Chapter 20
Limiting Allogenic Blood Transfusions
Marie K. Holowaychuk
Critical Care Vet Consulting, Calgary, Alberta, Canada
Introduction
Most people would not question the benefit of a blood transfusion in certain clinical situations, for example the young dog that is presented after being hit by a car with a severe traumatic hemoabdomen, hypovolemic shock, and a packed cell volume (PCV) of 18%. But there are other circumstances when the risk–benefit ratio of administering a transfusion is somewhat less clear, for example the cat with chronic anemia and the same PCV of 18% that is hemodynamically stable and relatively bright. In both of these situations, veterinarians are required to make a decision as to whether or not to recommend a blood transfusion, while considering what is in the best interests of the patient. The decision whether or not to transfuse patients like the cat that are relatively stable and chronically anemic is difficult for many veterinarians due to the fear of transfusion-related complications, lack of readily available blood products, or an absence of resources needed to ensure that the transfusion is safe, such as pre-transfusion screening. Regardless of these factors, given the increasing evidence in veterinary medicine documenting transfusion-related complications and the concern regarding the risks associated with unnecessary or liberal transfusions, veterinarians should continue to question the necessity of transfusions in their patients and consider strategies to limit or avoid allogenic blood transfusions.
Paradigm shift in human transfusion medicine
There has been a paradigm shift in the field of human transfusion medicine during recent years, which has moved clinicians towards more conservative transfusion triggers, using blood alternatives, and reducing blood loss as ways to limit or avoid allogenic blood transfusions. This shift has arisen from the expanding knowledge within the human transfusion literature documenting that unnecessary transfusions occur and that there are inherent risks involved with any transfusion (Shander et al. 2011). While risks such as infection transmission and transfusion reactions are mostly mitigated by appropriate blood donor and recipient screening, other transfusion-related complications (e.g., transfusion-related immunomodulation) are less clear with regards to how they might be prevented, aside from avoiding transfusions altogether. There are several studies that have demonstrated that transfused human patients have increased morbidity and mortality compared to their non-transfused cohort, but whether it is the underlying disease that necessitates the transfusion or the transfusion itself that worsens the outcome remains unclear (Isbister et al. 2011).
Other factors that have led physicians to seek out alternatives to allogenic blood transfusions include the management of patients that refuse transfusions for religious reasons and the concern regarding whether blood product supplies will continue to meet the demands of an aging human population (Drackley et al. 2011). The increased understanding of storage lesions, which are changes in blood products during storage that likely contribute to transfusion-related complications (see Chapter 11), is an additional incentive to seek out alternatives and limit transfusions. It is possible that the knowledge gathered from research regarding storage lesions and the safety of stored blood products will lead to new guidelines regarding the shelf-life of stored blood, thus further limiting the human blood supply. It is because of these issues that physicians are practicing “bloodless medicine” in an effort to avoid allogenic transfusions whenever possible, thereby reducing the costs and consequences of unnecessary and potentially harmful transfusions.
Patient blood management
In human medicine, patient blood management (PBM) is a relatively new concept initially proposed to provide treatment for patients who were unwilling or unable to receive transfusions. However, recently it has adopted a broader application with the goal of restricting or eliminating the administration of blood products in order to conserve the blood supply and avoid unnecessary transfusions that might put patients at risk of transfusion-related complications. PBM is employed to maintain hemoglobin concentrations, optimize hemostasis, and minimize blood loss to reduce the need for allogenic transfusions and with the ultimate aim to improve patient outcome (Goodnough and Shander 2012).
More than one-third of human surgery patients demonstrate a pre-operative anemia, which is the most important risk factor for transfusion in people (Musallam et al. 2011). As such, the detection, assessment, and treatment of pre-operative anemia are required in order to minimize the administration of allogenic blood products (Goodnough et al. 2011). Accordingly, PBM was originally intended for the care of elective surgery patients, but the same concepts can be applied to all patients with the potential to require a blood transfusion during their care including non-elective surgery patients, trauma patients, oncology patients, and patients experiencing non-surgical bleeding (Shander and Goodnough 2013).
The approach to PBM relies on input from a multidisciplinary team, including the surgical, medical, anesthetic, and nursing care teams. The main focus of PBM is to identify and manage the risk factors predisposing patients to receiving a blood transfusion, which are typically the existence of anemia, degree of blood loss, and the lack of reliance on evidence-based guidelines to determine when a blood transfusion should be given (Gombotz et al. 2007). PBM uses three main strategies to manage these risk factors: (1) optimizing hematopoiesis, (2) minimizing blood loss and bleeding, and (3) optimizing physiologic adaptation to anemia while implementing appropriate therapies (Shander and Goodnough 2013). When used in combination, these strategies are intended to mitigate or avoid the administration of allogenic blood products to anemic patients.
Optimizing hematopoiesis
Given the association between anemia and worse outcomes in surgical and critically ill human patients, it follows that identification and correction of the anemia would improve outcomes. Guidelines adapted by the Network for Advancement of Transfusion Alternatives (NATA), a multidisciplinary panel, suggest measuring hemoglobin as early as 28 days before any elective orthopedic surgeries. This allows time for the diagnosis and treatment of anemia, with the goal of identifying the underlying cause and normalizing the hemoglobin prior to surgery (Goodnough et al. 2011). This concept can be applied to any patient undergoing an elective surgical procedure that might result in a large volume of blood loss. Ideally, any elective procedure should be postponed until the anemia is corrected to avoid putting patients at risk of needing a perioperative blood transfusion (Shander and Goodnough 2013).
Algorithms have been proposed to investigate anemias in human patients and typically involve assessment of iron status, kidney function, nutritional deficiencies, and the presence of concurrent chronic diseases. Treatment strategies are aimed at the underlying cause and can include supplementation of iron (intravenous or oral), folic acid, or vitamin B12 (Shander and Goodnough 2013). Erythropoiesis stimulating agents are also sometimes used, but can increase the risk of complications such as thromboembolism (Stowell et al. 2009). These PBM strategies can also be applied to oncology, medical, or critically ill patients to reduce the risks associated with administration of allogenic blood transfusions.
Minimizing blood loss
An important point to remember with PBM is that the patient’s own blood is a limited resource that must be conserved whenever possible. Patients can lose blood for various reasons, including underlying conditions (e.g., gastrointestinal hemorrhage), iatrogenic causes (e.g., excessive blood draws), and surgical bleeding. A detailed history is imperative in uncovering less obvious causes of blood loss that might be discovered when prior medical concerns and current or previous medications are disclosed (Shander and Goodnough 2013). Importantly, noting any medications that can interfere with hemostasis (e.g., acetylsalicylic acid) or that are given for their anticoagulant properties (e.g., warfarin, heparin) is essential as these patients might be at increased risk of blood loss during medical or surgical procedures. Discontinuation or reversal of these medications might be required prior to undergoing any invasive planned procedure (Levi et al. 2011).
Iatrogenic blood loss
Hospitalized patients are frequently subjected to numerous blood draws to allow various diagnostic tests to be performed. Especially in smaller patients, these blood draws can quickly add up to clinically significant blood loss, resulting in iatrogenic anemia. A large multicenter study including over 17,000 non-anemic human patients with myocardial infarction found that 20% of these patients developed anemia during their hospitalization and the risk of anemia increased by 15% for every 50 mL of blood drawn (<1 mL/kg for an average-sized adult). Interestingly, the mean total phlebotomy volume varied significantly among the hospitals studied (Salisbury et al. 2011). To avoid inducing iatrogenic anemia, “standing” orders for laboratory tests without the potential to affect treatment decisions should be avoided. The timing of diagnostic tests should also be consolidated to minimize the frequency and volume of blood draws. Likewise, small volume blood draws utilizing pediatric-sized tubes to collect blood samples should be used whenever possible (Shander and Goodnough 2013).
Surgical blood loss
Avoiding or minimizing surgical blood loss is another important part of PBM. Strategies to facilitate this include planning and rehearsing the surgery to reduce surgery times, use of less or minimally invasive approaches, use of tourniquets, positioning the patient to reduce local blood flow to the surgical site, and avoiding unnecessary hypothermia. In addition, achievement of careful hemostasis and use of electrocautery (Figure 20.1) or harmonic scalpels and other hemostatic surgical tools, instead of traditional scalpels, can allow for a more “bloodless” surgery (Nelson and Fontenot 1995; Shander and Goodnough 2013).

Figure 20.1 Monopolar electrocautery handles. Monopolar electrocautery is grounded beneath the patient and is more effective for blood vessel cauterization, but also results in more collateral damage to tissues compared to bipolar electrocautery, which is grounded within the hand piece and results in minimal peripheral heat and necrosis.
(Image provided by Michelle L. Oblak, DVM, DVSc, Dipl. ACVS-SA.)
Topical dressings, sealants, and adhesives are increasingly available and can reduce intra-operative hemorrhage via mechanical blockage, promotion of thrombosis, or inhibition of fibrinolysis. Specifically, topical hemostatic agents applied in liquid, gel, or powder form are intended to achieve a local hemostatic effect, while minimizing systemic side effects (Goodnough and Shander 2013). Unfortunately, due to the relatively recent availability of many of these products, there is limited published research to indicate that they result in reduction of allogenic transfusions, despite evidence that they significantly reduce surgical blood loss (Carless et al. 2003).
Various systemically administered hemostatic agents are being increasingly investigated in people to determine their utility in reducing or preventing blood loss in surgery patients. Some of these agents, such as prothrombin complex concentrations, single factor concentrates, and recombinant coagulation factors, are already approved for the treatment of inherited coagulopathies, specific factor deficiencies, and some acquired factor deficiencies (e.g., warfarin reversal) in people. Additionally, desmopressin is typically used to ameliorate hemostatic derangements in patients with hemophilia A or type I von Willebrand disease and has shown a modest decrease in perioperative bleeding, especially in patients with platelet dysfunction (Crescenzi et al. 2008; Ozier and Bellamy 2010). Other agents include antifibrinolytics such as aprotinin, ϵ-aminocaproic acid (EACA), and tranexamic acid (TXA), which inhibit the pathways responsible for limiting clot formation or dissolving clots. When compared to placebo, these agents have been shown to reduce the need for red blood cell (RBC) transfusions in the perioperative period by approximately 20–40% (Henry et al. 2011). However, the most promising agent, TXA, has been associated with an increased risk of neurologic complications such as seizures, despite reductions in mortality (Ngaage and Bland 2010).
Calculation of allowable blood loss
Prior to surgeries when blood loss is anticipated, calculation of “allowable blood loss” and predetermination of transfusion criteria based on hemoglobin concentration or volume of blood loss is recommended so that decisions can be made during surgery without haste and based on careful consideration (Nelson and Fontenot 1995). For example, depending on the pre-surgery hemoglobin, a patient can safely lose approximately 10–20% of his/her blood volume, which is calculated by multiplying the body weight by the mL/kg blood volume that varies by species. Human patients have a blood volume, whereas dogs and cats have
and
estimated blood volumes, respectively. As such, a 20-kg dog could potentially lose up to 360 mL of blood during a surgical procedure yet possibly avoid a blood transfusion.
Viscoelastic testing to guide blood product administration
The use of viscoelastic testing such as thromboelastography (TEG) (Figure 20.2) and rotation thromboelastometry (ROTEM) has increased in recent years and provides a more accurate depiction of whole-blood hemostasis compared to other conventional methods of coagulation testing. Interestingly, human studies have shown that the use of TEG- or ROTEM-guided transfusion protocols have reduced the number of allogenic transfusion, especially administration of plasma products, during surgeries with a large propensity for blood loss and blood product usage, including aortic surgery and liver transplantation (Girdauskas et al. 2010; Wang et al. 2010).

Figure 20.2 Two thromboelastography machines, which are used to measure whole-blood coagulation, producing results that enable assessment of clotting factor activity, platelet function, clot strength, and fibrinolysis.
Optimizing physiologic adaptation to anemia
When a patient develops anemia, the body responds with several physiologic adaptations to maintain the balance between oxygen supply and demand. These adjustments include increased ventilation, hemoglobin oxygen saturation, and cardiac output, decreased systemic vascular resistance, alterations in local blood flow, increased tissue oxygen extraction, and cellular metabolic changes. As part of PBM, treatment strategies can be employed to support these adjustments to reduce the immediate negative effects of the anemia with the ultimate goal of reducing or avoiding allogenic blood transfusions. This can be achieved by providing supplemental oxygen, maintaining normovolemia and adequate perfusion, and avoiding tachycardia, hyperthermia, and other conditions associated with increased oxygen demand (Shander and Goodnough 2013). Tolerance of anemia decreases during hypotension, increased depth of anesthesia, fever, sepsis, and tissue trauma, whereas tolerance increases during neuromuscular blockade and hypothermia (Pape and Habler 2007).
Autologous transfusion
Another way to reduce the need for allogenic transfusions in surgery patients is to perform autologous transfusion techniques, including pre-operative autologous donation (PAD), acute normovolemic hemodilution (ANH), and cell salvage (CS) techniques. These techniques tend to be used in patients undergoing surgery during which substantial blood loss is anticipated, in patients unable to receive allogenic transfusions for religious reasons (e.g., Jehovah’s witness), or in patients with documented alloantibodies that make them poor candidates for allogenic blood transfusions (Spahn and Goodnough 2013; Shander and Goodnough 2013). Each of these techniques has advantages and disadvantages, which are summarized in Table 20.1 and described in more detail below.
Table 20.1 Comparison of autologous transfusion techniques used to reduce allogenic blood product use (Shander and Goodnough 2013)
Autologous transfusion techniques | |||
Key features | PAD | ANH | CS |
Timing of blood collection | 4–6 weeks before surgery | Immediately before surgery | During or after surgery |
Type of surgical procedure | Elective | Elective or urgent | Elective or urgent |
Blood collection method | Phlebotomy | Phlebotomy | Retrieval of shed blood from body cavity or via drains |
Replacement of collected blood | None | Colloids or crystalloids to achieve normovolemia | None |
Type of infused blood | Stored whole blood | Fresh whole blood | Washed or filtered and re-suspended red blood cells |
Possibility of storage lesions | Yes | Minimal | Minimal |
Possibility of transfusion error | Yes | Minimal | Minimal |
Possibility of wasted blood | Yes | Unlikely | No (collected blood otherwise lost) |
ANH, acute normovolemic hemodilution; CS, cell salvage; PAD, preoperative autologous transfusion.
Pre-operative autologous donation
PAD is occasionally performed in human patients undergoing elective surgical procedures when the hemoglobin is , anticipated blood loss is >500–1000 mL (10–20% of blood volume), and the likelihood of needing an allogenic transfusion is anticipated to be >50% (Vamvakas and Pineda 2000). This procedure involves the collection of 1 unit of whole blood 4–6 weeks prior to the scheduled surgery. The blood is separated into packed red blood cells (PRBCs) and plasma, labeled and allocated to the patient, and stored until re-infusion if needed during the surgical procedure. The use of PAD has been declining in recent years due to inconvenience to the patient, cost to the healthcare system (i.e., collection, processing, and storage), risk of clerical error (mistaken transfusion) and contamination, and concern regarding storage lesions (Pape and Habler 2007; Shander and Goodnough 2013).
Unfortunately, patients undergoing PAD are often anemic at the time of surgery secondary to the blood collection, which decreases their tolerance of bleeding and predisposes them to needing more frequent transfusions. Likewise, if surgeries are canceled or rescheduled, sometimes blood that was collected ahead of time has to be discarded because it has expired (Thomas et al. 2013). In patients undergoing multiple phlebotomies or donations, supplementation with erythropoietin (EPO), iron, folate, and vitamin B12 is often required; therefore, the procedure is not cost-effective if only 1 unit of allogenic blood is needed during the surgery or >15% of collected blood is discarded (Habler and Messmer 1997). Ultimately, a meta-analysis indicated a higher overall transfusion rate in patients undergoing PAD, likely secondary to pre-operative anemia (Carless et al. 2004), and therefore this technique has mostly fallen out of favor in human medicine (Thomas et al. 2013).
Acute normovolemic hemodilution
ANH is a relatively simple and low-cost autologous transfusion technique that is also used in surgical patients with a large volume of expected blood loss such as during cardiac, orthopedic, hepatic, and urogenital procedures (Monk and Goodnough 1998). It involves the removal of whole blood immediately before surgery; typically, 3–4 units of blood are collected (maximum 3 L per person) to target a patient hemoglobin concentration of . Immediately after, euvolemia is restored with crystalloids (3:1 ratio to whole blood volume collected) and/or colloids (1.1:1 ratio). The blood is stored as fresh whole blood in the operating room at room temperature and then returned to the patient when the surgical procedure is complete, if further blood loss is expected to be minimal, if the patient becomes hemodynamically unstable, if the intraoperative hemoglobin becomes
, or if the procedure exceeds 8 hours (Maithel and Jarnagin 2009).
Theoretically, ANH ensures that any blood lost during surgery will have a lower number of red blood cells and clotting factors compared to the blood returned to the patient, thereby reducing the actual amount of blood loss. However, it is important that patients chosen to undergo ANH have suitable pre-operative hemoglobin concentrations () and sufficient blood loss anticipated such that the cost of the procedure is considered worthwhile. The benefits of ANH compared to PAD include that ANH can be performed prior to urgent or elective surgeries, there are no storage or processing costs, there is minimal risk of clerical error or contamination, and collected units are rarely wasted (Maithel and Jarnagin 2009; Shander and Goodnough 2013). However, evidence supporting the ability of ANH to reduce allogenic transfusions is inconsistent among studies (Bryson et al. 1998; Segal et al. 2004), with prospective studies showing more benefit in patients with substantial blood loss (e.g., major liver resection) (Matot et al. 2002; Jarnagin et al. 2008). Likewise, the safety of hemodilution is not completely known and might precipitate cardiac ischemia or myocardial infarction (Thomas et al. 2013).
Cell salvage
CS involves the recovery of a patient’s own shed blood either intraoperatively from the surgical field or sponges, or postoperatively via drains and is typically used when blood loss is anticipated to be >500 mL (10% of blood volume in people). The shed blood is collected, washed, filtered, and reinfused back to the patient using specialized equipment (Shander and Goodnough 2013; Thomas et al. 2013) (Figure 20.3). CS has the benefit of enabling conservation of blood that would otherwise be lost and is efficacious in reducing allogenic blood transfusions in people (Carless et al. 2010). However, some controversy exists regarding the loss of coagulation factors during the washing process, hemolysis induced by collection and processing, and the possible increased risk of introducing unwanted cells (e.g., tumor cells) or contaminants (e.g., bacteria) into the bloodstream. Some of these concerns seem to be mitigated by the use of leukocyte reduction filters in CS devices (Esper and Waters 2011). This procedure is discussed in more detail in Chapter 21.

Figure 20.3 Cell salvage device
(Electa, Sorin Group USA, Arvada, CO).
Transfusion triggers
Transfusion algorithms have been designed to ensure that allogenic transfusions are only administered to patients under certain circumstances, such as moderate to severe anemia or blood loss, so that unnecessary transfusions can be avoided (Enriquez and Shore-Lesserson 2009). However, some controversy exists regarding the hemoglobin concentration that should be minimally acceptable in patients prior to giving a RBC transfusion or what degree of blood loss is considered acceptable during surgical procedures. For patients that are actively bleeding, a transfusion is usually not required if blood loss is <15% of blood volume, but it could be considered if the blood loss were to increase to 15–30%. In patients with blood loss 30–40% of blood volume, a transfusion is usually necessary and in patients whose blood loss exceeds 40%, a transfusion is absolutely indicated (Thomas et al. 2013).
The “critical hemoglobin” or hemoglobin concentration below which oxygen delivery is insufficient to meet oxygen demand varies among species and patients. In people, it is approximately and in anesthetized dogs it is
(Cain 1977). This is considered the lowest acceptable hemoglobin concentration to meet tissue oxygen demands and if not treated could lead to death within hours (Pape and Habler 2007). In the past, RBC transfusions were given according to the 10/30 rule (hemoglobin
and hematocrit [HCT] 30%). However, this has largely been abandoned in favor of more restrictive transfusion strategies. In general, it is advised to transfuse people whose hemoglobin is
, whereas transfusions are rarely recommended in patients with a hemoglobin
(Thomas et al. 2013).
Restrictive versus liberal transfusion strategies
There is a growing body of evidence in the human medical literature investigating the benefits of conservative transfusion strategies, which utilize lower hemoglobin thresholds to trigger RBC transfusions. Studies show that hospitals with restrictive transfusion strategies administer fewer transfusions to elective surgery patients without any adverse effects on outcome (So-Osman et al. 2013; Fan et al. 2014). Conversely, hospitals with liberal transfusion practices that transfuse non-cardiac surgical patients with higher hemoglobin concentrations have higher 30-day mortality rates and increased adverse postoperative outcomes (Abdelsattar et al. 2015). However, liberal blood transfusion practices do not affect mortality risk in elderly patients with underlying cardiovascular disease, thus questioning the hypothesis that blood transfusions lead to long-term immunosuppression that is severe enough to affect mortality rate in all patient populations (Carson et al. 2015).
A meta-analysis evaluating 19 prospective randomized trials comparing restrictive (transfusing when hemoglobin ) versus liberal (transfusing when hemoglobin
) transfusion strategies documented that adhering to a restrictive transfusion strategy reduces the risk of receiving a RBC transfusion by 39% and reduces the volume transfusion by 1.2 units. However, restrictive strategies did not appear to impact the rate of adverse events or 30-day mortality compared to liberal strategies (Carson et al. 2012). Another meta-analysis included 31 trials and also found that the number of patients receiving RBC transfusions, as well as the number of units transfused, were lower with the restrictive group (transfused if hemoglobin 7.0–9.7 g/dL [70–97 g/L], HCT 24–30%) compared to the liberal group (transfused if hemoglobin
, HCT 30–40%). However, mortality, overall morbidity, and other complications such as myocardial infarction were not associated with the transfusion strategy used (Holst et al. 2015). As such, tolerance of a lower hemoglobin threshold is recommended prior to considering a RBC transfusion and liberal transfusion strategies are unlikely to have any benefit in most patients.
Transfusion targets
In addition to considering when a patient should be transfused, the target hemoglobin for the transfusion administered should also be used to calculate the amount of blood products needed. Specifically, the dose of blood given is calculated from the patient’s current hemoglobin (or PCV), target hemoglobin, body weight, and blood volume. This is done in an effort to minimize the amount of allogenic blood products administered and conserve blood products, rather than simply transfusing based on a unit per patient basis. Given the studies documenting the survival advantages of maintaining hemoglobin at 7–9 g/dL (70–90 g/L), the maximum hemoglobin that should be targeted is 9 g/dL (90 g/L) or an approximate PCV of 27%. Calculations used to determine the amount of RBC products needed to achieve patient PCV targets are described elsewhere (see Chapter 3).
Applications in veterinary patients
The shift in mindset amongst veterinarians towards more conservative use of blood products is less widespread, but has started in recent years. This could be due to increasing concerns regarding storage lesions (Obrador et al. 2015) and the association between administration of older RBC products to canine patients and increased complications, including coagulopathies and thromboembolic disease (Hann et al. 2014). Occasionally, veterinary studies have documented an association between administration of blood products and increased morbidity risk, such as postoperative pulmonary complications (Alwood et al. 2006) or surgical site dehiscence (Ralphs et al. 2003). Likewise, a retrospective study investigating transfusion-related complication in dogs receiving allogenic transfusions found that administration of larger doses of PRBCs and a higher pre-transfusion PCV were risk factors for non-survival (Holowaychuk et al. 2014). Restrictive transfusion strategies therefore might also have the same beneficial effects in veterinary patients as in people.
Regardless of these findings, the concept of PBM has not yet been proposed officially in the veterinary literature. Despite that, several of the PBM concepts have been or could easily be applied to veterinary patients, such as optimizing hematopoiesis by treating the underlying cause of the anemia, as well as optimizing physiologic adaptation to anemia as is done in people. A study investigating the incidence of iatrogenic anemia in 45 critically ill cats hospitalized for more than 48 hours recorded that 74% of non-anemic cats developed anemia during their hospitalization. The number of phlebotomies ranged from one to six per day and a higher number of phlebotomy procedures was associated with a higher need for transfusions, which was associated with a longer duration of hospitalization. Cats that were given a transfusion also had a higher volume of blood drawn (3.3 mL/kg per intensive care unit stay) compared to cats that did not receive a transfusion (1.1 mL/kg per intensive care unit stay) during their hospitalization. More than 80% of cats included in the study had sampling catheters to facilitate phlebotomy procedures (Balakrishnan et al. 2016).
Methods to minimize blood loss are therefore needed in veterinary patients and easily applicable from human medicine. For example, veterinary technicians can be trained to minimize blood draw volumes by using pediatric blood collection tubes for the submission of necessary laboratory tests to reduce iatrogenic blood loss. Likewise, sampling catheters can be used to obtain small volume samples, with efforts made to return catheter purge samples to the patient. Additionally, calculation of allowable blood loss by determining the amount equal to 20% of the patient’s blood volume could easily be performed during pre-operative planning (Box 20.1), as well as calculation of the amount of blood that might be needed should a transfusion be indicated (see Chapter 3).

Full access? Get Clinical Tree
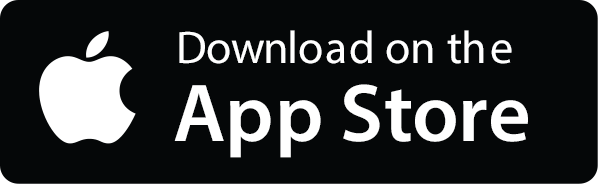
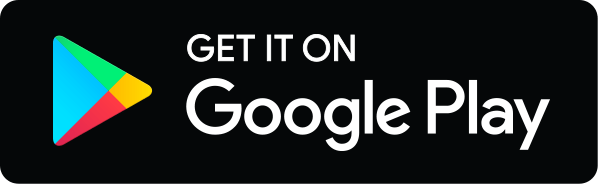