Factors that influence accuracy
Like many monitoring techniques used today pulse oximetry is also susceptible to inaccuracies. There are intrinsic and extrinsic factors that can provide inaccurate saturation data. Intrinsic factors include intravascular dyes (methylene blue) and dysfunctional hemoglobin (hemoglobin that does not carry oxygen, such as that bound to carbon monoxide, called carboxyhemoglobin). Extrinsic factors include ambient lights or heat lamps, use of electrocautery units, and more commonly patient movement or motion. Sources of ambient light can cause failure of the pulse oximeter to produce data or display erroneous SpO2 readings. If possible, shielding the pulse oximeter probe (with dark material) from light sources may help eliminate inaccuracies. During measurement patients need to remain still because most of the currently used pulse oximeter probes are sensitive to pulsating motion. Movement may affect the unit’s ability to detect a pulse as well as differentiate between movement and normal arterial pulsations. If the pulse oximeter does not detect a pulse at all or senses motion artifacts, it may audibly alarm and display an erratic heart rate. When the unit detects a pulse and displays a heart rate that matches the patient’s actual heart rate you can be assured the pulse oximeter is accurately detecting a pulse. Proper fitting and size-appropriate probes may help to decrease motion artifacts. Improving cardiac output during low perfusion states may also help decrease inaccuracies due to patient motion (Wright and Hellyer 1996; Grubb 2002), as will using pulse oximeters that are motion insensitive (such as many of those made by Massimo Corporation).
Interpretation of data
Pulse oximeters are known to be accurate in the 80–100% saturation range within 2–6%. At an oxygen hemoglobin saturation of greater than 90%, pulse oximetry saturation (SpO2) tends to be slightly lower than actual arterial oxygen hemoglobin saturation (SaO2). With saturations of less than 70%, SpO2 tends to be higher than SaO2. As SaO2 continues to decrease, the accuracy of SpO2 may decrease. The pulse oximeter probe must be in place for a minimum of 30 seconds before it will read and display an SpO2 value. The displayed pulse rate must match the palpable pulse rate (except in horses in which the pulse rate may double on the unit as a result of the large dicrotic notch), and the waveform should be consistent and smooth for the reading to be considered acceptable (Wright and Hellyer 1996; Grubb 2002).
Pulse oximetry data should be interpreted in conjunction with the status of the entire animal as well as other monitored patient parameters. For example, anemia may cause a low SpO2 reading despite a normal PaO2, and shocky animals may have poor perfusion, which may cause a falsely low SpO2. If the animal is breathing 100% oxygen, saturation should be maintained between 95–100%. Rapid response by the anesthetist is required when the saturation is less than 90% in the presence of a pulsating and strong signal. This can indicate severe shock, anemia, or significant desaturation and hypoxemia. The first response to a low saturation is to ensure that ventilation is adequate. Evaluate respiratory rate (RR) and tidal volume, and ensure a patent airway. Check that there are no interruptions of the flow of oxygen and that the nitrous oxide flow, if being used, is not in excess of 2/3 of the total fresh gas flow. To improve or treat a low SpO2 the anesthetist can institute mechanical or intermittent positive pressure ventilation, provide oxygen supplementation, correct ventilation-perfusion mismatches (by changing patient position), and administer diuretics if indicated. When high inspired oxygen concentrations are being used it is important to note that normal oxygen saturation is often achieved in a hypoventilating patient. SpO2 may not warn the anesthetist that the patient has extreme and non-physiologic elevations in PaCO2. A pulse oximeter is not a reliable indicator for hypercapnia or hypoventilation. Therefore, the adequacy of ventilation and the adequacy of saturation should be assessed separately (Wright and Hellyer 1996; Grubb 2002).
Patient assessment/treatment of decreased SpO2
If ventilation and arterial oxygenation are adequate but the SpO2 is decreasing, assess the patient’s cardiac output using some of the following indicators: capillary refill time (CRT) and mucous membrane color, pulse quality, and arterial blood pressure. An attentive anesthetist must rapidly respond to treat the problem if any of these indicators appear abnormal. Hypothermic, hypovolemic, vasoconstricted, and poorly perfused patients have decreased pulsatile flow, and this may result in a poor signal. Warming the patient, improving cardiac output first by reducing anesthetic concentrations and second by administering fluids and repositioning the sensor may help to improve the signal (Wright and Hellyer 1996; Grubb 2002). When the probe is being used on the tongue, periodic repositioning of the probe, massaging the tongue, and keeping it moist with water appear to help increase the signal (although the latter probably acts to increase perfusion at the site as well).
Conclusion
A veterinary practice is likely to purchase and use a pulse oximeter if the staff is familiar with it and comfortable recognizing and responding to abnormal values. A pulse oximeter has many advantages, and using it provides a higher level of care for veterinary patients.
Body Temperature
Heat loss is an almost inevitable consequence of anesthesia. The volatile anesthetics used in general anesthesia change the set point for thermoregulation (Harvey 2002). This change results in the patient’s inability to respond to changes in body temperature. If a response is initiated it may be ineffective at restoring normal body temperature.
Temperature normals
The normal rectal temperature in the cat is 100.5°F to 102.5°F. The normal rectal temperature in the dog is 101.0°F to 102.5°F (Eddlestone 2006).
Hypothermia
Hypothermia, decreased body temperature, is known to cause postanesthetic shivering (resulting in increased oxygen consumption), prolonged recoveries, decreased anesthetic requirements, electrocardiographic changes, coagulation deficiencies, impaired platelet function, increases in fibrinolysis, decreased activity of the pathways of coagulation, and death (Harvey 2002). Animals frequently become hypothermic during anesthesia due to the reduced heat production by skeletal muscles, open body cavities, cold intravenous fluids, cold environment (prep tables, cool air) and prolonged anesthetic periods where the shivering response is inhibited. Most healthy patients can tolerate the physiologic challenges brought on by mild to moderate hypothermia. Unlike healthy patients, a decrease in body core temperature of 2°F (1°C) can adversely affect the critical or significantly compromised patient. This patient population is of increased risk of morbidity and mortality as a result of anesthetic-induced hypothermia (Haskins 1999; Harvey 2002).
Monitoring body temperature
As mentioned earlier, hypothermia decreases the requirements for general anesthesia. For each 2°F decrease in body temperature there is a 5% decrease in the minimal alveolar concentration (MAC) of the volatile anesthetics. Hypothermia also causes a decrease in the clearance of anesthetic drugs as well as an increase in the solubility of volatile anesthetics in the body. Diligence by the anesthetist is warranted because the combined result of the processes can be a significant potential for anesthetic overdoses. Therefore, perioperative monitoring of body temperature is strongly encouraged. It is easy to do and is relatively inexpensive. The attentive anesthetist who monitors body temperature is then able to adjust vaporizer settings and anesthetic doses in order to minimize the anesthetic complications of hypothermia. A thermometer probe in the esophagus or rectum allows constant temperature measurement. Accidental placement in the stomach or oral cavity reduces accuracy of core body temperature estimate. These probes can be cleaned between patients with a diluted chlorhexidine diacetate solution and allowed to dry. Thermometers (mercury or digital) placed in the rectum for intermittent measurement can also be used. Thermometers can be cleaned with isopropyl alcohol and allowed to dry between patients. Mercury thermometers are easily broken and cleanup of the mercury is expensive and potentially dangerous, and disposal is hazardous to the environment. As a result, digital thermometers are strongly preferred (Haskins 1999; Harvey 2002).
Prevention of hypothermia
Prevention of hypothermia includes minimizing patient heat loss. The easiest thing to do to control radiant heat loss is to shield the patient from its cool environment with towels or blankets. When permitted, place a warm blanket or towel on the cold surgical or prep table to shield the patient from its surface and/or place warm blankets or towels directly on the patient on the nonsurgical areas to help minimize convective losses. Wrapping limbs in commercial bubble wrap or placing baby booties on the paws of the smaller patients seems to help in the prevention of heat loss. When possible the use of low flow gas techniques is also helpful. Keep prep time to a minimum and warm the antiseptic preparations used if at all possible. Minimizing surgical time will also help in preventing hypothermia but may not always be feasible (Haskins 1999; Harvey 2002).
Active patient warming
There are several active warming strategies that can be used during the patient’s perioperative period to maintain or restore body temperature. Turning up the heat in the operating suites as well as the recovery areas is recommended. Employing the use of circulating warm water blankets, not electric heating pads, as well as use of convective warm air devices are suggested. Electric heating pads are not recommended due to the very high temperatures reached, which increases the risk of iatrogenic thermal injuries. Tissue burns may been seen on patients warmed with electric heating pads due to the uneven distribution of heat and their inability to recognize excessive heat and remove themselves from the source. These pads are designed to be used on human patients who are conscious and able to remove the pad when they feel overheated. In addition, use of hot water bottles and gloves filled with warm or hot water is also dangerous for the anesthetized, comatose, or sedated patient. Thermal burns may be caused, and as these devices continue to cool, they contribute to the loss of body heat. In contrast, the circulating warm water blankets are less likely to cause thermal burns and can be used to prevent or minimize hypothermia when used correctly. Covering the patient’s cage floor with half of the warm water blanket allows the patient to move away from the heat source, when able, to prevent injury (Haskins 1999; Harvey 2002).
Convective warm air devices are very effective in preventing and treating hypothermia. Units such as the Bair Hugger® by Gaymar Industries, blow warm air through porous blankets to surround the patient in warm air (Harvey 2002). There are many sizes and configurations of blankets on today’s market. Use the best one to fit your patient to maximize the heat available to them. The Hot Dog Warming System® by Augustine Biomedical + Design features reusable, easy to clean, heavyweight nylon outer shell blankets that are flexible so they can be used around, under, or over the patient. Any of these blankets can also be covered with commercial bubble wrap or blankets and towels to help prevent the loss of heat. Body temperature must be monitored while using these units as it may increase rapidly. Use the blower units in the operating suites with caution as they work by “blowing” warm air and may blow debris and hair onto sterile fields, thereby contaminating various surfaces. If convective warm air devices are allowed in the operating suite, the anesthetist can decrease contamination risk by waiting until the patient is completely draped in before turning the unit on.
Warmed intravenous fluids or irrigation fluids must be used with caution. Overzealous warming of these fluids can be damaging to tissues. Fluid line warmers are available and act by heating the IV fluids as they run through the drip set. These may work better to control overheating of fluids but testing the temperature of the fluid first on one’s wrist is recommended. Depending on the fluid drip rate and where the warmer is positioned on the drip set, fluids can again cool off before reaching the patient. Radiant heat via a heat lamp above the patient and the surgical field can also damage tissues when used carelessly in operating suites and recovery areas. Radiant heat sources can overheat tissues and burn through oxygen hoses and breathing circuits when not used properly. Temperature monitoring is again mandatory to decrease the risk of thermal injury when using active heating systems. Whatever active heating methods are employed, they should be started early to prevent hypothermia and their use should be controlled. Monitoring of patient body temperature should be mandatory (Haskins 1999; Harvey 2002).
Hyperthermia
Hyperthermia, increased body temperature, may be an iatrogenically induced response to specific drug combinations in certain species (cats) or with overzealous use of heating devices (e.g., circulating hot water blanket) in an effort to prevent the animal from cooling. Disruption of the normal processes for heat loss may also cause abnormal increases in body temperature due to anesthesia. Particularly in the small animal patient, increased body temperature is a less common sequela of general anesthesia and surgery but nonetheless is important to watch for (Haskins 1999; Harvey 2002).
Causes of hyperthermia
Patients’ body temperatures may rise to above normal levels during anesthetic events. Decreased loss of body heat due to increased insulation (e.g., blankets, insulating hair coats), excessive or poorly monitored active heating devices, and increased metabolic production of heat (e.g., increased muscle tone) are mechanisms that may contribute to hyperthermia. Adverse effects of hyperthermia include increased metabolic rate, consumption of energy, and an increase in oxygen utilization, which may result in cellular hypoxia. Potential brain, kidney, liver, and blood damage may occur. Mild to moderate hyperthermia requires immediate intervention by the anesthetist. Active cooling of the patient and organ function monitoring are required to identify any complications from the hyperthermic event (Haskins 1999; Harvey 2002).
Treatment of hyperthermia
The underlying cause or causes should be identified and corrected during moderate hyperthermic events. Usually external active warming devices are to blame, but not always. Remove all external warming devices and begin to cool the patient immediately. Increasing oxygen flow can help cool the patient and prevent rebreathing of carbon dioxide and its retention. The application of cool water or alcohol to the footpads and skin may help to increase evaporative cooling. Judicious use of cold packs while avoiding tissue damage may help increase conductive heat loss. Cool intravenous fluids help to increase peripheral perfusion and heat loss. Cool enemas, cool abdominal lavage, and cool water submersion are some more aggressive methods of cooling. Whatever methods for cooling are employed, body temperature must be monitored continuously in order to remove the cooling methods before the return of normal body temperature. It may be difficult to anticipate the continued drop of body temperature once cooling methods are removed. Therefore, continued monitoring of temperature and evaluation of organ function for signs of compromise or failure is mandatory. Any adverse sequelae should be identified and treated appropriately (Haskins 1999; Harvey 2002).
Malignant hyperthermia
True malignant hyperthermia (MH) syndromes are rare but have been reported in humans, pigs, and dogs. MH is based on an abnormal release of calcium from the sarcoplasmic reticulum as well as abnormal calcium function. This excess concentration of calcium in the myoplasm causes extraordinary catabolic anaerobic and anaerobic metabolic reactions and is thought to be the functional defect in MH. In MH, patients may develop a syndrome of progressive and possibly fatal increased body temperature that is “triggered” by general anesthetics, particularly the volatile agents and succinocholine. Signs of MH include rapid increases in body temperature, increased end-tidal carbon dioxide levels in canines, and acidosis and muscle rigidity in porcines and humans. Fatalities may result from cardiac arrest, brain damage, failure of other body systems, and internal hemorrhage (Haskins 1999; Harvey 2002).
Rapid and aggressive response by the anesthetist is required in suspect cases of MH. Removal of the triggering agent, active cooling, intravenous fluid therapy, treatment for hypoxia, management of acidosis, and administration of dantrolene sodium, if possible, are imperative. Dantrolene is expensive and the results are variable in animal patients. Dantrolene is seen as a lifesaving component in human medicine and works by preventing the ongoing release of calcium from the sarcoplasmic reticulum storage sites in muscle tissue. Whatever therapies are initiated, intensive patient monitoring is required (Haskins 1999; Harvey 2002).
Conclusion
Monitoring body temperature during anesthesia and the postoperative period is recommended. Despite the fact that hypothermia is almost inevitable during anesthesia, there are many methods the anesthetist may employ to limit the loss of heat and/or to safely and actively warm the patient. Monitoring body temperature may also alert the anesthetist to an increase in body temperature, hyperthermia from overzealous active heating methods, or hyperthermia from the rare, but life-threatening, malignant hyperthermia syndrome.
Hands-on Monitoring
Anesthetic depth
The depth of anesthesia can be indirectly assessed by the position of the eye and the degree of depression of the eye’s protective reflexes. During a surgical plane of anesthesia, the eyes roll ventrally in most species (Fig. 10.2). The eye is centered in the globe during light and deep planes of anesthesia. Dissociative anesthetics (e.g., ketamine) keep the eye centered in the globe during all planes of anesthesia (Haskins 1999).
The palpebral reflex is absent in most species during a deeper level of surgical anesthesia (Haskins 1999). In small animals it is elicited by tapping the eyelids or medial/lateral canthus.
The corneal reflex should always be present during anesthesia. Since this reflex is lost relatively late and there is risk in causing trauma to the cornea, this reflex should never be used in an animal unless confirming complete cardiovascular arrest.
Figure 10.2. The canine eye rotates ventrally and medially during light planes of anesthesia.

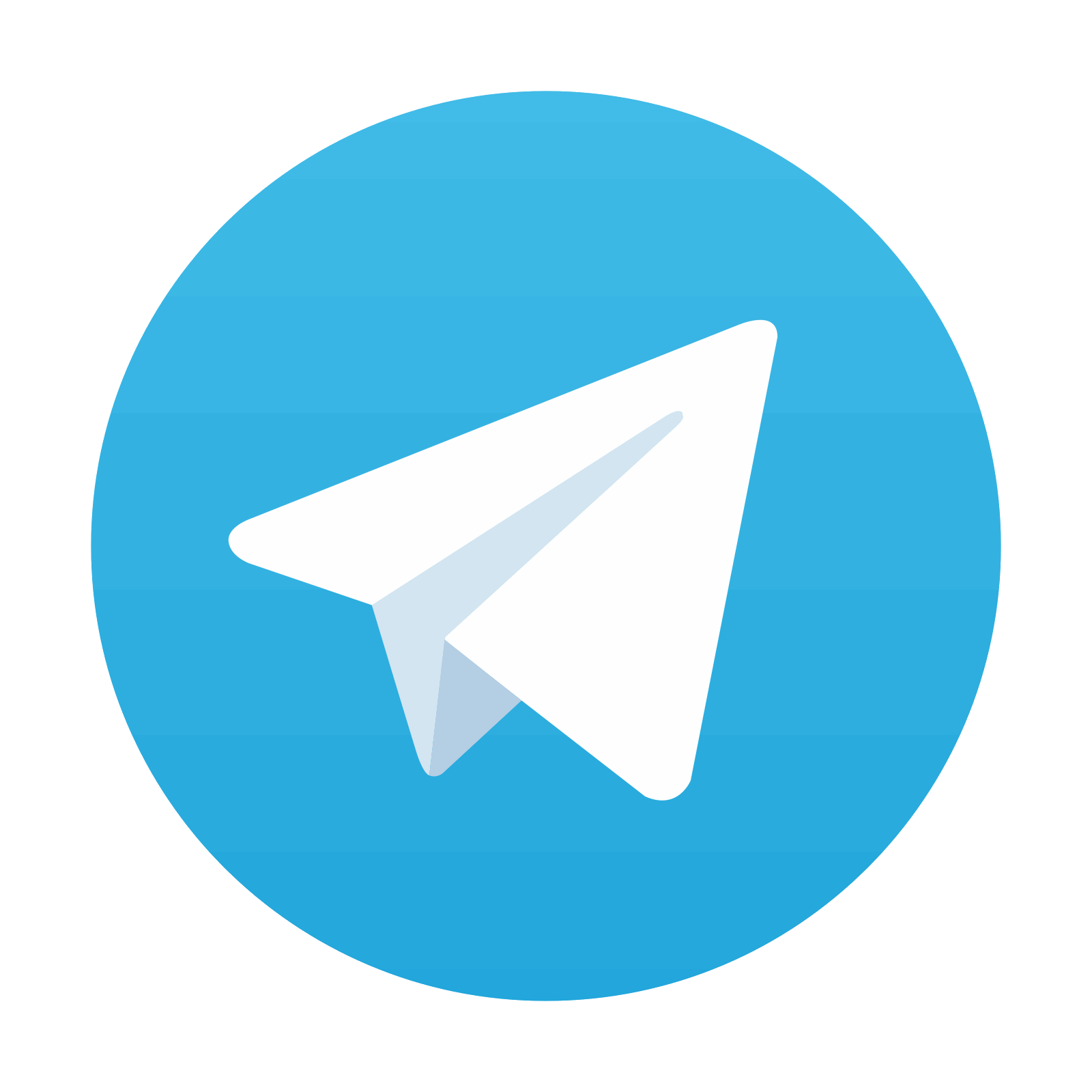
Stay updated, free articles. Join our Telegram channel

Full access? Get Clinical Tree
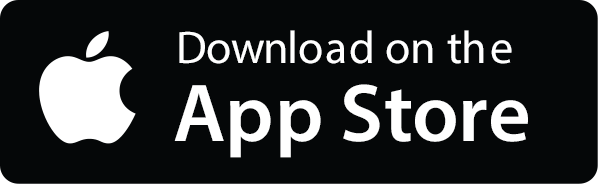
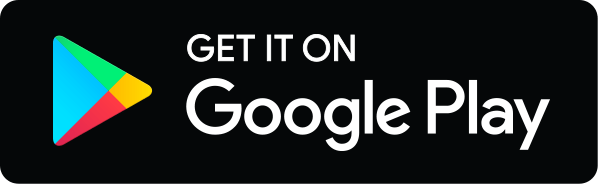