EMBRYOLOGY
The fusion of a female and male gamete results in a zygote. After repeated cleavage during the transport through the uterine tube to the uterine cavity in higher mammals (Eutheria), the zygote develops into a fluid-filled vesicle, the blastocyst, with a wall of simple epithelium, the trophoblast. An eccentrically located inner cell mass extends from the wall into the blastocyst cavity. The free-floating blastocyst is nourished by secretions from the endometrium. Because the increasing demand from the growing embryo necessitates a more efficient nutritive arrangement (i.e., a vascular transport system), the embryo produces membranes that, in a process called implantation, gradually attach to the endometrium. A close relationship is established between fetal and maternal circulatory systems for physiologic exchange. As a result, a combined organ, the placenta, is formed. A fetal part (pars fetalis) and a maternal part (pars uterina) are recognized. The fetus and fetal membranes, including the fetal part of the placenta, are known as the conceptus. The manner of attachment and subsequent formation of the placenta is termed placentation.
The first events in placentation occur in the blastocyst stage. The trophoblast is essential for the transfer of nourishment to the offspring during intrauterine life but has no function after birth of the young and is expelled with the afterbirth (secundinae). The inner cell mass differentiates into three germ layers in two stages, initially forming ectoderm and endoderm and then mesoderm between the two original layers. These three germ layers form the embryo, but they also participate in the formation of the fetal membranes. The ectoderm contributes to a vesicle enclosing the embryo, the amnion; it provides buoyancy and protected space for development for the embryo. The amnion contains antiangiogenic factors and is used for eye surgery to prevent unwanted vascular-ization. The endoderm contributes to the yolk sac, communicating with the midgut, and to the allantois, a diverticulum from the hindgut (Fig. 14-1).
The further development of the fetal membranes is directed by the mesoderm. Lateral mesoderm splits into a somatic and a splanchnic layer. The resulting intramesodermal cleft gives rise to the body cavity (intraembryonic coelom), which, at this stage, extends to form an extraembryonic cavity called the exocoelom. Somatic mesoderm combines with the trophoblast layer to form the chorion or with the ectoderm of the amnion. These membranes constitute the extraembryonic somatopleure. Splanchnic mesoderm fuses with the endoderm of the yolk sac and the allantois, and together these layers form the extraembryonic splanchnopleure.
FIGURE 14-1 Diagram of fetal membranes. The arrangement is subject to wide variation in different species. The amnion encloses the amniotic cavity and embryo. Ectoderm and somatic mesoderm (somatopleure) form the amnion and chorion, while the yolk sac and allantois consist of endoderm and splanchnic mesoderm (splanchnopleure). (From Dellmann H-D, Carithers JR. Cytology and Microscopic Anatomy. Philadelphia: Williams & Wilkins, 1996.)

Somatic mesoderm, and hence the chorion, is initially avascular. Blood islands and vessels first arise in the splanchnic mesoderm of the yolk sac and later in the splanchnic mesoderm of the allantois. Eventually, the avascular mesoderm of the chorion fuses with the mesoderm of the allantois, forming the allantochorion, which then becomes vascularized and filled with allantoic fluid. At birth, the allantoamnion, a fusion of the allantois and amnion, protrudes through the ruptured allantochorion and assists in dilation of the cervix at parturition. The placental vessels, together with the vitelline and allantoic ducts, are contained in the body stalk and later develop into the umbilical cord (see Fig. 14-10A).
Secretions from the fetal membranes play an important role in the maternal recognition of pregnancy and its continuation. The secretions result in an alteration of the endometrial signal to the ovary, leading to the preservation of the corpus luteum. In the pig, estrogen secreted by the blastocyst is antiluteolytic, while in ruminants, interferon τ (IFNτ) exerts a similar effect.
CLASSIFICATION
The placenta is subject to great variation in structure, which is species-dependent. The variation has given rise to many different systems of classification, based on five main criteria: (a) fetal extraembryonic membrane contributions, (b) macroscopic structure of the placenta, (c) three-dimensional structure of the maternal– fetal interface, (d) tissue layers of the fetal–maternal interhemal barrier, and (e) degree of fetal–maternal anchoring and fate of maternal tissues at birth.
Fetal Extraembryonic Membrane Contributions
Two types of placentae occur in domestic mammals: the choriovitelline placenta (omphaloid or yolk sac placenta) and the chorioallantoic placenta. The yolk sac, and hence the choriovitelline placenta, develops before the allantois and subsequent chorioallantoic placenta. Early during gestation, the choriovitelline and chorioallantoic placentae coexist temporarily, and then the choriovitelline placenta regresses.
Choriovitelline Placenta
The choriovitelline or yolk sac placenta is formed when the yolk sac wall (splanchnopleure) combines with the chorion and then contacts the endometrium (Figs. 14-1 and 14-16). It may be fully or partially vascularized by the vitelline plexus, which connects with the omphalomesenteric vein leading to the developing heart. Blood returns from the embryo to the vitelline circulation in the yolk sac via the omphalomesenteric artery, which branches from the dorsal aorta.
In sows and ruminants, the yolk sac wall is simply apposed to the uterine epithelium, and the yolk sac begins its involution 3 to 4 weeks after conception. However, in carnivores and mares, the yolk sac is well developed early in gestation and persists throughout gestation. In carnivores, a temporary lamellar choriovitelline placenta develops (see Fig. 14-16). In all domestic mammals, the choriovitelline placenta is of minor importance in physiologic fetal–maternal exchange.
In the inverted yolk sac placenta, the endoderm of the yolk sac is directly exposed to the uterine luminal content. It occurs in mice, rats, rabbits, and guinea pigs and participates in selective absorption and transmission of maternal immunoglobulins for fetal immunoprotection.
Chorioallantoic Placenta
When the allantois fuses with the chorion, an allantochorion is formed. The allantochorion contacts the endometrium, resulting in a chorioallantoic placenta (Fig. 14-1). This organ is the most efficient for mediating physiologic exchange between mother and offspring. It is very well vascularized by umbilical arteries and vein.
The principle of providing a vast area of fetal–maternal interchange determines the placental structure at the gross and microscopic anatomic levels. The structural variations of the placenta are numerous. Intermediate forms exist, and the placenta also changes its internal structure during the period of gestation.
Macroscopic Structure of the Placenta
The region of the chorion where folds, lamellae, or villi increase the surface area is called the frondose chorion (chorion frondosum); the region without projections is the smooth chorion (chorion laeve). Considering the frondose chorion region and its uterine counterpart, four types of placentae are recognized macroscopically. In domestic mammals, diffuse, cotyledonary, and zonary placentae are present (Fig. 14-2), whereas in humans and some other species, the discoid type develops.
In the diffuse placenta (placenta diffusa), most of the chorionic sac forms a frondose chorion attached to the endometrial epithelium (sows, mares, camels) (Figs. 14-2A and C; see also Figs. 14-5 and 14-11A).
FIGURE 14-2 Schematic drawings of the fetus in the fetal membranes to show differences in placental shape and internal structure among species. Horse with a diffuse villous (microcotyledonary) placenta (A); ruminants (cow), with a cotyledonary villous placenta (B); pig with a diffuse folded placenta provided with macroscopically visible areolae (C); carnivores (here, cat) with a zonary (girdle) lamellar or tightly folded placenta (D). Allantoic cavity (1); amnionic cavity (2); yolk sac (3); allantochorion (4). In the higher magnification views, the maternal side is dark, the fetal side is bright, and the areola–gland complex (5) in horse and pig and the endometrial glands (6) in bovine and cat are black.

In the cotyledonary placenta (placenta multiplex), tufts of chorionic protrusions (cotyledons) attach to preformed endometrial prominences (caruncles) (Fig. 14-2B). Cotyledons and caruncles combine to form placentomes. In the intercaruncular area, a smooth chorion is apposed to the endometrial epithelium (ruminants) (see Fig. 14-13).
In the zonary placenta (placenta zonaria), the frondose chorion forms a band or girdle around the equator of the chorionic sac (Fig. 14-2D; see also Fig. 14-17). Outside the girdle, the smooth chorion apposes to the endometrial epithelium (carnivores and minks) (see Fig. 14-17).
In the discoid placenta (placenta discoidalis), the frondose chorion forms a disc-shaped area of fusion with the endometrium (e.g., humans, apes, mice, rats, rabbits, guinea pigs).
Three-Dimensional Structure of the Interface Between Maternal and Fetal Tissues
The capacity for exchange between maternal and fetal tissues is enhanced by the increased surface area of a folded, villous, or lamellar interface (Fig. 14-2). This vast fetal–maternal contact area is further increased by an irregular cell surface or microvilli on both cells of the trophoblast and endometrial epithelial cells (see Figs. 14-8, 14-9, and 14-12B).
In the folded placenta, the fetal–maternal contact surface is increased by the presence of vascularized macroscopic folds (plicae) of varying magnitude and microscopic ridges (rugae) of varying height (sows) (see Figs. 14-5 through 14-7).
In the villous placenta, the allantochorion forms cotyledons (i.e., arborizing chorionic villi with vascular mesenchymal cores that fit into corresponding caruncular crypts). Together, the villi and crypts form macroscopic (ruminants) or microscopic placentomes (mares) (see Figs. 14-11 through 14-13), or independently, the villous tree extends into the maternal intervillous blood space (humans).
In lamellar placentation, the trophoblast of the allantochorion forms an intercommunicating network that envelopes the endothelium of maternal blood vessels (carnivores) (see Fig. 14-18), or it is in direct contact with maternal blood in trophoblast-lined vascular channels (rats, mice, rabbits).
Tissue Layers of the Fetal–Maternal Interhemal Barrier
The fetal and maternal placental circulations are separated by tissue layers that form the placental or fetal–maternal inter-hemal barrier. The barrier is a highly selective transport avenue in fetal–maternal exchange (Fig. 14-3). The fetal component of the barrier is chorionic tissue that is vascularized by allantoic vessels; it consists of three tissue layers: endothelium, mesenchyme, and trophoblast. The maternal counterpart consists basically of three corresponding layers in reverse order: endometrial surface epithelium, connective tissue, and endothelium.
Although the number of tissue layers of the fetal component is constant, the number of maternal layers varies with the species. Therefore, placentae are further classified on the basis of the number of uterine tissue layers.
In the epitheliochorial placenta (placenta epitheliochorialis), all three layers of the maternal component are present. This type of placenta occurs in sows, mares, and ruminants (see Figs. 14-8, 14-12A, and 14-15). In ruminants, however, binucleated trophoblast cells migrate and fuse with the endometrial surface epithelium, and the placenta is designated as synepitheliochorial.
In the endotheliochorial placenta (placenta endotheliochorialis), the endometrial surface epithelium and the underlying maternal connective tissue are absent, and endothelium alone separates the maternal blood from the trophoblast. This type of placenta is primarily present in carnivores and minks (see Fig. 14-18B).
In the hemochorial placenta (placenta hemochorialis), all three maternal layers are absent, rendering the trophoblast freely exposed to maternal blood. Up to three layers of trophoblast may be present (hemotrichorial), of which at least one is composed of syncytiotrophoblast (fusion of trophoblast cells into a sym-plasm). This type of placenta is present in humans, rats, mice, rabbits, and guinea pigs.
Fetal–Maternal Anchoring and Fate of Maternal Tissues at Birth
The degree to which the endometrium is changed and the fetal membranes are anchored into the maternal tissue determines the amount of uterine tissue lost at parturition. On this basis, two types of chorioallantoic placentation, nondeciduate and deciduate, are recognized (Fig. 14-3).
In the nondeciduate placenta, the fetal components interlock with relatively intact uterine tissue, from which they separate without much loss of endometrium. Epitheliochorial placentation (ungulates) is an example of nondeciduate placentation.
In deciduate placentae, the transformed part of the endometrial stroma, the decidua, is shed with the fetal membranes after parturition.
NOURISHMENT OF THE EMBRYO
The main physiologic principle of the chorioallantoic placenta is the substantial exchange between maternal and fetal blood. The substance that nourishes the developing offspring is termed embryotroph. The portion of embryotroph contributed from maternal blood is hemotroph, while the uterine glandular secretions and cell fragments form histotroph. Nutrients from both the hemotroph and histotroph are taken up by the trophoblast.
Histotroph nourishes the offspring before implantation. During gestation, histotroph is present in areolae, which are indentations of the chorion opposite the openings of endometrial glands. The areolae are scattered in the diffuse placenta of pigs and mares, or they are located in the smooth chorion of the ruminant placentae. In the carnivore placenta, histotroph is found in the junctional zone; it is typically seen in the areola–gland complex in the diffuse placenta, as well as in relation to the smooth chorion in the cotyledonary placenta (Fig. 14-2).
FIGURE 14-3 Schematic drawings of the tissue layers of the fetal–maternal interhemal barrier of placenta, including fetal components of the allantochorion (F) and maternal components (M). Dependent on species a reduction of the maternal components takes place, illustrated here by steps (A–F) until the trophoblast of the chorion comes into direct contact with maternal blood. Species differences in the trophoblast are also shown. The different placental types and corresponding species are: A. Epitheliochorial—sow, mare. B. Synepitheliochorial—ruminants (a–e show migration of binucleate trophoblast cells [a, b], fusion of binucleate cell with uterine epithelial cell [c], maternal–fetal hybrid cell [d], which secretes granular content [arrows] into maternal stroma, and degenerating hybrid cell [e]). C. Endotheliochorial—carnivores (bitch, queen). D. Hemotrichorial—rat, mouse. E. Hemodichorial—rabbit. F. Hemomonochorial—human, guinea pig. (Modified from Leiser R, Kaufmann P. Placental structure: in a comparative aspect. Exp Clin Endocrinol 1994;102:122–134.)

After successful implantation, the embryo is nourished by hemotroph, metabolites that cross the placental barrier from maternal circulation.
PLACENTAL VASCULARITY AND CIRCULATION
The two blood circulatory systems of the epitheliochorial and endotheliochorial placentae always remain morphologically separated by a species-dependent number of tissue layers (see Tissue Layers of the Fetal–Maternal Interhemal Barrier above). In hemo-chorial placentae, the uterine vessels are absent from the placental barrier, and the blood flows through trophoblastic tubules or intervillous spaces (lacunae). Regardless of the type of chorio-allantoic placentation, trophoblast and fetal endothelium are always present in the placental interhemal barrier (Fig. 14-3).
In the maternal part of the placenta, circulating blood is either contained in vessels or bathes the trophoblast directly. Uterine vessels also form placental hematomas, due to local degeneration of endometrial tissue. The hematomas are deposits of stagnant maternal blood between endometrial epithelium and the trophoblast in carnivores and ruminants (see Fig. 14-17).
The fetal placental capillaries have smaller lumina than the maternal capillaries (see Fig. 14-8); both are partially fenestrated and surrounded by basal laminae (Fig. 14-3; see also Fig. 14-18B).
The supply of oxygenated blood to the maternal part of the placenta is derived from the uterine artery and anastomoses from the ovarian and vaginal arteries. The arteries are enlarged during pregnancy, and their placental capillaries develop into a species-specific architecture reflecting the internal three-dimensional structure of the placenta (Fig. 14-4; see also Fig. 14-11B). The maternal part of the placenta is drained by uterine veins.
FIGURE 14-4 Combined maternal and fetal vascular cast (A), corresponding schematic of blood vessels from the placenta of the pig at day 99 of pregnancy (B), and idealized patterns of placental blood flow (C). The maternal arterioles (1) run to the top of maternal ridges, where they branch into the maternal capillary networks (2), which are drained by venules (2a). The fetal arterioles (3) can be followed into the fetal capillary networks (4), which have outlets through the fetal venules (4a). The large arrows in B demonstrate a mixture of crosscurrent (black/vertical versus white/horizontal arrows) and countercurrent (black/vertical versus white/vertical arrows) maternal–fetal blood flow interrelationship in the pig. The schematic C demonstrates, from left to right, blood flow of four different placental exchange types linked to typical species, including concurrent (theoretical), multivillous (human), crosscurrent (queen), and countercurrent (guinea pig). The density of dots in the fetal venules (4a) illustrates the efficiency of the various exchangers in diffusional exchange (e.g., oxygen). A: ×260. (C: From Dantzer V, Leiser R, Kaufmann P, et al. Comparative morphological aspects of placental vascularization. Trophoblast Res 1988;3:235.)

Fetal placental vessels take up oxygen and nutrients from maternal circulation and deliver waste products. To facilitate exchange, the different placental circulatory systems have multivillous, crosscurrent, or countercurrent flows between allantoic and uterine vessels; although the least efficient, the concurrent exchange system is only theoretical (Fig. 14-4). It is also noteworthy that the fetal veins leave the placenta apposed to the origin of uterine arterioles, thus enhancing the capability of exchange. The capillaries underlying maternal epithelium and the trophoblast bend and dilate. The bending of the vessels provides a flow stress that enhances vascular growth through interaction with hormones and cytokines. Dilation of blood vessels slows blood flow, enhancing the possibility for the active exchange of nutrients.
The yolk sac placenta, when present, receives deoxygenated blood from the omphalomesenteric arteries, which arise from the abdominal aorta. Oxygenated blood is then returned to the heart by the omphalomesenteric veins.
The fetal part of the chorioallantoic placenta receives deoxygenated blood from the paired umbilical arteries, which originate from the caudal aorta, and returns oxygenated blood through the umbilical veins. The left umbilical vein carries blood to the heart via the liver and the caudal vena cava, whereas the right vein undergoes involution in the fetus.
SPECIALIZED CELLS OF THE PLACENTA
Decidual cells are specialized cells derived from fibroblasts in the endometrium. They may develop in the endotheliochorial placenta of carnivores (see Fig. 14-18A) and are always present in hemochorial placentae. Decidual cells are enlarged and rounded or polyhedral; they are subject to considerable species variations in size, contents (lipid, glycogen, hormones, and growth factors), and structure.
The trophoblast (simple epithelium that forms the wall of the blastocyst) performs many different functions including absorption, exchange of metabolites, and synthesis of hormones and other signal transmitter substances. This diversity is reflected in the complex structure of individual cells and the cell types developed by differentiation (see Sow Placenta below, as a species example). The discrete cell of the trophoblast is termed a cytotrophoblast (Fig. 14-3). Another form of differentiation is the binucleate trophoblast cell, or giant cell, found in ruminants and mares (see Figs. 14-14 and 14-15). If multiple trophoblast cells fuse, they form a syncytiotrophoblast (Fig. 14-3; see also Fig. 14-18). When both cytotrophoblasts and syncytiotrophoblasts are present (carnivores), the cellular form is primitive, whereas the syncytial form is more differentiated with respect to the development of organelles. The presence of binuclear or multinuclear trophoblast cells corresponds to increasing invasion of the endometrium. In ruminants and mares, a relatively low degree of invasion occurs in the presence of binucleate cells, compared to the extensive endometrial invasion of multinucleated syncytiotrophoblasts in carnivores, rodents, and primates.
CHANGES DURING PLACENTATION
The placenta continuously changes in size, shape, and internal structure throughout gestation. After implantation, it grows at a rapid, although gradually decreasing, rate and may be subject to minor involution before term. Rearrangements at the cellular level are reflected in apoptosis (regulated cell death) and mitotic activity. In addition, the physical barrier between the maternal and fetal circulatory systems progressively attenuates with time (for details see Function–Structure Relationships below).
FUNCTION–STRUCTURE RELATIONSHIPS
Structural differences in the various placental types do not necessarily indicate differences in function. The transfer mechanism of the respiratory gases (i.e., oxygen and carbon dioxide under gradient pressure) is essentially simple diffusion. Therefore, the diffusion distance across the interhemal barrier is of major importance. Capillaries from both fetal and uterine sides of the placenta approach and indent the respective epithelia during gestation (Fig. 14-3; see also Fig. 14-8). Consequently, despite a varying number of layers in the interhemal barrier, the diffusion barrier thins to 2 µm, which is similar for most species.
The plasma membrane, with its variety of receptors, is an important structure in cellular transport. The membrane regulates cellular uptake and transfer of nutrients as well as export of unusable metabolic components. As parts of the placental barrier, the plasma membranes are present only in epithelium and endothelium. To traverse a cell (or syncytium), a substance must pass both the apical and basal plasma membrane. Therefore, placental selective barrier and transport functions are critically dependent on the number and activity of plasma membranes to be traversed.
A directional preference from mother to fetus generally exists for important inorganic elements (e.g., calcium, phosphorus, iodine, and iron). This characteristic is especially true of iron, as no retrograde transfer of iron from fetus to mother occurs. In different species, however, the iron transfer takes place by different mechanisms. In carnivores, and to a lesser extent in ruminants, iron is absorbed from blood hemoglobin in maternal hemorrhages. In the sow and mare, and also to some extent in ruminants, the source of iron is a glycoprotein complex secreted by the endometrial glands. The trophoblast takes up iron from circulating maternal transferrin in hemochorial placentation. Calcium is transferred in different regions of the fetal membranes, depending on the species. In sows, the transfer occurs across the folded interhemal barrier of the interareolar region, whereas in cows, it occurs at the intercotyledonary region, and in the mare, across the areolar-gland complexes by a different mechanism than for iron.
The hemoglobin of the growing offspring contains different types of polypeptide chains during different phases of development. The earliest (nucleated) erythrocytes from the yolk sac mesoderm contain embryonic hemoglobin. Later in intrauterine life, hepatic and splenic erythrocytes carry fetal hemoglobin, and near the time of birth, a gradual change to bone marrow cells with adult hemoglobin takes place. Embryonic and fetal hemoglobins have a higher affinity for oxygen than adult hemoglobin, and thus, they are able to more efficiently extract oxygen, which diffuses across the placental barrier. This difference is an adaptation for intrauterine life with low oxygen pressure.
Maternal proteins of high molecular weight, such as immunoglobulin G, which protects newborns against infectious diseases, sufficiently bypass some placental barriers. Proteins cross over in endotheliochorial placentae with hematomas and hemo-chorial placentae, but they do not cross in epitheliochorial placentae, although some proteins may be transferred at hematomas in ruminants. Therefore, neonates of species with epitheliochorial placentae are totally dependent on the immunoglobulins in the colostrum ingested immediately after parturition when the epithelium in the intestinal tract is temporarily permeable for these large molecules.
Placental tissues show a very high degree of expression of various genes. The placental trophoblast secretes hormones, for example, chorionic gonadotropin (mares), placental lactogen (ruminants), estrogens, and progesterone. During pregnancy, the placenta also produces a wide spectrum of other factors for the regulation of metabolic activity, growth, and structural changes (paracrine factors). These factors are used or promoted differently among species to regulate gestation and complete successful placentation.
SPECIES DIFFERENCES
The beginning of placentation and length of gestation vary considerably in different species (Table 14-1).
Sow
In early stages, the yolk sac is unusually large and well vascularized; its maximal development occurs at approximately day 20. A choriovitelline placenta of insignificant extent is formed, but disappears as the yolk sac rapidly decreases in size.
The chorioallantoic placenta is diffuse, folded, epitheliochorial, and nondeciduate (Fig. 14-5). The fusiform chorionic sac adheres to the endometrium over its entire area, except at the avascular extremities and over the uterine gland openings, where areola–gland complexes are formed (Figs. 14-2, 14-6, and 14-7).
TABLE 14-1 Implantation and Gestation in Domestic Animals
Beginning of Implantation (day) | Gestation Time (days) | |
Bitch | 17–18 | 58–63 |
Queen | 12.5–14 | 63–65 |
Mare | 35–40 | 329–345 |
Sow | 13–14 | 112–115 |
Cow | 16–18 | 279–285 |
Ewe | 15–20 | 144–152 |
FIGURE 14-5 Longitudinal section of uterine horn with placenta (sow), late midpregnancy. Primary fold (plica) of endometrium (1); rugae (2); plica of allantochorion (3); endometrium with glands (4); myometrium (5); allantoic cavity (6) (×20). (Courtesy of A. Hansen.)

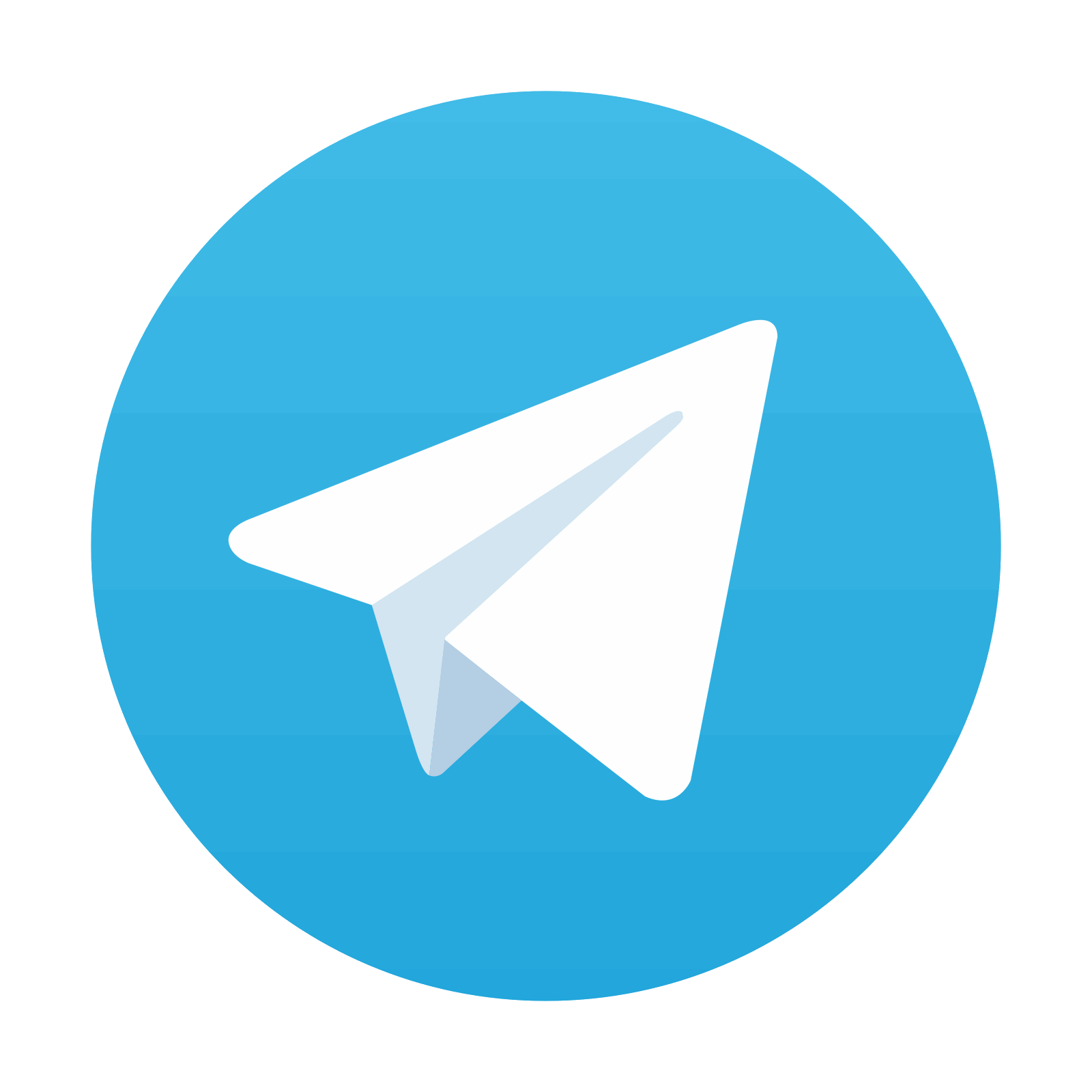
Stay updated, free articles. Join our Telegram channel

Full access? Get Clinical Tree
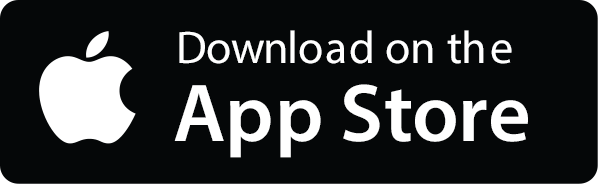
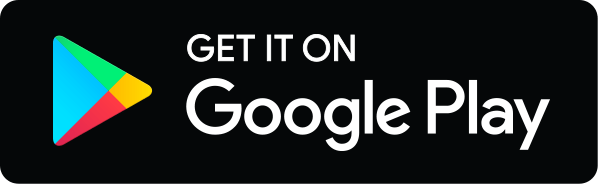