CHAPTER 57Maintenance of Pregnancy
In order to properly evaluate possible therapies to help mares maintain pregnancy, we first need to understand some of the reasons why mares suffer from either early embryonic death (EED) or abortion.
EARLY EMBRYONIC DEATH
Improvement in ultrasonographic equipment has permitted initial investigation of early embryonic losses between days 10 and 20 of gestation.1 This technique, combined with embryo recovery, permits investigation of embryo losses between day 6, which is the first time an embryo can be routinely recovered from the uterus, and day 11, which is the first time the vesicle can be consistently detected by ultrasonography. The real incidence of EED prior to day 6 is unknown. However, it was suggested that a major proportion of EED in infertile mares occurs in the oviduct.2 Workers in Wisconsin have suggested that in aged, subfertile mares, the primary problem may actually be the oocyte.3 In the future, much more clear delineation of the various incidences of problems from the different parts of the reproductive tract will be forthcoming. The incidence of EED has been reported to be between 5% and 30% of established pregnancies.4,5 In earlier studies utilizing ultrasonography, it appeared that EED occurred in mares much earlier than previously reported.1,6 Various causes and factors responsible for EED in mares, apart from presence of twins, have been suggested, including nutrition,7,8 plant estrogens and photo-period,9 endophyte infected tall fescue,10 seminal treatments,11 lactation stress, and foal-heat breeding,12 genital infections,4,5,12 chromosomal abnormalities, hormonal deficiencies,8 anabolic steroids,1 stress,8 failure of maternal recognition and deficiency of pregnant mare serum gonadotropin (PMSG or eCG) production,13 immunological factors,14,15 and even a higher incidence from some individual stallions.16 Migration of the conceptus was originally believed to be a contributing factor in EED; however, it is now known to be a normal characteristic of the horse conceptus.17 Lactating mares and mares bred during foal heat have been reported to have a higher incidence of EED than non-lactating mares.12,18,19 However, in a survey of 2,562 pregnancies in lactating and non-lactating mares, the incidence of EED was similar.20 Prior to the advent of ultrasonography, recognition and timing of EED was difficult. From data collected over 2 breeding seasons1 involving 356 mares diagnosed pregnant by ultrasonography, the overall incidence of EED through day 50 postovulation was 17.3%. The majority (77.1%) of EED occurred prior to day 35 postovulation. During the period 15 to 35 days postovulation, a greater (P < 0.05) incidence of EED occurred between days 15 and 20 (26.2%) and 30 and 35 (29.5%) postovulation compared to other time periods. Maternal recognition of pregnancy has been reported to occur between 14 and 16 days postovulation.21,22
Embryo development and morphology was useful to predict EED in an embryo transfer program.23
638 embryo transfers conducted over 3 yr were retrospectively examined to determine which factors (recipient, embryo and transfer) significantly influenced pregnancy and embryo loss rates and to determine how rates could be improved. On Day 7 or 8 after ovulation, embryos (fresh or cooled/transported) were transferred by surgical or nonsurgical techniques into recipients ovulating from 5 to 9 d before transfer. At 12 and 50 d of gestation (Day 0 = day of ovulation), pregnancy rates were 65.7% (419 of 638) and 55.5% (354 of 638). Pregnancy rates on Day 50 were significantly higher for recipients that had excellent to good uterine tone or were graded as “acceptable” during a pretransfer examination, usually performed 5 d after ovulation, versus recipients that had fair to poor uterine tone or were graded “marginally acceptable.” Embryonic factors that significantly affected pregnancy rates were morphology grade, diameter and stage of development. The incidence of early embryonic death was 15.5% (65 of 419) from Days 12 to 50. Embryo loss rates were significantly higher in recipients used 7 or 9 d vs 5 or 6 d after ovulation. Embryos with minor morphological changes (Grade 2) resulted in more ( P < 0.05) embryo death than embryos with no morphological abnormalities (Grade 1). Between Days 12 and 50, the highest incidence of embryo death occurred during the interval from Days 1 7 to 25 of gestation. Embryonic vesicles that were imaged with ultrasound during the first pregnancy exam (5 d after transfer) resulted in significantly fewer embryonic deaths than vesicles not imaged until subsequent exams. In the present study, embryo morphology was predictive of the potential for an embryo to result in a viable pregnancy. Delayed development of the embryo upon collection from the donor or delayed development of the embryonic vesicle within the recipient’s uterus was associated with a higher incidence of pregnancy failure. Recipient selection (age, day after ovulation, quality on Day 5) significantly affected pregnancy and embryo loss rates.23
In addition, a higher rate of EED has been reported with delayed insemination that is either greater than 12 hours24 or greater than 18 hours postovulation.25
It should be noted that formation of endometrial cups occurs on approximately day 35. EED is diagnosed when an embryonic vesicle seen previously is not observed on 2 consecutive ultrasonographic scans and/or when only remnants of a vesicle are observed. Ultrasonographic criteria for impending EED are an irregular and indented vesicle, fluid in the uterine lumen, and vesicular fluid that contains echogenic spots. EED is suspected, particularly after day 30, when no fetal heartbeat is observed, there is poor definition of fetal structure, fetal fluids are very echogenic, or the largest diameter of the fetal vesicle is 2 standard deviations smaller than the mean established for that specific day of age. Vesicles increasing in size more slowly than normal may also be characteristic of EED.6 Indications obtained by ultrasonographic scanning of impending loss at later stages include failure of fixation, an echogenic ring within the vesicle, a mass floating in a collection of fluid, and a gradual decrease in volume of placental fluid with disorganization of placental membranes (Figure 57-1). On occasion EED may be anticipated by identification of endometrial folds in an early pregnant uterus with or without visible fluid (Figure 57-2). Ultrasonographic scanning during early pregnancy is an extremely useful management tool for pregnancy detection and determination of EED. However, if pregnancy rates are not reported until day 50, the discrepancy between pregnancy and foaling rates decreases. In one study, the risk of pregnancy loss decreased as gestation increased.
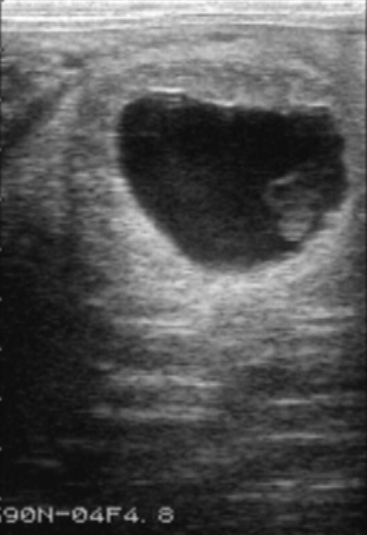
Figure 57-2 EED depicted by increased echogenicity of the fetal fluids and loss of fetal structure and clarity.
3740 mares were diagnosed pregnant by ultrasound around day 22 (± 5) (day 0 being the day of last service) and checked again around day 44 (± 12). Parturition or abortion was recorded for all mares which were still pregnant at the second test. Pregnancy loss between the two early pregnancy diagnoses was 8.9% (n = 3740). This rate was 5.5% (n = 2984) when one normal single pregnancy was diagnosed on the first test. It was increased when uterine endometrial cysts were present (24.4%; n = 86) or when the conceptus looked abnormal (34.8%; n = 279). Following 278 twin pregnancy tests, 9.7% of total resorptions of both twins and 61.5% of unilateral resorption of one of them were observed. Following 113 crushings of one twin conceptus, 20.3% of resorption of the remaining twin conceptus was noted. Abortion rate between day 44 and day 310 was 9.1% (n = 2988). Abortions were more frequent in the case of twin pregnancies (52.8%; n = 53). A higher abortion rate of the remaining twin conceptus was observed after crushing (23.8%; n = 80) or after natural resorption of one of the twins (13.5; n = 148). Early and late pregnancy loss increased with the age of the mare. Resorption rate, but not abortion rate, increased with parity. Breed had no effect on pregnancy loss. Reproductive status was not linked with embryonic death frequencies, but abortion rate increased if the mare became pregnant during foal heat. Similar resorption rates were observed in 261 different stallion “harems” (groups of mares served by the same stallion). Similarly, the rate of abortion was not linked to the stallion (192 groups of mares). The day by day risk of pregnancy loss decreased steadily as pregnancy progressed.26
In this study, the higher than expected pregnancy loss from mares with twin pregnancies that either were assisted in elimination of or spontaneously eliminated one pregnancy was surprising and does not parallel our experiences.
ABORTION
Abortion can be infectious or noninfectious, and the subject has been well reviewed.27 Many studies have been reported that implicate the relative importance of various causes. Unfortunately there are still many gaps in our knowledge, and the one concerning the common pathological diagnosis is “unable to determine the cause.” This may on occasion be our fault for not providing adequate or suitable material in a sterile or even timely manner to the laboratory.
A total of 309 miscarried equine fetuses and foals were submitted for necropsy at the Institut de pathologie du cheval between May 1st, 1986 and April 30th, 1990. An infectious origin could be established in 34.6% of the cases, of which 79.0% were of bacterial aetiology and 21.0% of viral aetiology. Of the bacteria, 26.1% of the isolates were streptococci and 19.3% were Escherichia coli. The only virus involved was the equine rhinopneumonitis virus.28
Pathology case records of 3,514 aborted fetuses, stillborn foals, or foals that died < 24 hours after birth and of 13 placentas from mares whose foals were weak or unthrifty at birth were reviewed to determine the cause of abortion, death, or illness. Fetoplacental infection caused by bacteria (n = 628), equine herpesvirus (143), fungi (61), or placentitis (351), in which an etiologic agent could not be defined, was the most common diagnosis. Complications of birth, including neonatal asphyxia, dystocia, or trauma, were the second most common cause of mortality and were diagnosed in 19% of the cases (679). Other common diagnoses were placental edema or premature separation of placenta (249), development of twins (221), contracted foal syndrome (188), other congenital anomalies (160), and umbilical cord abnormalities (121). Less common conditions were placental villous atrophy or body pregnancy (81), fetal diarrhea syndrome (34), and neoplasms or miscellaneous conditions (26). A diagnosis was not established in 16% of the cases seen (585). The study revealed that leptospirosis (78) was an important cause of bacterial abortion in mares, and that infection by a nocardioform actinomycete (45) was an important cause of chronic placentitis.29
Pathologic and microbiologic examinations were performed on 1,211 aborted equine fetuses, stillborn foals, and placentas from premature foals in central Kentucky during the 1988 and 1989 foaling seasons to determine the causes of reproductive loss in the mare. Placentitis (19.4%) and dystocia-perinatal asphyxia (19.5%) were the 2 most important causes of equine reproductive loss. The other causes (in decreasing order) were contracted foal syndrome and other congenital anomalies (8.5%), twinning (6.1%), improper separation of placenta (4.7%), torsion of umbilical cord (4.5%), placental edema (4.3%), equine herpesvirus abortion (3.3%), bacteremia (3.2%), fetal diarrhea (2.7%), other placental disorders (total of 6.0%), and miscellaneous causes (1.6%). A definitive diagnosis was not established in 16.9% of the cases submitted. Streptococcus zooepidemicus, Escherichia coli, Leptospira spp., and a nocardioform actinomycete were organisms most frequently associated with bacterial placentitis, and Aspergillus spp. was the fungus most often noted in mycotic placentitis. No viral placentitis was noticed in this series. Dystocia-perinatal asphyxia was mostly associated with large foals, maiden mares, unattended deliveries, and malpresentations. The results of this study indicate that in central Kentucky, the noninfectious causes of equine reproductive loss outnumber the infectious causes by an approximate ratio of 2:1, placental disorders are slightly more prevalent than non-placental disorders, Leptospira spp. and a nocardioform actinomycete are 2 new important abortifacient bacteria in the mare, the occurrence of contracted foal syndrome is unusually frequent, the incidence of twin abortion has sharply declined, and torsion of the umbilical cord is an important cause of abortion in the mare.30
Observations on 2,000 pregnancies revealed that 175 (8.7%) equine abortions occurred over a 4-year period. The peaks of abortion were at mid-gestation (20-32 weeks) and at late gestation (37–44 weeks). Abortion was higher in mares bred to donkey (10.4%) than in those bred to horse stallion (7.8%). Maximum abortions (80%) occurred in winter. Out of 175 equine abortions, 102 (58.3%) were due to infectious (bacterial, viral, fungal) causes, while 41.7% were due to noninfectious causes, viz. placentitis, malpresentation, twin pregnancy, foetal abnormality, toxaemia and trauma. Sixty-one abortions were grouped as due to unspecified noninfectious causes.31
Severe stress, such as colic, would appear to play a major part in late-term pregnancy loss.
The records of 105 pregnant mares and 105 non-pregnant horses with colic admitted to an equine hospital were reviewed. The 2 groups had similar types of colic and short-term survivability. Of the 105 pregnant mares, 31 were treated medically and 74 required surgical intervention. Thirty-three of the 105 mares died or were euthanatized. Thirteen (18%) of the 72 remaining mares aborted. Of 4 mares with severe medical cases, 2 died, 1 aborted, and 1 aborted and died. Of 27 horses with medical cases that required less intensive treatment, none died and 2 aborted. Of the 74 horses that required surgery, 45 survived to termination of pregnancy (foaling or abortion); 36 of these mares (80%) had a live foal. The type of surgical lesion had no effect on pregnancy outcome. Stage of gestation at initial examination, duration of anesthesia, or intraoperative hypoxia or hypotension had no effect on pregnancy outcome. However, when hypoxia occurred during colic surgery in the last 60 days of pregnancy, the mares either aborted or delivered severely compromised foals that did not survive.32
Stresses early in pregnancy associated with transport33 or rectal palpation for pregnancy diagnosis34 apparently have little or no effect on fetal loss.
Incidence of early embryonic death (EED) and associated changes in serum cortisol, progesterone and plasma ascorbic acid (AA) in transported mares were investigated. Mares were transported for 472 km (9 h) during either d 16 to 22 (T-3 wk, n = 15) or d 32 to 38 (T-5 wk, n = 15) of gestation. Blood samples were drawn from control, non-transported mares (NT-3 wk, NT-5wk, n = 24) and transported mares pre-trip, midtrip, and at 0, 12, 24, 48 and 72 h post-transport and daily for the next 2 wk. Incidence of EED between transported and non-transported mares was not different ( P >.05). Serum cortisol in all transported mares increased ( P <.05) relative to pre-trip values at midtrip and 0 h post-transport. Relative to NT mares, serum cortisol was higher ( P <.05) at midtrip in T-3 wk mares and 0 h post-transport in T-5 wk mares. Serum progesterone in all T mares increased ( P less than.05) at midtrip relative to pre-trip values and was higher ( P <.05) in T-3 wk mares than in NT-3 wk mares at midtrip and 0 h post-transport. Post-transport decreases ( P <.05) in concentrations of progesterone were observed in mares that aborted. Plasma AA in transported mares increased ( P <.05) at midtrip in T-5 wk mares and decreased (P <.05) relative to pre-trip values at 24 and 48 h post-transport (T-3 wk and T-5 wk mares, respectively.33
Placentitis
There is little doubt that placentitis is an emerging disease in intensively managed broodmare farms.35 It is possible that our sophisticated management procedures have led to more mares becoming pregnant, mares that without intense management may have struggled to become pregnant, thus creating a group of mares either susceptible to or more likely to be affected by placentitis.
Placentitis may be recognized by vulval discharge, premature lactation, or spontaneous abortion. Ultrasonography has been useful in examining the placenta at the region of the cervical star (Figure 57-3).
Feto-placental infection caused by microorganisms was the most common cause of death of fetuses, stillborn foals, and foals that died within 24 hrs after birth in central Kentucky. Pathologically, three types of placentitis were seen: ascending, diffuse, and focal mucoid. The pathogenesis in each form is believed to be different and each is associated with certain types of causative bacteria or fungi. Ascending placentitis was the most common type of placentitis prior to the 1998 foaling season in Kentucky. This form is thought to be the result of microorganisms gaining access to the cervical portion of the placenta during gestation by spread from the lower reproductive tract through the cervix. Streptococci and E. coli were the most commonly isolated bacteria. Diffuse or multifocal placentitis was less commonly diagnosed and is associated with hematogenous spread of microorganisms to the uterus of the mare with subsequent infection of the placenta. This form was associated with infection by bacteria in the genera Leptospira, Salmonella, Histoplasma, and Candida. Focal mucoid placentitis is also known as nocardioform placentitis. Nocardioform placentitis has emerged as the most commonly diagnosed type of placentitis over the last two foaling seasons and is characterized by unique pathology and distinct bacteria. At present the pathogenesis of this form is unknown. Diagnosis of placentitis during gestation is often difficult. Most mares show no outward signs of infection. Some mares will undergo premature mammary development with lactation and, occasionally, a vaginal discharge is present. Transrectal and transabdominal ultrasound examinations are useful in arriving at a diagnosis if placentitis is suspected. Various treatment modalities have been utilized in an attempt to maintain gestation for as long as possible to enhance foal viability. Placentitis results in several outcomes. In addition to abortions and stillbirths, the mare may produce small, weak foals or normal foals. The small, weak neonates represent a special management and medical challenge. These individuals have an increased risk of sepsis and orthopedic problems requiring extensive care.36
Placentitis is a common cause of equine abortions. In a majority of cases, the route of infection is believed to be ascending through the cervix, and an area of the chorion adjacent to the cervical star shows characteristic pathology in aborting mares. This area is depleted of chorionic villi, thickened, discolored, and covered by fibronecrotic exudate. Mares with placentitis due to a hematogenous infection show multifocal pathology of the chorionic surface of the placenta. Clinical signs include udder development, premature lactation, cervical softening, and vaginal discharge. Treatment is often unsuccessful once the mare has developed clinical signs. Ultrasonographic evaluation of the placenta in late gestational mares allows the clinician to detect preclinical signs of placentitis and premature separation, which then could be treated in its early stages. Transabdominal ultrasonography: Focal areas of utero-placental thickening and partial separation of the allantochorion from the endometrium in the uterine body and horns, can be observed by this approach. The combined thickness of the uterus and the placenta (CTUP) should not be more than 12 mm at any site. In addition to evaluating the placenta a biophysical profile of the fetus can be performed by a transabdominal approach. Transrectal ultrasonography: This approach provides an excellent image of the caudal portion of the allantochorion adjacent to the cervical star. Using transrectal ultrasonographic evaluation of the placenta, we have observed abnormal thickness and partial separation of the allantochorion from the endometrium in mares with clinical signs of placentitis. In advanced stages, the space between the uterus and the placenta is filled with hyperechoic fluid. Normal values of the CTUP in an area immediately cranially and ventrally of the cervix were determined to be: <8 mm between day 271 and 300; <10 mm between day 301 and 330; and <12 mm after day 330. Increased CTUP suggests placental failure and pending abortion. Treatment of placentitis should be aimed toward elimination of the infectious agents, reduction of the inflammatory response, and reduction of the increased myometrial contractility in response to the ongoing inflammation. Broad spectrum antibiotics, anti-inflammatories (flunixin meglumine, 1.1 mg/kg BID; phenylbutazone, 4 mg/kg BID) and tocolytics (Altrenogest; Clenbuterol), are recommended for treatment of placentitis. Pentoxyfylline (7.5 mg/kg p.o. BID) has been suggested to increase oxygenation of the placenta through an increased deformability of red blood cells.37
Mare Reproductive Loss Syndrome (MRLS)
Chapter 56 specifically discusses MRLS.
A brief description of the problem and the steps taken to elucidate the etiology are listed below.
During 2001, central Kentucky experienced acute transient epidemics of early and late fetal losses, pericarditis, and unilateral endophthalmitis, collectively referred to as mare reproductive loss syndrome (MRLS). A toxicokinetic/statistical analysis of experimental and field MRLS data was conducted using accelerated failure time (AFT) analysis of abortions following administration of Eastern tent caterpillars (ETCs; 100 or 50 g/day or 100 g of irradiated caterpillars/day) to late-term pregnant mares. In addition, 2001 late-term fetal loss field data were used in the analysis. Experimental data were fitted by AFT analysis at a high ( P <.0001) significance. Times to first abortion (“lag time”) and abortion rates were dose dependent. Lag times decreased and abortion rates increased exponentially with dose. Calculated dose X response data curves allow interpretation of abortion data in terms of “intubated ETC equivalents.” Analysis suggested that field exposure to ETCs in 2001 in central Kentucky commenced on approximately April 27, was initially equivalent to approximately 5 g of intubated ETCs/day, and increased to approximately 30 g/day at the outbreak peak. This analysis accounts for many aspects of the epidemiology, clinical presentations, and manifestations of MRLS. It allows quantitative interpretation of experimental and field MRLS data and has implications for the basic mechanisms underlying MRLS. The results support suggestions that MRLS is caused by exposure to or ingestion of ETCs. The results also show that high levels of ETC exposure produce intense, focused outbreaks of MRLS, closely linked in time and place to dispersing ETCs, as occurred in central Kentucky in 2001. With less intense exposure, lag time is longer and abortions tend to spread out over time and may occur out of phase with ETC exposure, obscuring both diagnosis of this syndrome and the role of the caterpillars.38
From the preceding abstract quotations it appears that infectious causes of abortion, twins, and stress constitute a major proportion of reported fetal demise late in pregnancy, and many poorly defined factors such as uterine environment and the hormonal milieu are involved earlier in pregnancy. A background on endocrinology of the mare during pregnancy may be useful in providing rational approaches to therapy.
ENDOCRINOLOGY OF PREGNANCY
In mares, maternal recognition of pregnancy associated with production of an immunosuppressive agent, a pregnancy specific protein called early pregnancy factor, may be as early as 48 hours after conception. Early pregnancy factor has been detected in mice, sheep, human beings, and mares, and may, in the future, aid in detection of pregnancy and EED. The second time of maternal recognition of pregnancy probably arises on or before day 5 or 6 after ovulation, as fertilized ova are transported from the oviduct through the uterotubule junction into the uterus by 5 or 6 days after ovulation but unfertilized oocytes generally are retained in the oviducts.39–42 The third recognizable time for maternal recognition (or reinforcement) of pregnancy occurs between 12 and 16 days. During this time, the conceptus is extremely mobile and probably secretes an antiluteolytic substance that prevents release of PGF2α, thus maintaining the corpus luteum and preventing the mare from returning to estrus.
Two experiments were performed to determine the critical time at which the equine blastocyst must be present within the uterus of the mare to prevent regression of the corpus luteum, and thus establish the critical time for the maternal recognition of pregnancy. A non-surgical blastocyst collection technique was developed to study this relationship between the blastocyst and the maternal ovary. Results from these experiments demonstrated that the cyclic life-span of the corpus luteum is not affected by the presence of the blastocyst within the mare’s uterus until after Day 14 after ovulation. Luteal function was prolonged when blastocysts were removed on Day 15 or later. The critical period for the maternal recognition of pregnancy in the Pony mare appears to be confined to the period between Days 14 and 16 after ovulation.43
Two experiments were conducted to assess the effect of exogenous hormone treatment on uterine luminal prostaglandin F (PGF). In the first experiment ovariectomized pony mares received either corn oil (21 days, n = 3), estradiol valerate (21 days, n = 3), progesterone (21 days, n = 3) or estradiol valerate (7 days) followed by progesterone (14 days, n = 4). Progesterone-treated mares had higher ( P <.01) uterine luminal PGF compared with all other groups, and no differences were detected between other treatment comparisons. In Experiment II, uterine fluid was collected from 4 ovariectomized horse mares before and after treatment with estradiol valerate (7 days) followed by progesterone (50 days). Pre-treatment uterine luminal PGF levels were lower ( P <.001) than post-treatment levels (.03 vs 76.80 ng/ml). In a third experiment PGF was measured in uterine fluid of pony mares on days 8, 12, 14, 16, 18 and 20 of the estrous cycle and pregnancy. In nonpregnant mares a day effect ( P less than.03) was observed in which uterine fluid PGF increased during the late luteal phase and declined thereafter. In contrast, no day effect was observed in pregnant animals and uterine luminal PGF was lower ( P <.001) than in cycling animals. These studies indicate that exogenous progesterone administration results in a large increase in uterine luminal PGF, whereas pregnancy results in suppression. Taken collectively with previous work from our laboratory, these results suggest that while the endometrium of pregnant mares is capable of producing large amounts of PGF, the presence of a conceptus impedes its synthesis and/or release which allows for luteal maintenance.44
Comparisons of estrone, 17 beta-estradiol, and plasma progestin concentrations were made in uterine fluid and peripheral blood of nonpregnant and pregnant pony mares. Concentrations of these steroids were also measured within yolk sac fluid from blastocysts on days 12, 14, 16, and 18 of pregnancy to obtain more complete analyses of the uterine environment (uterine fluid plus yolk sac fluid) of early pregnancy. Thirty mares were randomly assigned to six treatment groups (n = 5/group), and uterine fluid and peripheral blood samples were obtained on days 8, 12, 14, 16, 18, and 20 post-ovulation. After a recovery period of one estrous cycle, mares were bred at their next estrus. Animals were hysterectomized on the same treatment day to which they had previously been assigned in the nonpregnant phase of this study. Using this design, uterine fluid and peripheral blood samples were collected from each mare on equivalent days of the estrous cycle and pregnancy. Significant differences in day trends were found between nonpregnant and pregnant animals for estrogens and progestins in both uterine fluid and peripheral plasma. Furthermore, these data demonstrate that large increases in estrogens occur after day 12 of pregnancy in uterine and yolk sac fluids, with estrone becoming the predominant estrogen by days 18 and 20 in yolk sac and uterine fluids, respectively. These changes were not detected in peripheral plasma, which indicates that changes occurring within the uterine environment are not discernible in the systemic circulation during early pregnancy. These results indicate that the large amounts of estrogens appearing in uterine fluids during early pregnancy are of conceptus origin and may be an important factor in regulating the environment in which the conceptus develop.45
The next piece of the puzzle was also provided by researchers in Florida.
Cycling pony mares were bred and used to test the effect of restricted conceptus mobility on luteal maintenance (i.e., maternal recognition of pregnancy). In Experiment 1, uterine horns were ligated to restrict conceptus mobility to one uterine horn, Group 1; one horn plus the uterine body, Group 2; or one horn, the body and approximately 80% of the second horn, Group 3. Pregnancies were monitored with real-time ultrasonography. Four of five mares in Group 1 and two of four mares in Group 2 returned to estrus (Day 16.0 +− 1.9 and 14.5 +− 0.7, respectively) and subsequently lost the embryonic vesicles (Day 17.2 +− 1.2 and 15.7 +− 0.7, respectively). None of the four mares in Group 3 lost the vesicles. There was a significant effect of the interaction of treatment (amount of uterus available to the conceptus) and day on plasma progesterone (P) concentration ( p < 0.005). In Experiment 2, conceptus mobility was restricted to one uterine horn in two groups of mares, of which the second was treated with the synthetic progestin, Regumate (allyl trenbolone). In the first group, each of three mares lost the vesicle (Day 17.3 +− 4.3). In the second group, four of five mares maintained the pregnancies, indicating that pregnancy failure was due to the effects of declining P. These data indicate that restricted conceptus mobility results in luteolysis in the mare, and that the subsequent decline in P leads to embryonic death. This supports the notion that unrestricted mobility of the equine conceptus, allowing it to interact with most the uterine endometrium, is necessary for luteal maintenance and conceptus survival.21
Estrogens
A minor maternal (ovarian) production of estrogen occurs between days 35 and 60 but has little diagnostic significance. A major increase in plasma estrogen concentration occurs after day 60.46 This increase comes from the fetal placental unit and, thus, is not affected by ovariectomy.
Plasma total (conjugated + unconjugated) oestrogens were measured from Day 0 to 100 of pregnancy and compared with the levels found during the oestrous cycle. From Day 0 to 35 of gestation, the concentrations were similar to those during dioestrus. An increase in total oestrogens between Days 35 and 40 was followed by a plateau of 3 ng/ml between Days 40 and 60 which was slightly higher than preovulatory concentrations. This first increase in total oestrogen level was produced by the ovaries since values were suppressed after ovariectomy; stimulation may be due indirectly to PMSG causing follicular growth. After Day 60, a second increase was detected and considered to be of feto-placental origin as the levels at this time were not suppressed after ovariectomy. By Day 85, oestrogen concentrations exceeded those detected in non-pregnant mares so that a direct measurement of total oestrogens in plasma by a simplified radioimmunoassay after Day 85 of gestation offers a reliable method of pregnancy diagnosis.46
Concentrations of estrogen in urine and serum peak at about day 210 of gestation47 and are thought to come from fetal gonads, which are enlarged at this time. If fetal gonads are removed, plasma estrogen concentrations drop immediately to very low levels.48 In general, estrogen detection in serum or urine has only limited value for pregnancy diagnosis since they become reliable only relatively late in pregnancy. Earlier it was not recommended to test for estrogens before 150 days of pregnancy, but more recently, measurement of estrone sulfate (conjugated) for diagnosis of pregnancy and evidence of a viable conceptus has been advocated after day 60 of pregnancy.49 Fetal death results in an immediate decrease in estrone sulfate concentration.49 If a mare in the second or third trimester of pregnancy begins to lactate, measurement of estrone sulfate may provide an index of fetal viability. This may be particularly useful when other tests of fetal viability such as ultrasonography and/or electrocardiographic monitoring are not available.
Biological, chemical, and immunologic tests are available for estrogen determination. Biological tests, such as increased nipple length in guinea pigs or induction of vaginal cytological findings typical of estrus in immature or ovariectomized mice, are rarely used. They have been replaced by chemical tests largely because of cost and time. Chemical tests for urinary estrogen rely on color changes and fluorescence in the presence of warm, concentrated sulfuric acid. Radioimmunoassay can be used for determination of plasma total estrogen (conjugated plus unconjugated)46 concentration or urinary estrone conjugates. The method described for plasma analysis46 requires enzymatic hydrolysis and extraction and may not reveal all estrone conjugates because the concentration of estrone sulfate is 1,000 times higher in the urine. Direct conjugated estrogen measurement on serum or milk has been described,50 and differentiation between pregnancy and estrus was possible after approximately 50 days. In mares, an enzymatic determination of unconjugated estrogens in the faeces for pregnancy diagnosis has been described.51 It was possible to confirm pregnancy after 120 days of gestation, and because this technique may be totally noninvasive to the mare, some benefits to the equine reproductive industry may be realized.
Immunoreactive urinary oestrogen conjugates were assessed in daily urine samples (∼ 5 samples/week) collected from 8 Przewalski’s mares maintained under semi free-ranging pasture conditions. The relative percentage contributions of immunoreactive urinary oestrogens during different reproductive states (oestrus, luteal phase, early, mid- and late gestation) were determined using high-pressure liquid chromatography. In general, conjugated forms of oestrone (oestrone sulphate and oestrone glucuronide) were the major excreted immunoreactive oestrogens in non-pregnant and pregnant Przewalski’s mares. Variations in urinary oestrogen conjugates indicated that the onset of oestrous cyclicity coincided with increasing daylengths, and the non-conception oestrous cycle was 24.1 +− 0.7 days (n = 17) in duration. Most copulations (29/35, 82.9%) were observed between Day -4 and Day +1 from the preovulatory oestrogen conjugates peak (Day 0). Based on known copulation dates, the mean gestation length was 48.6 +− 0.4 weeks (range 47.3-50.3 weeks). During pregnancy, urinary excretion of oestrogen conjugates increased apprx 300-fold over levels in non-pregnant mares, reaching peak concentrations by Week +24 (51% of gestation). These results demonstrate that longitudinal reproductive events, including oestrous cyclicity and pregnancy, can be monitored precisely by evaluating urinary oestrogen conjugates in samples from Przewalski’s mares maintained under semi-free-ranging conditions.52
Further studies on uses of estrogen analysis are presented below.
Serum and urinary estrone sulfate concentrations were determined in 7 pregnant mares before and after prostaglandin-induced abortion (n = 4) or surgical removal of the fetus (n = 3) to determine the source of estrogen during early pregnancy (gestation days [GD] 44 to 89). Estrone sulfate concentrations also were determined in serum samples (stored frozen for 2 years) from 3 mares that had been ovariectomized between GD 51 and 58. Estrone sulfate concentrations decreased in serum and urine after expulsion or removal of the fetus (urinary patterns were more definitive than were patterns for serum), whereas a transient decrease in serum estrone sulfate concentration was observed after ovariectomy. Seemingly, products of conception are the major source of estrone sulfate during early pregnancy, although there appears to be some ovarian contribution. Serum or urinary estrone sulfate measurements provide a simple and accurate test for fetal viability after GD 44 in the mare.53
The removal of one of twin embryos was attempted by infusion of 24% (w/v) saline into the gestation sac in 2 mares by laparotomy. The treatment was successful in one mare (Case 1) and the untreated embryo remained viable. However, neither foetus survived in the second mare (Case 2). Plasma oestrone sulphate (E1S) concentrations fell immediately after treatment in both mares but recovered to approximately 50% of pre-treatment levels in Case 1. In Case 2 plasma E1S concentrations declined steadily and were < 1 ng/ml within 6 days of treatment. These preliminary results suggest that the method may be useful for selective removal of one of twin embryos in mares. Furthermore, plasma E1S concentrations may be a useful indicator of embryonic viability.54
Two mares received PGF-2 alpha twice daily until abortion, and 2 mares received a combined treatment with oestradiol benzoate and oxytocin. The mares were about 150 days pregnant. The PG-treated animals aborted after 37 and 61 h, respectively, and the fetuses were expelled in intact fetal membranes. The other 2 mares aborted 13 and 27 h after the first oxytocin injection, respectively, and showed strong uterine contractions and expelled the fetuses in disrupted fetal membranes. Concentrations of 15-ketodihydro-PGF-2 alpha increased both after PG and oxytocin injections and in association with the abortion, but after the PG-induced abortion there was an immediate return to basal levels and after the oxytocin-induced abortion there was a large increase in the concentrations, indicating damage of the uterus. Progesterone and relaxin concentrations followed the placental function and decreased in association with the abortions. Oestrone sulphate values differed in the two groups; the oxytocin-treated animals showed a rapid decrease while the mares treated with PG showed first a marked increase and then a decrease. Concentrations of PMSG appeared to be unaffected by the abortions.55
We evaluated 2 rapid non-instrumented field tests for pregnancy on feral horses (Equus caballus) because previous techniques required sophisticated and expensive instrumentation that limited their usefulness to field researchers. The measurement of urinary estrone conjugates (E-1C) by an enzyme immunoassay and based on observable color changes was 100% accurate when compared with instrumented spectrophotometrically measured tests for E-1C. A non-instrumented “dipstick” enzyme immunoassay for equine chorionic gonadotropin-like (eCG) molecules was 83% accurate in diagnosing mare pregnancies when compared with the results of the instrumented test for E-1C. The decrease in accuracy using the non-instrumented eCG test resulted from a time period of 40-140 days when eCG was measurable, whereas E-1C elevation were measurable after day 35 of a mare’s pregnancy. Our results indicate that it is possible to detect pregnancy in free-roaming horses under field conditions and without instrumented assays; such field tests provide opportunities to study fecundity and fetal loss in a variety of free-roaming animals.56
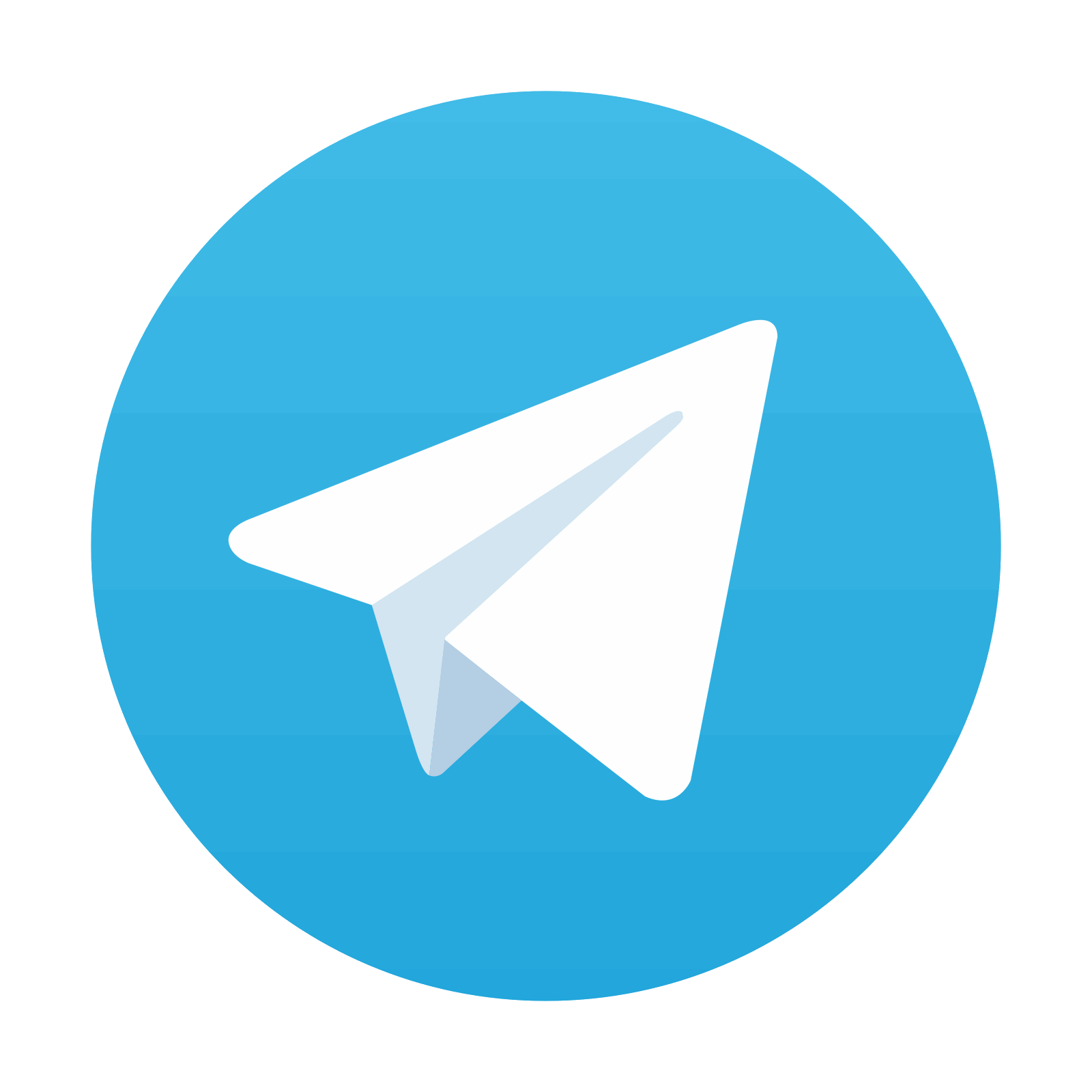
Stay updated, free articles. Join our Telegram channel

Full access? Get Clinical Tree
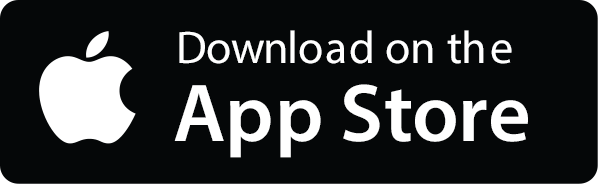
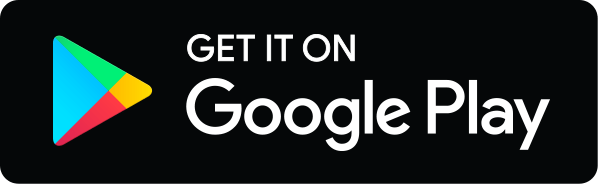