TESTIS
Stroma and Interstitium
Tunica Vaginalis
The tunica vaginalis consists of mesothelium and a connective-tissue layer that blends with underlying connective tissue of the scrotum (described in Chapter 16) and testis. When the testis is removed from the scrotum, the parietal layer of the tunica vaginalis remains attached to the inner surface of the scrotum, whereas the visceral layer, the peritoneal covering of the testis (and epididymis), remains intimately associated with the underlying capsule (tunica albuginea) of the testis. A space, the vaginal cavity, separates the parietal and visceral layers.
Tunica Albuginea
The tunica albuginea (Fig. 12-1) is a solid capsule of dense irregular connective tissue. It consists predominantly of collagen fibers, a few elastic fibers, and myofibroblasts. Intratunical interstitial endocrine cells occur in the cat. Meandering branches of the testicular artery and a network of anastomosing veins constitute the vascular layer (tunica vasculosa) of the tunica albuginea.
Septula Testis and Mediastinum Testis
The tunica albuginea is continuous with connective-tissue trabeculae, the so-called septula testis, which converge toward the mediastinum testis. These trabeculae are rather complete septa in dogs and boars, whereas in the other domestic animals, they are inconspicuous connective-tissue strands surrounding the large intratesticular vessels. The septula testis divide the testicular parenchyma into a varying number of testicular lobules, each containing one to four convoluted seminiferous tubules (Fig. 12-2). The septula testis are continuous with the mediastinum testis (Fig. 12-3), a connective-tissue area containing the channels of the rete testis and large blood and lymph vessels. The mediastinum testis of stallions and many rodents is relatively small and located in a marginal position, whereas in ruminants, pigs, cats, and dogs, it occupies a central position along the longitudinal axis of the gonad.
Interstitial Endocrine Cells
The intertubular spaces of the testis contain loose connective tissue, blood and lymph vessels, fibrocytes, free mononuclear cells, and interstitial endocrine (Leydig) cells (Fig. 12-4). Interstitial endocrine cells constitute approximately 1% of the entire testicular volume in adult rams, approximately 5% in bulls, and 20 to 30% in boars. In seasonally breeding males (e.g., camel), interstitial endocrine cell volume and number may change during the year. The interstitial endocrine cell is a large polymorphous cell with a spherical nucleus. The cell occurs in cords or clusters, and not every interstitial endocrine cell is in close contact with a capillary. Smooth endoplasmic reticulum (sER) (in bulls, a granular endoplasmic reticulum) is the dominating organelle in interstitial endocrine cells. sER membranes incorporate most of the enzymes necessary for steroid biosynthesis. Mitochondria of the interstitial endocrine cell have tubular cristae and are involved in the first step of steroid hormone production (e.g., transformation of cholesterol to pregnenolone). The relatively small Golgi complex does not participate in secretion of androgen. Release of androgen from interstitial endocrine cells is morphologically inconspicuous. Lipid inclusions are found in all species, but are particularly abundant in cats (Fig. 12-4). Between adjacent cells are intercellular canaliculi and gap junctions.
FIGURE 12-1 Schematic drawing of a bovine testis, epididymis, ductus deferens, and spermatic cord. The testicular lobules contain seminiferous tubules. Branches of the arteries and veins in the vascular layer supply and drain the testicular parenchyma and stroma.

FIGURE 12-2 Seminiferous tubules (S) and intertubular tissues (IT) of the canine testis. Hematoxylin and eosin (×140).

FIGURE 12-3 The mediastinum testis (A) of the cat is a connective-tissue area in the central longitudinal axis of the gonad and contains the anastomosing channels of the rete testis (B). Convoluted seminiferous tubules (C) empty into straight testicular tubules (D), which connect with the rete. The septula testis (E) are located between testicular lobules (F). Masson-Goldner (×35).

Two generations of interstitial endocrine cells, fetal and pubertal, develop from mesenchymelike precursors. In some species (e.g., bovine and porcine), a third generation of interstitial endocrine cells (early postnatal) is encountered.
Interstitial endocrine cells produce testicular androgens (testosterone) and, in boars, large amounts of estrogen as well. More than 90% of all androgens in the organism are produced by the testis. Among the main functions of testosterone (in some tissues, to be effective, testosterone must be converted by the enzyme 5-α reductase to dihydrotestosterone) are (a) promotion of normal sexual behavior (libido); (b) triggering of the growth and maintenance of the function of the penis, male accessory glands, and secondary sex characteristics; (c) control of spermatogenesis (together with follicle-stimulating hormone [FSH]); (d) negative feedback action on the hypophysis and hypothalamus; (e) general anabolic effects; and (f) the prenatal maintenance of the mesonephric (wolffian) duct and its differentiation into the epididymis and ductus deferens.
FIGURE 12-4 Intertubular region of the cat testis. The interstitial endocrine cells (IC) have round nuclei and many lipid inclusions. The small artery (A) with loosely arranged muscle cells represents the type with dual innervation by noradrenergic and cholinergic fibers. Semithin section (×450).

Convoluted Seminiferous Tubules
The convoluted seminiferous tubules (tubuli seminiferi convoluti) in most mammals are tortuous two-ended loops with a diameter between 150 and 300 µm (Fig. 12-3). They are lined by the stratified spermatogenic epithelium (germinal epithelium), surrounded by a lamina propria, and connected at both ends to straight testicular tubules by a specialized terminal segment (see Fig. 12-12). The length of all seminiferous tubules in the testis of the adult bovine amounts to approximately 5000 m. Histologically, the seminiferous tubules have three components: lamina propria, sustentacular cells (somatic, supporting, or Sertoli cells), and spermatogenic cells.
Lamina Propria
The lamina propria surrounds the seminiferous tubule. Its innermost layer is a basement membrane, often with club-shaped projections that extend into basal infoldings of sustentacular cells and spermatogonia. Collagen and elastic fibers connect the basement membrane to the flat peritubular cells, which form a stratum of one to five layers, depending on the species. At birth, these peritubular cells resemble mesenchymal cells, which gradually differentiate into contractile cells postnatally. In some species (e.g., boars), the cells acquire all features of typical smooth muscle cells; in other species (e.g., bulls), they represent myofibroblasts.
The peritubular cells contain actin filament bundles that are arranged in both circular and longitudinal directions and are responsible for tubular contractions. Thus, the peritubular cells participate in transport of tubular content and in spermiation, that is, the release of spermatozoa into the tubular lumen. The outermost layer of the tubular lamina propria consists of fibrocytes and collagen fibrils. Lymphocytes and monocytes invade the lamina propria but never the intact tubular epithelium.
Sustentacular Cells
Sustentacular (Sertoli) cells are derived from undifferentiated supporting cells of the prepubertal gonad. The undifferentiated cells are mitotically active, contain large amounts of rough endoplasmic reticulum (rER), and produce antiparamesonephric hormone, a glycoprotein that suppresses development of uterine tubes, uterus, and vagina in the male. During puberty, sustentacular cell differentiation is accompanied by a morphologic transformation and loss of mitotic capability.
The adult sustentacular cells are irregularly outlined, elongated cells (Fig. 12-5). Their broad base rests on the basement membrane, and the remaining cytoplasm extends upward to the tubular lumen. They are rather evenly spaced; approximately 20 sustentacular cells are seen in a cross section of an adult seminiferous tubule. Lateral and apical cytoplasmic processes of the sustentacular cells fill all the spaces between adjacent spermatogenic cells. The oval or pear-shaped nucleus is generally located in the broad basal portion of the cell, is often deeply infolded, and contains a large nucleolus. The basal portion and the central trunk region of the sustentacular cell contain mitochondria, an inconspicuous Golgi complex, abundant sER, little rER, free ribosomes, microtubules, actin and vimentin filaments, lysosomes, and lipid inclusions. Only a few organelles are found in the lateral and apical sustentacular cell processes.
The shape of the cell, the surface area, volume percentages of the nucleus and organelles (sER, lysosomes, lipid inclusions), and the amount, and organization of the cytoskeleton change in accordance with spermatogenic events (sustentacular cell cycle) (Fig. 12-6). Sustentacular cells form hemidesmosomes with the basement membrane. Temporary junctions with adjacent germ cells play a part in vertical displacement and release (spermiation) of the germ cells into the lumen of the tubule. Adjacent sustentacular cells are joined by tight junctions associated with actin filaments and subsurface cisternae of the ER. These junctions separate a basal compartment from an adluminal (apical) compartment, and constitute a diffusion barrier, also referred to as the blood-testis barrier. Renewal of spermatogenic stem cells and multiplication of spermatogonia take place in the basal compartment, to which intertubular tissue fluid has relatively free access. The blood-testis barrier selectively prevents many substances from entering the adluminal compartment, where the vital processes of meiosis and spermiogenesis take place in a controlled microenvironment. Another function of the blood-testis barrier is to prevent autoimmune reactions against postspermatogonial germ cells. Early spermatocytes must pass through these specialized intercellular junctions joining sustentacular cells without interrupting the physiologic blood-testis barrier. Such passage is probably accomplished by a zipperlike opening of these junctions, which closes again below the spermatocytes before they reach the adluminal compartment.
FIGURE 12-5 Diagrammatic representation of sustentacular cell–germ cell interrelationships in the bull. 1. Sustentacular cell nucleus. 2. Smooth endoplasmic reticulum. 3. Tight junctions between adjacent sustentacular cells constituting the morphologic equivalent of the blood-testis barrier. 4. Phagolysosomes. 5. Golgi complex. 6. Space occupied by a spermatogonium. 7. Space occupied by a primary spermatocyte. 8. Space occupied by a spherical spermatid. 9. Elongated spermatid within apical recess of sustentacular cell. 10. Basement membrane. 11. Peritubular cell (myofibroblast). (With permission from Mosimann M, Kohler T. Zytologie, Histologie und mikroskopische Anatomie der Haussäugetiere. Hamburg: Paul Parey, 1990.)

Sustentacular cells have nutritive, protective, and supportive functions for the spermatogenic cells. In addition, they phagocytize degenerating spermatogenic cells and detached residual bodies of spermatids. Sustentacular cells release the spermatozoa into the lumen of the seminiferous tubules (spermiation). They mediate the action of FSH and testosterone on the germ cells, participate in the synchronization of spermatogenic events, and secrete constituents of the intratubular fluid such as transferrin, androgen-binding protein, and inhibin. Inhibin is reabsorbed from the lumen of the efferent ductules and the initial segment of ductus epididymidis; it then reaches the bloodstream and exerts negative feedback on hypophyseal FSH secretion. Paracrine signals from sustentacular cells modulate the activity of nearby interstitial endocrine cells. Although normal sustentacular cells have minimally proven steroidogenic function, sustentacular cell tumors may produce large amounts of estrogen, leading to feminization of the animal.
FIGURE 12-6 Shape and size of the sustentacular cells (ovine; dark cells) vary characteristically in accordance with the stages of the seminiferous epithelial cycle. 1. Stage 2. 2. Stage 4. 3. Stage 8. Alpha-tubulin reaction (×560).

Spermatogenic Cells
Various spermatogenic cells, representing different phases in the development and differentiation of the spermatozoon, are located between the sustentacular cells. The sequence of events in the development of spermatozoa from spermatogonia is referred to as spermatogenesis and is subdivided into three phases: (a) spermatocytogenesis, the process during which spermatogonia divide mitotically several times and finally develop into primary spermatocytes; (b) meiosis, the maturation division of spermatocytes that results in spermatids with a reduced (haploid) number of chromosomes; and (c) spermiogenesis, the process of transformation of spermatids into spermatozoa. The duration of spermatogenesis is approximately 39 days in boars; 50 days in bulls, rams, and stallions; and 55 days in dogs.
Germ cell loss by apoptosis or degeneration is low during spermatocytogenesis, but affects between 6% and 18% of the cells during the two meiotic divisions in the ruminant spermatogenic epithelium. The main reason for this numerical decrease is an escape of cells into the tubular lumen during the second meiotic division. As a consequence, exfoliated germ cells are regularly observed within the lumen of the testicular excurrent duct system (see Fig. 12-15).
In addition to the cell population involved in spermatogenesis (cycling population), the spermatogenic epithelium contains a separate spermatogonia stem and precursor cell line, which guarantees uninterrupted spermatogenesis and can adapt to changing demands. Spermatogonia stem and precursor cells are morphologically similar to cycling spermatogonia and are also located in the basal tubular compartment.
Spermatocytogenesis
During spermatocytogenesis, spermatogonia multiply mitotically, resulting in A-, I- (intermediate), and B-spermatogonia and finally in preleptotene primary spermatocytes (Figs. 12-7 and 12-8). Primary spermatocytes no longer divide mitotically, but instead undergo two meiotic divisions, which result in a fourfold increase in the number of germ cells. Therefore, the number of spermatozoa that originate from one A-spermatogonium is decisively influenced by spermatogonial proliferation during spermatocytogenesis. In most mammals, a variable number of generations of A-spermatogonia are followed by one generation each of I- (intermediate) and B-spermatogonia, respectively. In a given tubular segment, the few A-spermatogonia are irregularly distributed. Daughter cells of A- and I-mitoses drift apart to achieve an even distribution. A-spermatogonia are the largest spermatogonia and share an extensive contact area with the tubular basement membrane. They possess prominent nucleoli and nuclei with a pale or cloudy appearance. The small B-spermatogonia have spherical nuclei containing numerous chromatin particles and less prominent nucleoli. Most spermatogonia of a given tubular segment are interconnected by cytoplasmic processes and form a network composed of individual cells (Fig. 12-7). The mitotic division of B-spermatogonia results in the formation of preleptotene primary spermatocytes. These cells and their descendents are interconnected by true cytoplasmic bridges and form a syncytium until shortly before spermiation. Preleptotene primary spermatocytes gradually lose contact with the basement membrane and are passively translocated into the adluminal tubular compartment across the intercellular junctions between the sustentacular cells. In preleptotene primary spermatocytes, the nuclear deoxyribonucleic acid (DNA) is replicated and all chromosomes consist of two sister chromatids.
Meiosis
During meiosis, two successive nuclear divisions occur, resulting in the formation of four haploid spermatids from one primary spermatocyte. Primary spermatocytes are the largest spermatogenic cells in the tubular epithelium and are located in the intermediate position between spermatogonia and spermatids. Because the prophase of the first maturation division is extremely prolonged, two generations of primary spermatocytes are present in many tubular sections (Fig. 12-8; see also Fig. 12-11). The prophase of the first maturation division is subdivided into the leptotene, zygotene, pachytene, diplotene, and diakinesis stages according to characteristic changes in nuclear chromatin. During the leptotene stage, the chromosomes become arranged in thin threadlike strands. In the zygotene stage, homologous chromosomes begin to pair and tetrads of four chromatids emerge. Visible evidence of pairing is the synaptic complexes seen under the electron microscope. Completion of pairing initiates the pachytene phase, during which crossing over occurs between the nonsister chromatids of the paired chromosomes. In microscopic preparations, primary spermatocytes are best identified when they are in this phase of meiosis (Fig. 12-8; see also Fig. 12-11). During the diplotene phase, the paired chromosomes pull away from each other, but sister chromatids remain attached through chiasmata (i.e., sites where crossing over has occurred). During the prophase of the first meiotic division, the cells grow consider ably. For instance, between preleptotene and diplotene, ovine primary spermatocytes increase in volume 4.8 times and their nuclei increase in volume 3.3 times. During diakinesis, the chromosomes shorten and broaden and the four separate chromatids are clearly evident. At the end of prophase, the nuclear membrane disappears.
FIGURE 12-7 1. Surface of a tubular whole mount (bovine) showing evenly distributed small B-spermatogonia and irregularly spaced larger A-spermatogonia. Protein gene product 9.5 reaction (×225). 2. Surface of tubular whole mount at higher magnification (×560). The small B-spermatogonia and the large future A-spermatogonia for the next round of spermatocytogenesis are both members of a common cellular network and are interconnected by narrow mutual processes (arrows) but remain individual cells. On the contrary, postspermatogonial germ cells undergo incomplete cell divisions and form true syncytia.

The metaphase, anaphase, and telophase of the first maturation division occur rapidly. During these phases, the paired chromosomes are first arranged at the equatorial plate. Subsequently, homologous chromosomes move to opposite poles of the cell to be distributed to the secondary spermatocytes, which have only half the number of chromosomes, but two chromatids each (dyads). Secondary spermatocytes are short-lived (a few hours), intermediate in size between diplotene primary spermatocytes and spherical spermatids, and occur exclusively in phase 4 of the seminiferous epithelial cycle (Fig. 12-8).
After a short period of interphase, during which no duplication of genetic material occurs, the secondary spermatocytes undergo the second maturation division, with a short prophase followed by metaphase, anaphase, and telophase, which are essentially similar to those of mitotic divisions. During this division, the centromeres divide and the sister chromatids of the secondary spermatocytes separate and are distributed to each of the spermatids resulting from that division. Thus, these cells possess a haploid set of chromosomes with 1N DNA each.
Spermiogenesis
The process by which interconnected clones of newly formed spermatids differentiate into individual testicular spermatozoa is referred to as spermiogenesis. The most important morphologic changes during spermiogenesis are formation of the acrosome, condensation of the nuclear chromatin, outgrowth of a motile tail, and loss of excess spermatid material (cytoplasm, water, organelles) not necessary for the later spermatozoon.
Spermiogenesis is divided into the Golgi phase, cap phase, acrosomal phase, and maturation phase (Fig. 12-9). In histologic sections, the Golgi and cap phases are characterized by spherical nuclei, whereas the acrosomal and maturation phases have elongated nuclei (Fig. 12-8; see also Fig. 12-11).
During the Golgi phase, proacrosomal granules appear in Golgi vesicles and eventually fuse to form a single acrosomal granule within a single acrosomal vesicle. Both structures make contact with an indentation of the nucleus, thus marking the anterior pole of the future sperm head.
During the cap phase, the acrosomal vesicle grows and forms the head cap that covers the anterior two thirds of the nucleus. In the late cap phase, the still spherical spermatid becomes polarized, and the nucleus and head cap are shifted to an eccentric position. The two centrioles assemble at the posterior pole of the nucleus, and the distal centriole gives rise to the outgrowing flagellum.
In the early acrosomal phase, the nucleus and cell body start to elongate in a craniocaudal direction. At the same time, the spermatids rotate so that the nucleus is directed toward the periphery of the tubule and the developing tail is directed toward the lumen. The spermatids, formerly separated by lateral susten tacular cell processes, are now embedded in apical recesses of these cells. As the nucleus begins to elongate, nuclear histones, which are responsible for the normal spatial nucleosomal arrangement of nuclear DNA, are gradually replaced by basic proteins (protamines), which allow dense packing of the chromatin. After this condensation, the DNA is transcriptionally inactive and resistant to noxious influences. During fertilization, protamines must be replaced by histones before decondensation of the chromatin. These histones are provided by the oocyte.
FIGURE 12-8 Typical morphology of the various cells in the spermatogenic epithelium (water buffalo). The numbers on the images correspond to the stages of the epithelial cycle (compare to Fig. 12-11). Spermatogonia (S); preleptotene primary spermatocytes (PL); leptotene primary spermatocytes (L); zygotene primary spermatocytes (Z); zygotenes entering pachytene (Z/P); pachytene primary spermatocytes (P); diplotene primary spermatocytes (D); secondary spermatocytes (II); Golgi-phase spermatids (G); cap-phase spermatids (C); early acrosome–phase spermatids (EA); acrosome-phase spermatids (A); maturation-phase spermatids (M). Semithin sections (×1560).

During the maturation phase, nuclear condensation is completed. In the region of the future middle piece of the spermatozoon, most of the mitochondria gather around the axoneme in a helicoidal manner. The system of the outer fibers and the fibrous sheath of the future principal piece develop. The volume of a spermatid in the late maturation phase amounts to only 20 to 30% of that of a cap-phase spermatid. Autolytic processes are generally responsible for this reduction. In the bovine, sustentacular cell processes contacting the spermatids participate in absorption of spermatid material. Before spermiation, the cytoplasmic bridges between maturation-phase spermatids are disconnected and excess cytoplasm is detached as residual body. Residual bodies have different fates; they may be phagocytosed by sustentacular cells, lost into the tubular lumen, or subjected to rapid autolysis when attached to the apical border of the tubular epithelium. A small remaining portion of spermatid cytoplasm, the cytoplasmic droplet of young spermatozoa, is lost during epididymal passage.
Spermatozoon
Spermatozoa vary in length from approximately 60 µm (boars, stallions) to 75 µm (ruminants). Under the light microscope, the spermatozoon consists of two portions: the head and the tail. With the electron microscope, the tail is subdivided further into the neck, middle piece, principal piece, and end piece (Fig. 12-10).
Head
The shape of the nucleus and acrosome determines the shape of the head of the spermatozoon, which is species-dependent and subject to great variations. The anterior pole of the nucleus is covered by the acrosomal cap, with an outer and an inner acrosomal membrane that fuse at the caudal end. The acrosomal cap contains several hydrolytic and proteolytic enzymes (e.g., acrosin), which are set free during the acrosome reaction of capacitated spermatozoa in the uterine tube. Acrosomal enzymes are needed for the penetration of the zona pellucida during fertilization. The posterior region of the acrosome is characterized by a narrowing of the cap and condensation of its contents. This area is the equatorial segment of the acrosome. The base of the nucleus is surrounded by the postacrosomal sheath, which consists of fibrous proteins rich in sulfur. In dead spermatozoa, the sheath stains intensely with certain dyes, such as eosin or bromophenol blue. This reaction is used to evaluate the quality of an ejaculate. The plasma membrane of the postacrosomal head region contains receptor molecules necessary for the recognition of a homologous oocyte. At the posterior surface of the sperm head, the nuclear envelope lines an implantation groove where the tail is inserted in a jointlike manner.
FIGURE 12-9 Spermiogenesis (bull). A. Spherical spermatid, Golgi phase. 1. Golgi complex; 2. Golgi vesicle; 3. acrosomal vesicle containing acrosomal granule; 4. nucleus; 5. developing tail. B. Spherical spermatid, cap phase. 1. Golgi complex; 2. head cap containing acrosome; 3. developing tail. C. Elongated spermatid, acrosomal phase. 1. acrosomal cap containing acrosome; 2. nucleus; 3. manchette; 4. Golgi complex. D. Elongated spermatid, maturation phase. 1. nucleus (condensation completed); 2. middle piece with mitochondrial sheath; 3. annulus; 4. principal piece; 5. Golgi complex. (With permission from Mosimann W, Kohler T. Zytologie, Histologie und mikroskopische Anatomie der Haussäugetiere. Hamburg: Paul Parey, 1990.)

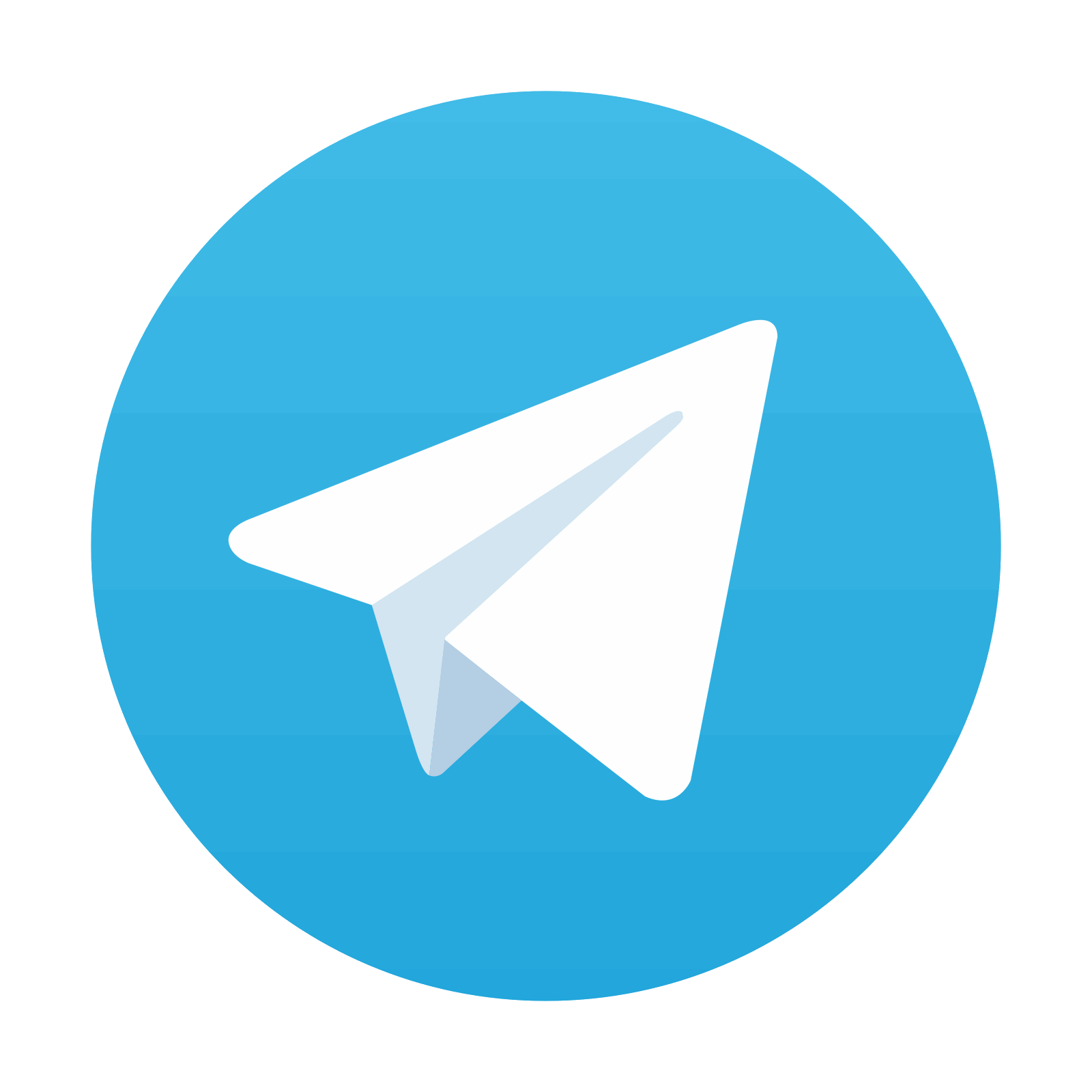
Stay updated, free articles. Join our Telegram channel

Full access? Get Clinical Tree
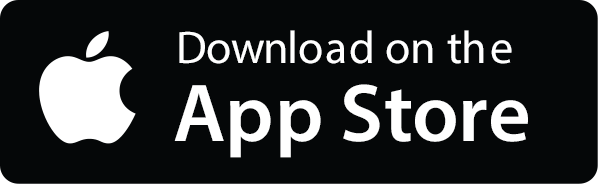
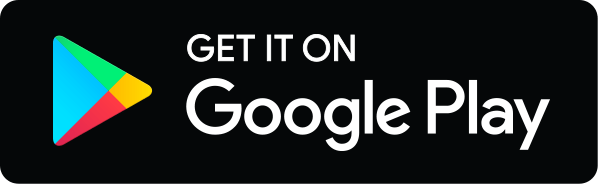