Derivative reagent
Detection
Sensitivity
Reproducibility
Stable derivative
Removal of redundant reagent by drying
Removal of redundant reagent by solvent extraction
Interfering side-products
Secondary amino acids detected
OPA
Fluor
fmol
Good
No
No
No
No
No
PITC
UV
pmol
Good
Yes
Yes
No
No
Yes
FMOC-Cl
Fluor
fmol
Good
Yes
No
Yes
Yes
Yes
Dansyl-CL
UV/Fluor
pmol
Good
Yes
No
No
Yes
Yes
FDNB
UV
pmol
Good
Yes
Yes
No
Yes
Yes
FDNPAA
UV
pmol
Good
Yes
Yes
No
Yes
Yes
FDNDEA
UV
pmol
Good
Yes
Yes
No
Yes
Yes
DABS-Cl
UV/Fluor
pmol
Good
Yes
Yes
AQC
UV/Fluor
fmol
Good
Yes
No
Yes
No
Yes
PITC
UV
pmol
Good
Yes
Yes
No
No
Yes
15.3.3 Capillary Electrophoresis
Capillary electrophoresis (CE) has been developed in the 1980s as a high-performance liquid technology. It is based on the use of an electric field to separate charged compounds such as amino acids. It has proven to be a powerful technique for the rapid and highly efficient separation of minute amounts of protein and amino acid sample (Issaq 2001). The analysis of AA by CE has been mainly performed with precolumn, postcolumn, and on-column derivatization techniques with UV chromophore or fluorophore reagents to provide better resolution and sensitivity. Komarova et al. (2004) used pre-capillary derivatization of amino acids with PITC and separation of PTC-derivatives by capillary zone electrophoresis (CZE) and detection at 254 nm. This method allows to widen a list of detectable components up to 19 (without tryptophan) and significantly improve detection limits. Thorough discussion of this method for AAA has been published (Poinsot et al. 2012). Derivatization of amino acids prior to chromatography with chiral reagents has gained in popularity, because the diastereomers formed can be resolved on conventional RP-HPL. Compared with conventional electrophoresis and HPLC, this method has the advantages of high sensitivity and high degree of automation. CE does not require derivatization, but sensitivity of CE-UV analysis can be increased by introduction of a UV-active label. Most of derivatization reagents used for HPLC method can be used for CE method.
The literature of amino acid analysis using CE has been extensively reviewed (Smith 1997, 1999; Poinsot et al. 2006). The new developments of CE, especially those concerning the novelties in detection methods and chirality and the different biological applications have been recently described (Poinsot et al. 2012).
15.3.4 Mass Spectrometry
Ion-exchange chromatography is limited by long run time and by the fact that co-eluting compounds may prevent accurate quantification in some cases. Some amino acids commonly have overlapping retention times by ion-exchange chromatography with postcolumn ninhydrin detection. For example, this is the case for methionine, phenylalanine, and histidine. Mass spectrometry methods, for instance GC-MS (Revelsky et al. 2003; Fiamegos and Stalikas 2006), LC-MS (Molnár-Perl 2003), and GE-MS have the potential to avoid these limitations.
GC-MS
Derivatization of amino acids needs to be performed before analysis to produce volatile compounds which are analyzed by gas chromatographic method. The main derivatization methods include silylation, alkylation (Marquez et al. 1994), and acylation. The silylation is the main derivatization method. Silylation is carried out using BSTFA and MTBSTFA under anhydrous conditions and heating or esterification/acylation in two successive steps (Fiamegos and Stalikas 2006). GC is easily combined with mass spectrometry and a vast number of methods for the preparation of volatile derivatives suitable for GC and GE-MS analysis of amino acids have been reported. Kataoka et al have summarized these approaches (Kataoka et al. 2000). GC/MS methods are known to have good precision and accuracy. Duncan et al have employed isotope dilution gas chromatography/electron capture negative ionization/mass spectrometry (GC/ECNI/MS) to provide accurate and reliable data on <100 fmol of material (Duncan and Poljak 1998; Fan et al. 2006).
LC-MS/MS
LC-MS/MS is comparable to traditional LC-ninhydrin detection method. Mass spectral detection shortens analysis times and reduces interference in analysis involved in the detection of inborn metabolic errors (Dietzen et al. 2008). Both GC-MS and iTRAQ-LC-MS/MS are suited for high-throughput amino acid analysis, with the former offering at present higher reproducibility in a completely automated sample pretreatment method, while the latter covers more amino acids and related amines (Kaspar et al. 2009a, b).
CE-MS
CE-MS is based on CE as a front-end fractionation coupled to a mass spectrometer. This method provides fast separation and high resolution and is compatible with most buffers and compounds to be analyzed. The method for the determination of underivatized amino acids based on capillary electrophoresis coupled to electrospray ionization mass spectrometry (CE-ESI-MS) has been described (Soga 2000; Soga et al. 2004). GE-MS is a valid alternative to GC-MS for targeted profiling of metabolites, such as amino acids, and possesses some significant advantages over GC-MS (Williams et al. 2007). A review related to the use of CE-MS in the field of metabolomics has been published (Ramautar et al. 2009).
NMR-Spectroscopy
Metabonomics provides a useful systems approach to understand global changes in metabolites in animals in response to alterations in genetics, nutrition, environments, and gut microbiota. He et al. have, for instance, compared the metabonome of serum between the genetically obese and lean pigs using a NMR-based metabolomic method (He et al. 2009, 2012) and compared serum and jejunum metabonome in the situation of intrauterine growth restriction of piglets (He et al. 2011a, b, c)
References
Battaqlia A, Bertoluzza A, Calbucci F, Eusebi V, Giorqianni P, Ricci R, Tosi R, Tuqnoli V (1999) High-performance liquid chromatographic analysis of physiological amino acids in human brain tumors by pre-column derivatization with phenylisothiocyanate. J Chromatogr B 730:81–93CrossRef
Bensen JR, Hare PE (1975) O-phthaladehyde: fluorogenic detection of primary amines in the picomole range. Comparison with fluorescamine and ninhydrin. Proc Natl Acad Sci USA 72:619–622CrossRef
Bosch L, Alegria A, Farre R (2006) Application of the 6-aminoquinolyl-N-hydroxysccinimidyl carbamate(AQC) reagent to the RP-HPLC determination fo amino acids in infant foods. J Chromatogr B 831:176–183CrossRef
Boutry C, Matsumoto H, Airinei G, Benamouzig R, Tome D, Blachier F, Bos C (2011) Monosodium glutamate raises antral distension and plasma amino acid after a standard meal in humans. Am J Physiol Gastrointest Liver Physiol 300:137–145CrossRef
Boutry C, Matsumoto H, Bos C, Moinard C, Cynober L, Yin YL, Tome D, Blachier F (2012) Decreased glutamate, glutamine and citrulline concentrations in plasma and muscle in endotoxemia cannot be reversed by glutamate or glutamine supplementation: a primary intestinal defect? Amino Acids 43:1485–1498PubMedCrossRef
Cynober L, Ziegler F, Coudray-lucas C, Chauffert M et al (1987) Comparison of methods of assay of amino acids by gas chromatography and ion exchange chromatography. Ann Biol Clin 45:537–540
Damm M, Michael H, Radspieler G, Marsche G, Kappe O (2011) Microwave-assisted high-throughput acid hydrolysis in silicon carbide microtiter platforms-A rapid and low volume sample preparation technique for total amino acid analysis in proteins and peptides. J Chromatogr A 1217:7826–7832
Darragh AJ, Garrick DJ, Moughan PJ et al (1996) Correction for amino acid loss during acid hydrolysis of a purified protein. Anal Biochem 236:199–207PubMedCrossRef
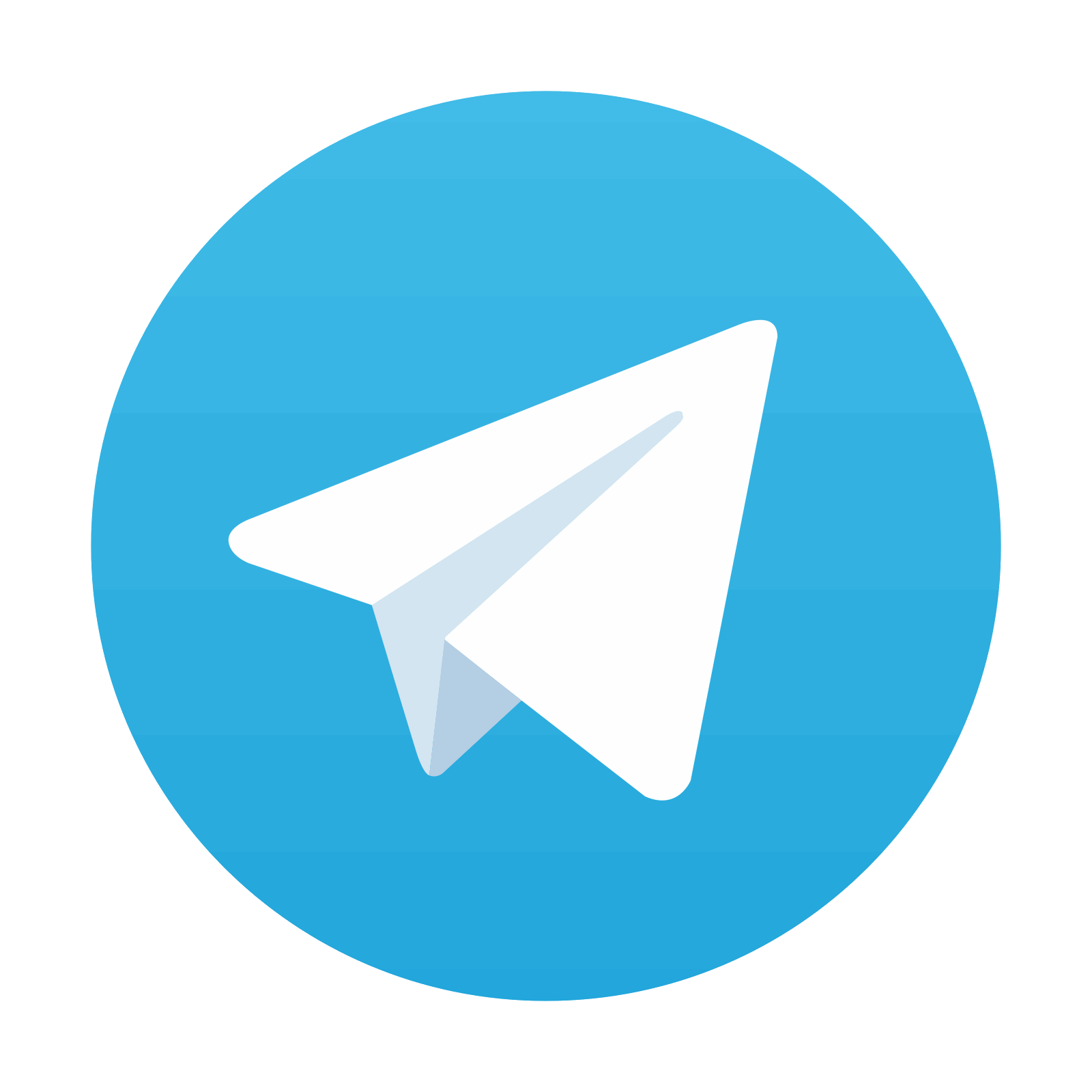
Stay updated, free articles. Join our Telegram channel

Full access? Get Clinical Tree
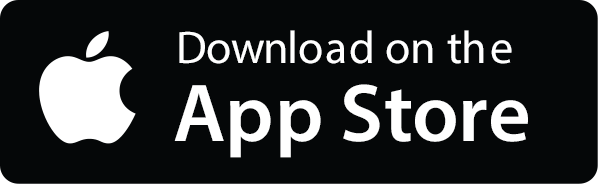
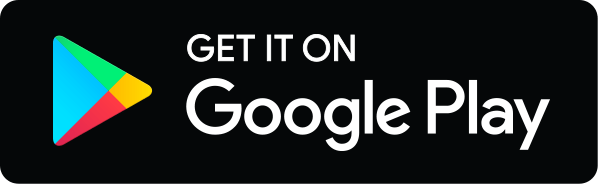