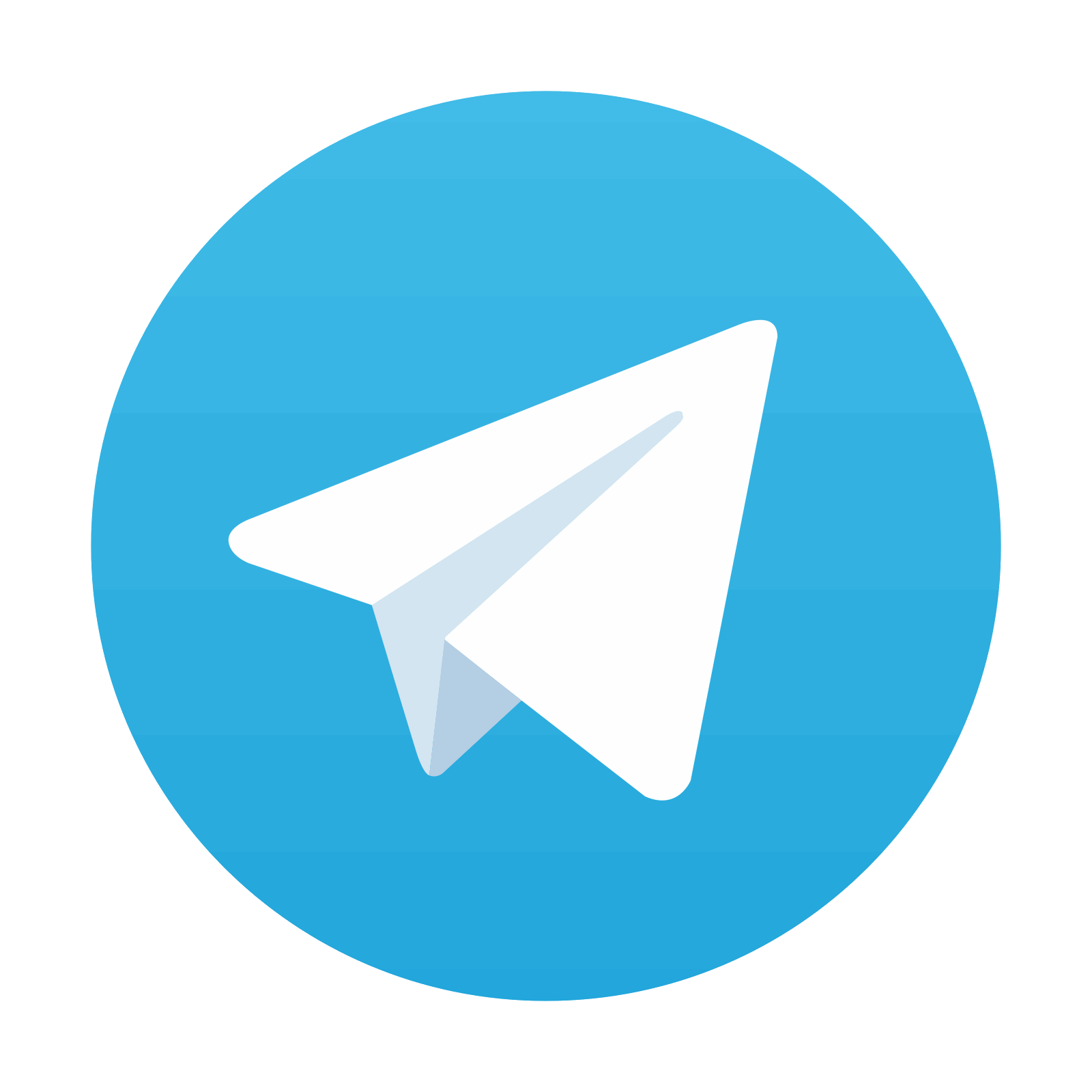
Stay updated, free articles. Join our Telegram channel

Full access? Get Clinical Tree
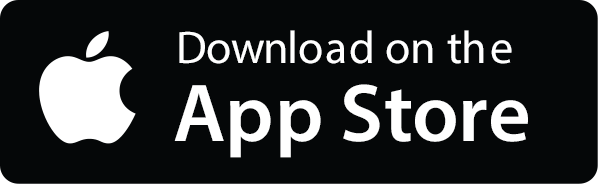
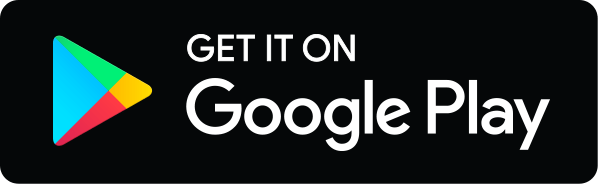