Technique
Sex
Significance
Example studies
Captive studies
F
Longitudinal monitoring, estrous cycling, gestational changes, growth rate
M
Longitudinal monitoring, sexual maturity and activity, ejaculate characteristics, growth rate
Carcass dissection and histology, immunohistology
F
Ovarian follicular activity, previous ovulations and birth, absolute age
M
Testicular development, stage of spermatogenesis, absolute age
Direct observation
M/F
Mating behavior, social associations, calf abundance, length of nursing, intercalving period, growth and maturity rates
Sexual dimorphism
M/F
Mating system, indicator of maturity
Body scarring
M/F
Aggressive social interactions: sexual coercion, male-male competition, energetics
Endocrinology
F
Estrous cycling, pregnancy diagnosis, lactation, fertility potential
M
Sexual maturity and activity, mating behaviour, development of secondary sexual characters, fertility potential
Ultrasound
F
Reproductive tract activity, fetal development
M
Gonadal activity
aBrook et al. (2000)
Spermatozoa analysis
M
Sperm morphology and morphometrics, ultrastructure, phylogenetic comparisons
Gamete cryopreservation
F
Oocyte preservation
M
Sex sorting, artificial insemination (AI)
2 State of the Art: How to Study Reproduction in Fully Marine Mammals
2.1 Captive Fully-Marine Mammals
Marine mammals in captivity provide a unique opportunity to investigate species-specific behavior (e.g., vocalizations: Edds et al. 1993; Vergara et al. 2010; Ridgway et al. 2012; cognitive function: Mercado and deLong 2010; post-natal development: Lyamin et al. 2005; Favaro et al. 2013) and physiology (e.g., dive response: Kooyman et al. 1981) that normally would be challenging to study in the wild. Extrapolation of information gained from captive individuals to wild populations can be complicated (see Lambrechts et al. 1999; Jenssen et al. 2001), because extended periods spent in artificial environment may induce variation in physiological traits (e.g., Mellish et al. 2006), including reproduction. Nonetheless, significant insights into reproductive cycles of marine mammals have been made through captive breeding programs, where individuals’ reproductive status have been monitored in longitudinal studies of behavior and internal physiological state (e.g., Atkinson 1997; Boyd et al. 1999; Robeck et al. 2001).
Captive marine mammals for which reproductive parameters have been obtained, including within artificial breeding programs, comprise delphinids (dolphins, killer whales, false killer whales; e.g., O’Brien and Robeck 2012a; Robeck and Monfort 2006; Atkinson et al. 1999), monodontids (beluga; e.g., Steinman et al. 2012), sirenians (manatees, dugongs; e.g., Amaral et al. 2009; Burgess et al. 2013a) and pinnipeds (seals, sea lions, walrus; e.g., Atkinson and Gilmartin 1992; Myers et al. 2010; Kinoshita et al. 2012). Due to limitations of space and provision of adequate nutrition, marine mammals housed in zoological settings are typically small (<10 m long) and mostly tractable. Larger marine mammals, such as all mysticete cetaceans (Edds et al. 1993; Sumich et al. 2001), have not been held in captivity past reproductive age. A number of factors may affect the quality and robustness of reproductive data obtained from captive animals. First, numbers of captive individuals are usually small. Second, individuals are often segregated by sex and age so that natural social groupings may be fragmented or nonexistent. Furthermore, the effects of highly artificial physical surroundings on an animal’s reproductive behavior and functioning are not well understood.
Despite these limitations, observations of mating and subsequent parturition in captive animals have enabled at least initial estimates of age at maturity, reproductive seasonality, and length of gestation for many smaller species (McBride and Hebb 1948; McBride and Kritzler 1951; Essapian 1962; Cornell et al. 1987; Odell et al. 1995; Blanchet et al. 2006). More recently, training programs using operant conditioning along with advanced monitoring technologies (see Sects. 2.4 and 2.5) have enabled collection of serial biological samples and/or physical examinations under temporal regimes that are adequate to characterize reproductive cycles in live females and males (reviewed by O’Brien and Robeck 2012b).
A great advantage of captive marine mammals is that most can be trained to present voluntarily for collections of body fluids including blood (Robeck and Monfort 2006; Bauer et al. 2010), saliva (Pietraszek and Atkinson 1994; Hogg et al. 2005), respiratory mucus (Hogg et al. 2005), ocular secretions (Amaral et al. 2009), urine (Walker et al. 1988; Robeck et al. 1993, 2005a; Wakai et al. 2002), feces (Biancani et al. 2009), and milk (West et al. 2000), all of which may be useful for longitudinal monitoring of hormone profiles. The relatively non-invasive techniques of fecal sampling (and urinary sampling with training) can be conducted daily and offer the best prospect for examining endocrine-reproductive relationships. Such investigations of reproductive cycling have been enhanced by monitoring body temperature (Terasawa et al. 1999; Katsumata et al. 2006a, b), examining the reproductive tract using trans-abdominal ultrasound (Brook et al. 2000, 2001, 2004; Lacave et al. 2004), conducting vaginal cytology of females (Pietraszek and Atkinson 1994), collecting semen from males (Schroeder and Keller 1989; Robeck and Monfort 2006; O’Brien et al. 2008; Robeck et al. 2009; Yuen et al. 2009) and simultaneously documenting reproductive behavior (e.g., Tavolga and Essapian 1957; Shane et al. 1986).
Understanding the reproductive cycles of captive individuals has advanced husbandry practices including management of pregnant females and fetal health (Williamson et al. 1990; Lacave et al. 2004; Katsumata et al. 2006b), establishment of socially cohesive groupings (Shane et al. 1986; Waples and Gales 2002; O’Brien and Robeck 2006), contraception (Atkinson et al. 1993; Briggs 2000; Calle 2005) and captive breeding programs (Robeck et al. 2005b). It has enabled the development of assisted reproductive technologies including artificial insemination (Robeck et al. 2004, 2005b, 2009, 2010; O’Brien and Robeck 2006, 2010; O’Brien et al. 2008), estrous synchronization (Robeck et al. 2009), sperm sexing (O’Brien and Robeck 2006; O’Brien et al. 2009), and semen preservation (Robeck and O’Brien 2004; O’Brien et al. 2008; Yuen et al. 2009). Understanding the relationships between reproductive behavior and physiological events for marine mammals in captivity has enabled facilities to determine and/or manipulate readiness for breeding, and to ensure successful conception for several cetacean taxa (Cornell et al. 1987; Robeck et al. 2005b; O’Brien and Robeck 2012a, b). With the increasing need for aquaria to display animals born in captivity rather than wild-caught individuals, the success of captive breeding programs for marine mammals is necessary to sustain ex situ populations and manage genetic bottlenecks that can occur in reproductively-isolated facilities (reviewed by O’Brien and Robeck 2012b).
2.2 Dissection of Carcasses
Almost all of the information regarding basic reproductive patterns of wild cetaceans and sirenians has been derived from the examination of reproductive tracts recovered from carcasses obtained from hunted (e.g., Best 1969; Miyazaki 1984; Desportes et al. 1993; Dawbin 1997; Kwan 2002) or stranded animals (e.g., Calzada et al. 1996; Evans and Hindell 2004) or from those incidentally caught and killed in fishing nets (e.g., Read 1990; Van Waerebeek and Read 1994; Hohn et al. 1996) or shark nets (e.g., Marsh et al. 1984a, b; Cockcroft and Ross 1990). Early studies using post-mortem material provided information on gross morphology and anatomy of the reproductive tract (e.g., Harrison and Ridgway 1971). Functional morphology of reproductive tracts, histology of gonads and mammary glands, and embryology (van der Schoot 1995; Thewissen and Heyning 2007) have provided information on the timing of reproductive activity, conception, gestation, birth and lactation, and also information concerning age and/or body size-related life history parameters including first reproduction and numbers of offspring produced over lifetimes (e.g., cetaceans: Laws 1961; Ohsumi and Masaki 1975; Lockyer and Martin 1983; Marsh and Kasuya 1984, 1986; Kato and Sakuramoto 1991; Read and Hohn 1995; sirenians: Marsh et al. 1984a, b; Hernandez et al. 1995; Marmontel 1995; Kwan 2002). For example, sexual maturity in males can be determined through assessment of testicular development (including seminiferous tubule diameter, Sertoli and Leydig cell activity), relative weight and length of testes, stage of spermatogenesis and presence/abundance of sperm (e.g., Perrin and Henderson 1984; Perrin and Reilly 1984). More recently, immunocyto-chemical methods have been applied to examine maturation of gonads: these include screening for distribution of cytoskeletal proteins (e.g., smooth muscle actin and vimentin) as markers of testis development (Holt et al. 2004). For females, ovarian follicular activity is indicative of maturity, and previous ovulations have been confirmed by presence of corpus luteum and/or corpus albicans, since in many marine mammals, ovarian scars appear to be persistent (e.g., Marsh and Kasuya 1984; Perrin and Reilly 1984). Pregnancy rate and calving intervals can be determined though counts of placental scars (e.g., dugongs, Marsh et al. 1984a) or ovulatory scars (in cetaceans, most are thought to represent pregnancies, Boyd et al. 1999) regressed against age or body length at death. Timing of sexual activity is suggested by the presence of mature spermatozoa in males and ovarian activity in females (Perrin and Reilly 1984) and reproductive senescence through their absence (Marsh and Kasuya 1986).
Carcass analysis, for all of its value, is prone to both temporal and/or spatial biases (Boyd et al. 1999). Carcasses are usually sourced from places where hunting, harvest or other causes of mortality are most likely, or from areas where reportage or recovery of carcasses are most common (e.g., Chittleborough 1954; Marsh et al. 1984a, b; Marsh and Kasuya 1986; Desportes et al. 1993; Kwan 2002). In some cases, certain individuals or life stages are more likely to be sampled, e.g., the targeted fishery for dugongs where hunters preferentially take pregnant rather than resting females (Boyd et al. 1999); or samples obtained at certain times of the year, e.g., from baleen whales during the hunting season (Chittleborough 1954; Laws 1961). Further, the utility of a carcass depends on its degree of decomposition, so that if carcasses from warmer climates decompose faster, less information may be available for these than for animals from higher latitudes. However, the value of carcasses for studying reproduction and other facets of marine mammal biology cannot be understated. Indeed, for some rare marine mammal species, carcass recovery has been the only avenue for direct examination (e.g., Reyes et al. 1991; Thompson et al. 2012). In many cases, however, recoveries are too few to obtain useful information regarding reproductive biology. In contrast, for those species that strand en masse, recovery of multiple carcasses has yielded reproductive profiles of social groupings (e.g., Mignucci-Giannoni et al. 2000). When using carcass analysis to obtain information, there is significant value in establishing coordinated responses to marine mammal strandings (e.g., Thompson et al. 2012) at regional or national levels to ensure that, when rescue attempts fail, there is timely collection of tissues (including from reproductive tracts) and other vital data.
2.3 Direct Observations
Direct observations of reproductive activity and behavior at sea of free-ranging, fully marine mammals vary from single or opportunistic sightings (e.g., courtship: Preen 1989; birth: Stacey and Baird 1997; copulation: Adulyanukosol et al. 2007; naso-suckling: Gero and Whitehead 2007) through to longitudinal sightings (Glockner-Ferrari and Ferrari 1990; Scott et al. 1990; Whitehead 1993; Langtimm et al. 1998; Rathbun et al. 1995; Wells 2000; Rowntree et al. 2001; Burgess et al. 2012a, b). Unsurprisingly, the quality of these data varies markedly depending on approachability of the species and the observational methods applied. The observation methods should be selected carefully to meet the objectives and scale of the research project.
Distinguishing sexes of marine mammals through visual means can be challenging for species that exhibit no obvious sexual dimorphism in body size or morphology, e.g., most cetaceans and sirenians. Male marine mammals are testicond (ascrotal) with their testes permanently concealed in the abdomen (excluding otariid seals which have scrotal testes; Atkinson 1997) and the flaccid penis can be retracted into the body wall (Marsh et al. 1984b; Rommel et al. 1992) facilitating streamlining. Thus, for the majority of sexually-monomorphic species, the sex of most observed individuals remains unknown unless genetic sexing is accomplished. For most cetaceans and sirenians, mating has never been confirmed and courtship is difficult to interpret or recognize.
In fact, detailed information on patterns of courtship, mating and parturition in the wild only exists for a small number of fully-marine mammal species (e.g., Karczmarski et al. 1997; Stacey and Baird 1997; Hückstadt and Antezana 2001; Parks et al. 2007) and is mostly documented for pinnipeds that breed and give birth on land (e.g., Le Boeuf 1991; Cassini 1999; Acevedo et al. 2008; Karamanlidis et al. 2010). A general understanding of reproductive patterns for most entirely-aquatic species has largely been compiled from limited, often opportunistic, data and then analogized according to our knowledge of other mammalian species, e.g., primates (Wells 2003; Connor 2007). For some large odontocetes including beaked and bottlenose whales, their rarity, remote habitats and secretive habits ensure that they are rarely, if ever, observed alive (Heyning 1984; Thompson et al. 2012) so that their reproductive behavior remains a mystery. For many of the mysticete whales, direct observation is often fleeting and opportunistic, and rarely extensive or enlightening in terms of reproductive behavior. Exemplifying this point is the little we know about the reproductive habits of the largest animals that have ever existed, blue whales, Balaenoptera musculus (Yochem and Leatherwood 1985) despite having documented extensively various aspects of their ecology. Their elusive nature, deep-water habits and open geographic ranges (i.e., the only clear geographic boundaries to baleen whale movement are large continental land masses) have meant that breeding grounds of Southern Hemisphere populations remain unverified (Attard et al. 2012). However, for other large baleen whales (e.g., Kraus et al. 2001; Clapham 2008), some observations regarding reproductive behavior have been conducted at particular locations along predictable migration routes between feeding and breeding grounds or in breeding grounds themselves, particularly for species that travel along topographic features such as coastlines or continental shelves. Amongst the best known of the marine mammals are those that spend significant time in coastal habitats (including migratory whales, delphinids, manatees) or at known breeding aggregation points, e.g., around islands, sea mounts, reefs and along mid ocean ridges.
Direct observation methods usually include distant sightings from elevated platforms: vessels, aircraft or land-based vantage points. Even though some fully-marine mammals may be approached closely by boat at times (e.g., manatees, Hartman 1979; dugongs, Preen 1992), most of their reproductive activities probably occur underwater and are unavailable to observers, so that some inference may be required (e.g., sex of observed individuals). In contrast, indirect ‘observations’ rely on telemetry to track animals, and these methods call for capture and attachment of radio/satellite/GPS/acoustic tags or recoverable data loggers (e.g., Mate et al. 1998; Noad and Cato 2001; Deutsch et al. 2003). Information on location and movements of tagged individuals have been particularly useful for tracking breeding migrations, determining timing of reproductive cycles, or for examining smaller scale movements and social associations within breeding grounds (Mate et al. 1998, 2003; Russell et al. 2013).
Direct visual observations may also be conducted at the population level and may yield information regarding spatial distribution of animals, density and timing of breeding migrations, adult aggregations, breeding associations, and presence of neonates. In these cases, individual identity of group members is not always necessary. Examples of this approach include aerial surveys of humpback whale adults aggregating within breeding grounds (Andriolo et al. 2006), surveys of right whale nursery areas and adult aggregations (Rowntree et al. 2001; Parks et al. 2007) and calf counts for various taxa as an index of annual recruitment of breeding success (Perryman et al. 2002). Compilation of these observations over extended temporal scales gives information regarding reproductive timing and breeding patterns.
Behavioral observations are greatly enhanced through recognition of individuals. If individual animals can be distinguished and identified through discriminate marks (permanent pigmentation, dorsal fin shape and/or body scarring, genetic tags) or artificially-applied marks (physical tags), there is the opportunity for longitudinal studies of life history. Recognizable individuals allow for descriptions of basic activity patterns (resting, socializing, travelling, feeding) as well as interpretation of inter-specific behaviors, especially if sex and reproductive condition are known (e.g., Connor et al. 1992; Smolker et al. 1992; Brown et al. 1995). For example, long-term observations of known identified mother-calf pairs of Florida manatees at winter aggregation sites have provided insight into frequency of calving, intercalving period, growth rates of calves, duration of nursing, time to maturity and other reproductive measures critical to population modeling (Langtimm et al. 2004). The value of longitudinal sightings data lies not only in compilation of life history parameters relevant to reproductive biology (including reproductive and total life span if individuals are recognized from an early age), but in elucidation of social behaviors including social associations, mate selection, nurturing of young and other details about a species’ breeding behavior (Mann et al. 2000). Individual identification has become a staple of many field studies of cetaceans (reviewed by Würsig and Jefferson 1990).
Despite the technical difficulties in directly observing and identifying mammals in the pelagic environment, our understanding of reproductive behavior and social interactions is improving. New techniques that allow us to track individuals through time and space, such as the amplification of molecular markers from tissue biopsies for tagging as well as advanced telemetry and biologging (Block 2005; Bograd et al. 2010), are providing superior insights into social structure and social associations related to reproduction.
2.4 Endocrinology
Since most reproductive processes are mediated through hormones circulated in the bloodstream, and hormonal functions tend to be conserved across mammalian taxa, endocrine analysis is a useful means of assessing reproductive status (e.g., sexual maturity, pregnancy diagnosis) and reproductive activity (e.g., estrous cycles, seasonality), particularly for live individuals.
The most commonly measured hormone in marine mammals is progesterone (Table 11.2) since its diagnostic use indicates the onset of female sexual maturity or pregnancy. Understanding reproductive status of females provides vital information for the management of both wild (e.g., Gardiner et al. 1996; Atkinson et al. 1999; Rolland et al. 2005; Kellar et al. 2006; Tripp et al. 2008; Villegas-Amtmann et al. 2009; Burgess et al. 2012a) and captive populations (e.g., Pietraszek and Atkinson 1994; Atkinson et al. 1999; Biancani et al. 2009; O’Brien and Robeck 2012a). The other sex steroid hormones (Table 11.2) that are routinely measured are testosterone (Atkinson and Gilmartin 1992; Desportes et al. 1994; Kellar et al. 2009; Burgess et al. 2012b) and various forms of estrogen (e.g., Francis-Floyd et al. 1991; Pietraszek and Atkinson 1994; Robeck et al. 2005b). Gonadotropins including luteinizing hormone (LH) and follicle stimulating hormone (FSH), and other protein hormones (Table 11.2) have primarily been measured in cases of assisted reproduction (i.e., artificial insemination or gamete harvesting), often requiring multiple samples collected daily (Robeck et al. 2004, 2009; Muraco et al. 2010); although some studies exploiting opportunistic sample collection have been reported (Suzuki et al. 2001; Watanabe et al. 2004; Hao et al. 2007)
Table 11.2
Summary of endocrine glands and hormones investigated in marine mammals with referenced applications in free-ranging populations (where possible)
Gland | Hormone | Chemical class | Principal functions | Example studies |
---|---|---|---|---|
Ovary Graafian follicle | Estrogens (e.g. estradiol) | Steroid | Female mating behavior, secondary sex characteristics, maintenance of female duct system, mammary growth | |
Inhibin | Protein | Regulates release of FSH from anterior pituitary | Wetzel et al. (2009) | |
Anti-Müllerian hormone (AMH) | Protein | Sophisticated biomarker of reproductive potential | Wilson et al. (2011) | |
Ovary Corpus luteum | Progestins (e.g. progesterone) | Steroid | Maintenance of pregnancy, mammary growth and secretion | |
Relaxin | Polypeptide | Expansion of pelvis, dilation of cervix | ||
Testis Leydig cells | Androgens (e.g. testosterone) | Steroid | Male mating behavior, spermatogenesis, maintenance of male duct system and accessory glands | |
Sertoli cells | Inhibin | Protein | Regulates release of FSH | |
Anti-Müllerian hormone (AMH) | Protein | Sophisticated biomarker of reproductive potential | Wilson et al. (2011) | |
Adrenal cortex | Glucocorticoids (e.g. cortisol, corticosterone) | Steroid | Stress response, parturition induction, milk synthesis | |
Pineal gland | Melatonin | Biogenic amine | Control of seasonal reproduction | |
Posterior Pituitary | Oxytocin | Octapeptide | Parturition and milk ejection in females | |
Anterior pituitary | Follicle stimulating hormone (FSH) | Glycoprotein | Stimulate follicle growth and estrogen production in females, spermiogenesis in males | |
Luteinizing hormone (LH) | Glycoprotein | Stimulate ovulation, support corpus luteum formation and progesterone secretion, stimulate testosterone synthesis by Leydig cells of testis | ||
Prolactin | Protein | Development of mammary function, maintain lactation, effects on maternal behavior, post-partum estrous cycling | ||
Adrenocorticotrophic hormone (ACTH) | Protein | Release of corticosteroids and glucocorticoids, initiate parturition | ||
Hypothalamus | Gonadotrophic releasing hormone (GnRH) | Decapeptide | Stimulate release of FSH and LH from anterior pituitary |
In early studies, endocrine analysis relied on collecting blood samples to determine circulating hormone concentrations so that marine mammals held in captivity were sampled more frequently than those in the wild (Yoshioka et al. 1986; Cornell et al. 1987; Duffield et al. 1995). The first ground-breaking hormonal study on marine mammals investigated serum testosterone in two captive male bottlenose dolphins (Harrison and Ridgway 1971). Broad-scale blood sampling for endocrine analysis in field studies has been possible in few circumstances such as during haul-out of the semi-aquatic pinnipeds (e.g., Atkinson and Gilmartin 1992; Gardiner et al. 1996; Harcourt et al. 2010), or when sampling relatively small numbers of fully aquatic mammals during health assessments (e.g., Tripp et al. 2008) or even during lethal harvests (e.g., Suzuki et al. 2001; Kjeld et al. 2004, 2006). The need for physical restraint out-of-water to ensure collection of blood samples uncontaminated by seawater has hampered endocrine evaluations in most species, particularly of large or rare cetaceans. More recently however, sampling of media other than blood for hormone profiles has been increasingly recognized as a useful approach for free-ranging aquatic mammals (reviewed by Amaral 2010; Hunt et al. 2013).
Hormones are removed from circulation through metabolic processes and eventually excreted from the body through various pathways (depending on the species), providing alternative opportunities for measuring hormones and their byproducts. Hormone concentrations have been measured in a variety of body tissues and excreta from marine mammals, including saliva (Pietraszek and Atkinson 1994; Atkinson et al. 1999; Hogg et al. 2005; Amaral et al. 2009), milk (West et al. 2000), ocular secretion (Amaral et al. 2009), respiratory exudate (Hogg et al. 2005, 2009), adipose tissue (Mansour et al. 2002; Kellar et al. 2006, 2009; Pérez et al. 2011), muscle (Yoshioka et al. 1994), urine (Walker et al. 1988; Robeck et al. 1993; Wakai et al. 2002; Muraco et al. 2010) and feces (Larkin et al. 2005; Rolland et al. 2005; Biancani et al. 2009; Burgess et al. 2012a, b). These alternative endocrine approaches have already been widely applied to monitoring reproductive processes in captive individuals (see Sect. 2.1) and show promise for live, free-ranging populations. In fact, measuring levels of excreted hormones may offer advantages over blood levels because these represent averaged values pooled over time (i.e., integrated over the gut passage time for fecal samples), rather than a single point-in-time measure. However, it is important that for each new species under study, careful biochemical and biological validation of hormones in each sampled medium is conducted (Lasley and Kirkpatrick 1991). Furthermore, researchers must carefully consider the biology, ecology, and habits of the species, as well as the project’s scale, when deciding on which media are most appropriate (see Hunt et al. 2013).
To date, a few field studies have successfully sampled feces (Lanyon et al. 2005; Larkin et al. 2005; Rolland et al. 2005; Burgess et al. 2012a, b) or blubber (Kellar et al. 2006, 2009; Pérez et al. 2011) to assess reproductive hormone profiles of both sexes of live cetaceans and sirenians. Obviously, logistical difficulties arise with collection of such samples from elusive and large marine mammals (reviewed by Hunt et al. 2013). In some cases, floating feces voided by large whales have been sampled by dip-net during focal follows of, for example, right whales (Rolland et al. 2005; Hunt et al. 2006), sperm whales (Smith and Whitehead 2006; Marcoux et al. 2007), gray whales (Newell and Cowles 2006), blue and humpback whales (Lefebvre et al. 2002) either in feeding grounds or along migration routes. Sinking feces have also been collected from smaller cetaceans by snorkelers towed by boats traveling in close proximity to dolphin groups, although a large proportion of fecal material disperses too rapidly for collection (Parsons et al. 1999, 2003). One innovation to facilitate collection has been the use of ‘sniffer’ dogs trained to detect floating whale feces (Rolland et al. 2006). Another approach has been to collect feces of uncertain origin, either floating or as stools washed ashore, and then conduct molecular analysis for taxon and/or sex identity (Rolland et al. 2005). In live-capture studies of smaller marine mammals, fecal samples have been collected either opportunistically as they are voided during restraint (Lanyon et al. 2005, 2010b; Larkin et al. 2005) or directly by inserting a soft latex tube into the distal rectum (Biancani et al. 2009; Burgess et al. 2012a, b).
For blubber hormone analysis, remote biopsy darting has been used to obtain small cores of fatty hypodermis from free-swimming cetaceans (Kellar et al. 2006, 2009) and this also has potential application in pinnipeds (e.g., Hoberecht et al. 2006). One unique study on Weddell seals (Leptonychotes weddellii) analyzed hormones in urine samples that had become preserved in ice (Constable et al. 2006). However, the routine collection of fluid samples is logistically difficult when collecting in an aquatic environment, unless the mammal is first removed from the water.
Perhaps the most innovative approach for measuring hormone levels in marine mammals has been the use of respiratory exudate or ‘blow’, which is currently in the early stages of development (see Hunt et al. 2013). Cetaceans ventilate a larger percentage of the respiratory system with greater force than other mammals (Ridgway et al. 1969) and their lungs are heavily vascularized (Pabst et al. 1999), causing expulsion of a substantial volume of lung exudate droplets (along with respiratory gases) with each exhalation. Preliminary research on captive bottlenose dolphins and large free-ranging baleen whales (humpback whales Megaptera novaeangliae and North Atlantic right whales Eubalaena glacialis) has demonstrated that at least some exhaled blow samples (collected into a sampling device at the end of a cantilevered pole) contain detectable steroid hormones (Hogg et al. 2005, 2009). However, current analytical techniques only detect presence/absence and not quantitative concentrations of steroid hormones (see Trout 2008), suggesting that important methodological issues need to be addressed before hormone analysis of exhaled blow can be applied more widely to assess physiological state of free-ranging marine mammals (Hunt et al. 2013). The relative ease with which blow samples can be collected and the promise that these may be chemically analyzed to answer a number of biological questions (Acevedo-Whitehouse et al. 2010; Frère et al. 2010) have already led several researchers to begin collecting these samples (e.g., Gero and Whitehead 2007). Ultimately, less-invasive hormone monitoring (i.e., using non-blood samples) provides marine mammal scientists with the capability to conduct both basic and applied physiological-endocrine research that can be integrated with other disciplines including genetics, behavior, nutrition, animal health, ecology and evolution.
The discipline of conservation endocrinology is still evolving for free-ranging marine mammals, and will undoubtedly continue to provide new and valuable information to ensure the survival of viable marine mammal populations into the future. Exciting developments in wildlife endocrine studies are enabling us to move beyond assessing an individual’s reproductive status to being able to understand an individual’s reproductive potential (i.e., fertility status). Managers of marine mammal populations need to know not only how many individuals are present and/or how many are of breeding age (i.e., effective population size), but also understand an individual’s contribution to population growth. Most recently, sophisticated biomarkers (including anti-Müllerian hormone, AMH, inhibin A, inhibin B) have been developed to provide direct measures of gonadal function as well as fertility potential (ovarian reserve) in both female and male mammals (Table 11.2) (Lee and Donahoe 1993; Tremellen et al. 2006; Kumar et al. 2010). The first reports of detection of AMH and inhibin A and B in marine mammals, including Florida manatees (Wilson et al. 2011), bottlenose dolphins (Schwierzke-Wade et al. unpubl. data), beluga whales and dugongs (Wetzel et al. unpubl. data) suggest that these biomarkers offer promise as a means of evaluating the ‘reproductive quality’ of individuals comprising a population. With careful development and validation, the use of such biomarkers may provide insight into the effects of stressors on critical biological functions such as reproduction, which can then be used to inform effective and focused conservation efforts.
2.5 Diagnostic Imaging
Diagnostic imaging (ultrasonography and endoscopy) has been used to examine the urogenital tracts and implement advanced reproductive technologies (including artificial insemination) of terrestrial wildlife (Hildebrandt et al. 2003). These techniques have been applied to fully marine mammals, but in relatively limited ways due to the need for restraint and immobility during the procedures. Ultrasonography is a useful technique for examination and differentiation of internal soft tissues and compared to other reproductive imaging techniques is arguably the simplest, safest, less invasive and most cost effective method. It has been useful for obtaining vital information regarding reproductive activity and fetal development in mostly captive cetaceans (Brook 1997, 1999; Brook et al. 2001, 2004). Recent advances in ultrasound technologies have enabled researchers to examine gonadal activity through development of testes and ovarian follicles, monitor ovulatory cycles, implement artificial insemination, and estimate gestational age of fetuses and therefore parturition date for small captive marine mammals (Robeck et al. 1998, 2001, 2005b; Brook 1997; Brook et al. 2001, 2004; Lacave et al. 2004). Some captive cetaceans have been trained to station for ultrasound examination (Brook et al. 2001), whilst in a field situation, ultrasound may be applied to individuals removed from the water (Wells et al. 2004; Goldstein et al. 2006) or even restrained alongside a vessel if a portable device with waterproof transducer is deployed (Nichols 2005). For larger, but still tractable marine mammals, e.g., big males or individuals with thick blubber layers, ultrasonography may be relatively challenging and the outcome of examinations doubtful, whilst it is not practical for the largest free-ranging cetaceans.
Perhaps the greatest value of diagnostic sonography with respect to reproduction has been as an adjunct tool to physical examination and endocrinology. The use of ultrasonography for validation of reproductive state has been crucial in developing hormone-based diagnostic tests for pregnancy (e.g., in wild sirenians: Burgess et al. 2012a, Fig. 11.1) and for confirming pregnancy in captive cetaceans. Pseudo-pregnancy is possible in captive marine mammals (i.e., elevated hormone levels in animals that are not carrying a fetus) (Atkinson 1997; West et al. 2000) and it is only through the use of ultrasound examination, that true pregnancy can be discriminated from this condition (Robeck et al. 2001). Furthermore, ultrasound combined with endocrine monitoring offers insight into how hormonal changes directly relate to ovulation (Robeck et al. 2001). Interestingly, the first evidence of facultative-induced ovulation in a cetacean, the beluga, was determined using serial urinary hormone monitoring combined with ovarian ultrasound (Steinman et al. 2012). Reproductive cycles of both males and females of some cetacean taxa have been determined through routine repeat ultrasounds of the gonads (e.g., inshore bottlenose dolphins: Brook 1997; Indo-Pacific dolphins: Brook et al. 2004). As ultrasound devices become smaller, more robust and affordable, and their value apparent, their use in marine field situations is only likely to increase.
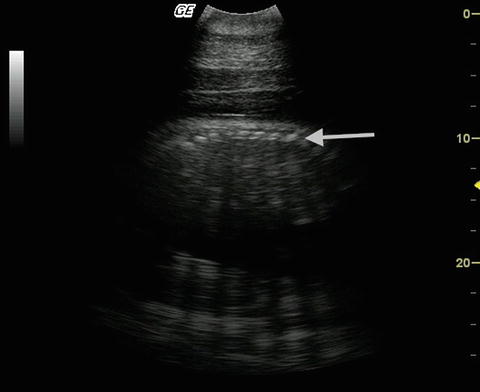
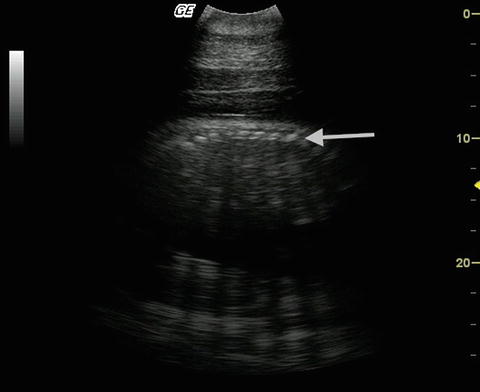
Fig. 11.1
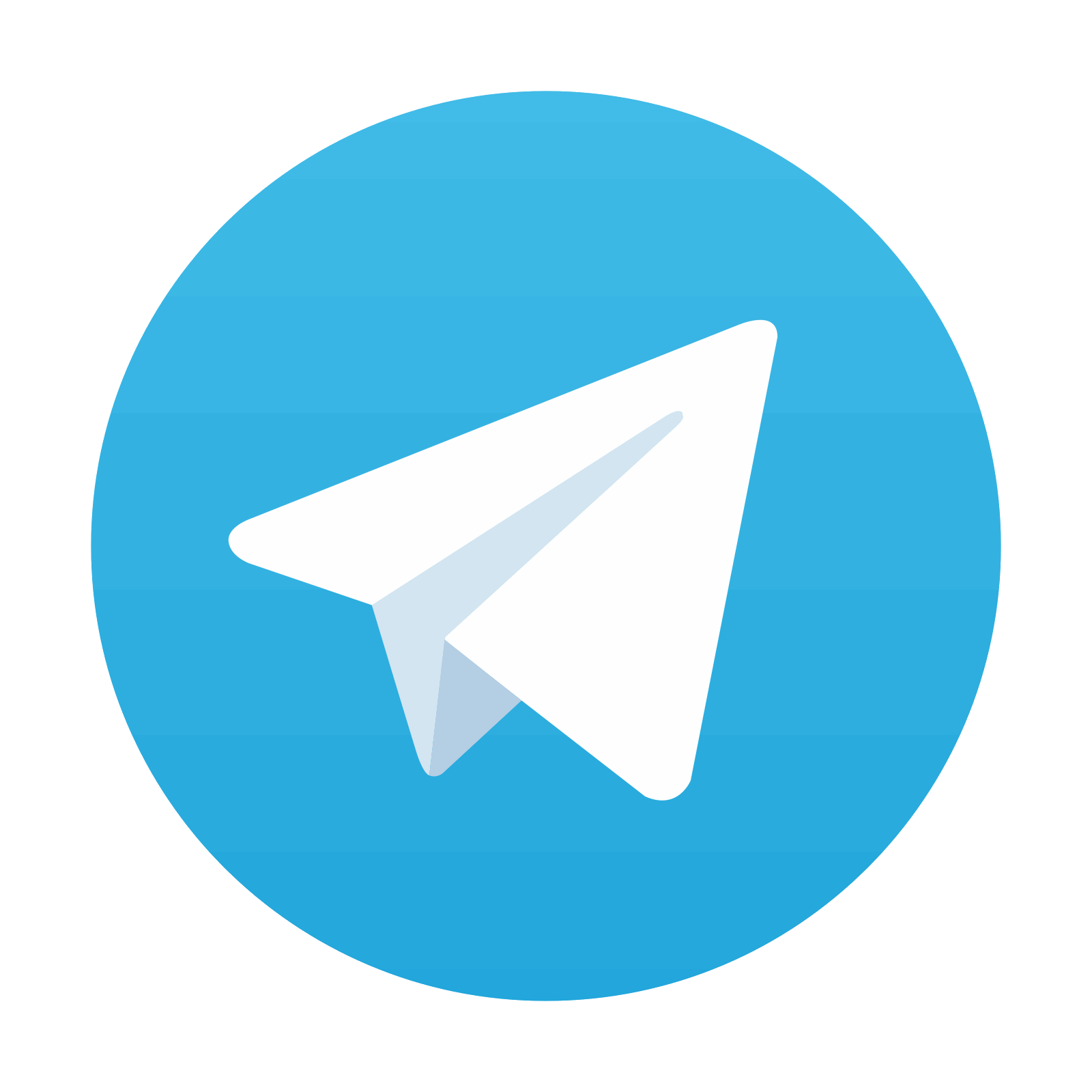
Ultrasound image showing a sagittal cross-section of a fetus of a free-ranging dugong. An arrow marks the bony axial components of the fetus (Burgess et al. 2012a)
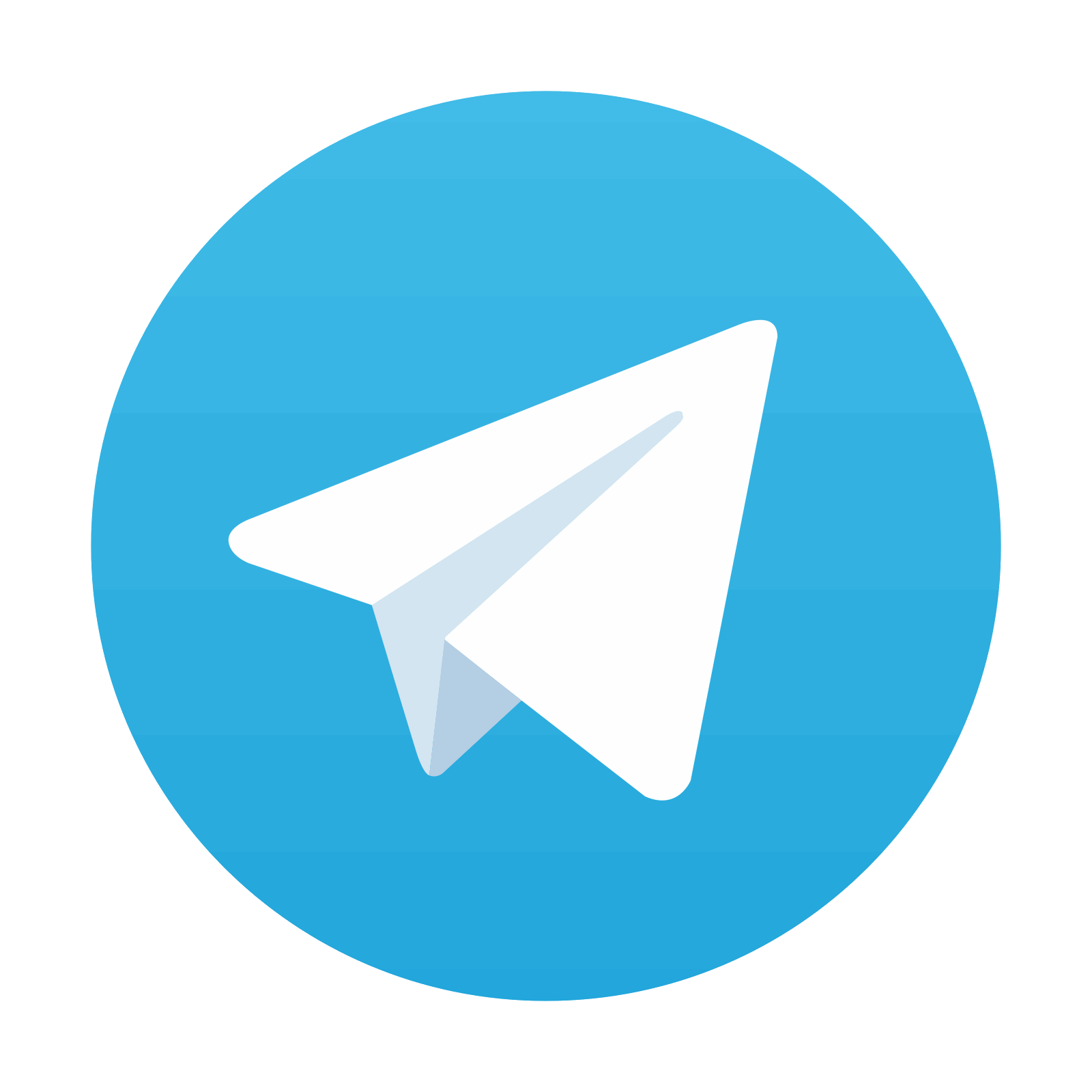
Stay updated, free articles. Join our Telegram channel

Full access? Get Clinical Tree
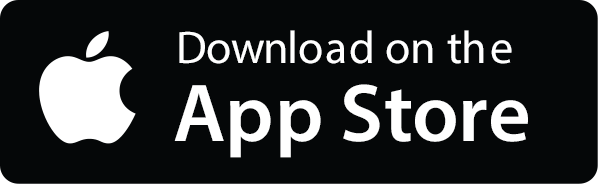
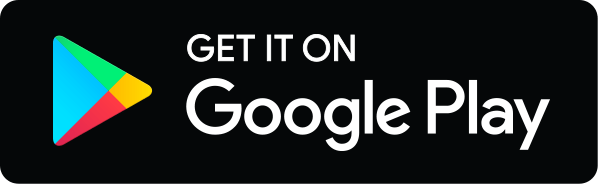
