Roberto M. C. Guedes Fabio A. Vannucci and Connie J. Gebhart Lawsonia intracellularis is the sole species in the genus Lawsonia. It is the etiologic agent of proliferative enteropathy, an infectious, intestinal hyperplastic disease characterized by thickening of the mucosa of the intestine due to enterocyte proliferation (McOrist and Gebhart 2006; Figure 22.1). L. intracellularis is an obligately intracellular, curved‐shaped bacterium found in the apical cytoplasm of infected enterocytes. This bacterium infects epithelial cells from the intestinal overlayer and prevents immature cell differentiation, resulting in enterocyte proliferation in vivo (Lawson and Gebhart 2000). Although the disease in pigs was first reported in 1931 (Biester and Schwarte 1931), it was not until the early 1970s that Lawson’s research group in the United Kingdom began studying field outbreaks of proliferative enteropathy (Lawson and Gebhart 2000). The early description of the disease was based on gross and histologic features followed by demonstration of its reproducibility (Biester and Schwarte 1931). Rowland et al. (1973) detected intracellular bacteria in proliferative lesions by use of immunofluorescence, probing affected tissue with hyperimmune serum from an affected pig. Roberts et al. (1977) demonstrated that lesion material contained the infectious agent by experimentally infecting pigs with homogenates of affected intestine. However, it was not until the 1990s that this intracellular bacterium was isolated from hamsters (Stills 1991), pigs (Lawson et al. 1993), and horses (Cooper 1996), and cultivated in vitro. The disease was then reproduced in pigs (McOrist et al. 1993) and horses (Al‐Ghamdi 2003) with pure cultures. The causative intracellular agent of proliferative enteropathy was determined to be of a new genus and species (Gebhart et al. 1993) and was named L. intracellularis in honor of Dr. Lawson (McOrist et al. 1995a). L. intracellularis is a small, Gram‐negative, curved rod that is 1.25–1.75 μm in length and 0.25–0.43 μm in width. The bacterium has a trilaminar outer envelope, which is frequently separated from the cytoplasmic membrane by an electronlucent zone. No fimbriae or spores have been detected. Extracellular, cell culture‐grown isolates have a long, single, unipolar flagellum (Lawson and Gebhart 2000; Figure 22.2), and the organism has a darting motility in vitro upon escape from infected enterocytes. L. intracellularis divides transversely by septation, and organisms are located free within the apical cytoplasm of infected enterocytes (Gebhart et al. 1993). Strict environmental conditions are required for cultivation of L. intracellularis in vitro. Cell‐culture systems were used in early attempts to isolate the bacterium due to its intracellular location in vivo (Figure 22.3). Growth of L. intracellularis in vitro requires dividing eukaryotic cells and an atmosphere of 82.2% nitrogen, 8.8% carbon dioxide, and 8% oxygen (Lawson et al. 1993). Alternative chambers, such as sealable bags, providing similar microaerophilic atmospheres or suspension cultures have been used for small‐ or large‐scale cultivation and production of vaccines, respectively (Vannucci et al. 2012c). These suspension cultures are propagated in spinner flasks or bioreactors with automatized agitation and monitoring of temperature and gas mixture. The peak of the infection, where 80–90% of the cells are infected with more than 30 bacteria in the cytoplasm of each cell, occurs between five and seven days post‐inoculation (Lawson and Gebhart 2000). Figure 22.1 Ileal section of a pig infected with Lawsonia intracellularis. Hematoxylin and eosin stain of hyperplastic crypts. Figure 22.2 Transmission electron microscopy photograph of Lawsonia intracellularis cells depicting the presence of a single polar flagellum. Since it is not possible to cultivate L. intracellularis in conventional bacteriological media, the final classification was done by molecular taxonomic methods. Using 16S ribosomal DNA (rDNA) sequence analysis, Gebhart et al. (1993) showed that sequences obtained from organisms purified, without cultivation, from the ileal mucosa of multiple pigs were 91% similar to those of Desulfovibrio desulfuricans. However, the physiological and biological features of the organism were sufficiently unique for it to be considered a new genus. Its closest genetic relative is Bilophila wadsworthia (92% 16S rDNA similarity), a free‐living anaerobic human pathogen (Sapico et al. 1994). L. intracellularis is classified in the delta subdivision of Proteobacteria (Gebhart et al. 1993). Although this bacterium replicates within eukaryotic cells, similar to Rickettsiae and Chlamydiae, it is taxonomically distinct from these and other intracellular pathogens (McOrist et al. 1995a; Dale et al. 1998; Schmitz‐Esser et al. 2008). This intracellular bacterium, previously known as a Campylobacter‐like organism, Ileal symbiont intracellularis, and Ileobacter intracellularis, was established in a new genus as L. intracellularis (McOrist et al. 1995a). Figure 22.3 Cell culture of intestinal 407 cells infected with Lawsonia intracellularis. Only a few cells are heavily infected. Immunoperoxidase stain using L. intracellularis‐specific monoclonal antibody. Source: Vannucci and Gebhart (2014). The whole genome of a porcine L. intracellularis isolate (PHE/MN1‐00) was sequenced and annotated, revealing a total of 1 719 014 base pairs distributed into one small chromosome and three plasmids (accession: PRJNA183). High levels of expression of numerous bacterial genes encoding hypothetical proteins during L. intracellularis infection both in vitro and in vivo suggests that this organism has adopted heretofore uncharacterized mechanisms of survival and pathogenesis. Phenotypical characterization, by immunoblotting of outer membrane proteins, and conventional molecular characterization methods of the L. intracellularis genome demonstrate minor differences among isolates from different host species and no strain differences within host species (Al‐Ghamdi 2003; Guedes and Gebhart 2003c). Recent metagenomic sequencing and phylogenetic analysis study of clinical samples from pigs and horses have demonstrated a genetically monomorphic clonal lineage responsible for infections in pigs, with distinct subtypes associated with infections in horses (Bengtsson et al. 2020). This single major lineage associated to the worldwide distribution of this agent suggests its recent emergence and clonal expansion. Proliferative enteropathy can be reproduced using pure cultures of L. intracellularis at low passages (4–20) in cell culture (McOrist et al. 1993; Guedes and Gebhart 2003a, 2003b; Vannucci et al. 2013a). No disease was demonstrated in pigs experimentally infected with a porcine isolate passed 40 times in vitro, indicating that attenuation occurs between passages 20 and 40. The loss of pathophysiological properties of L. intracellularis associated with adaptation to in vitro conditions has been speculated. However, DNA‐based typing techniques, such as variable number tandem repeat, have shown identical genotypes in homologous isolates at low and high passages (Pusterla and Gebhart 2013; Vannucci et al. 2013a). Proliferative enteropathy has been reported in a broad range of hosts, including pigs, non‐human primates, hamsters, rabbits, rats, guinea pigs, foals, sheep, white‐tailed deer, ferrets, arctic foxes, dogs, and certain birds (Lawson and Gebhart 2000; Vannucci et al. 2019). L. intracellularis infection has been established experimentally in mice deficient in interferon‐gamma receptors (Smith et al. 2000), in both inbred and outbred mice (Viott et al. 2013) and has occurred spontaneously in conventional mice (Guedes 2008). There are no reports of proliferative enteropathy in human beings, but cases have been reported in non‐human primates (Klein et al. 1999). The source of infection for these animal species has not been determined. It may be endemic in certain species, or it may be present in the environment. Free‐living animals serve as the reservoir host and are involved in the transmission of L. intracellularis between affected animals or from the natural environment (Pusterla et al. 2008). Species‐to‐species transmission has been documented. Pig isolates infect hamsters (McOrist and Lawson 1987) and mice (Smith et al. 2000; Viott et al. 2013; Gabardo et al. 2017). However, cross‐species transmission of proliferative enteropathy usually results in subclinical, mild infections and, therefore, some host specificity for infection seems to exist. Horses challenged with a pig L. intracellularis strain, and vice versa, induced milder clinical signs, shorter fecal shedding, and weaker seroconversion when compared with species‐specific isolates (Vannucci et al. 2012b). Hamsters are a well‐published animal model for porcine proliferative enteropathy infection studies, and species specificity has been demonstrated for the disease in pigs and horses (Vannucci et al. 2012b). Hamsters challenged with an equine isolate of L. intracellularis do not develop lesions, whereas hamsters challenged with a pig isolate do develop disease. Conversely, rabbits challenged with a pig isolate do not develop typical intestinal lesions, whereas challenge with an equine isolate does induce typical lesions in rabbits (Sampieri et al. 2013). Feces from infected pigs may be the source of new infections for susceptible pigs (McOrist and Gebhart 2006), and pig‐to‐pig contact is an important route of transmission (Vannucci et al. 2019). Mice infected with pig isolates shed and infect susceptible pigs, and pigs infected with pig isolates shed and infect mice (Gabardo et al. 2017). Other possible mechanisms of transmission include mechanical vectors, such as rubber boots, and biological vectors, such as small birds, and insects. Isolation or re‐isolation of viable organisms from diseased intestines is not easily achieved due to the difficulty in extraction, growth, and maintenance of L. intracellularis in vitro. Therefore, information about the survival and resistance of the organism in the environment is scarce. Intestinal colonization of pigs by L. intracellularis was detected after oral inoculation with feces from infected animals, which had been stored for up to two weeks at 5°C or 15°C (Collins et al. 2000). The presence of intestinal microbiota modifies the ability of L. intracellularis to colonize the intestinal tract and cause proliferative lesions. Germ‐free pigs are not susceptible to infection by cell culture‐purified L. intracellularis (McOrist et al. 1994). However, pigs exposed to intestinal material from L. intracellularis‐infected animals develop the disease (McOrist and Lawson 1989). Gnotobiotic pigs inoculated with cultured, normal intestinal microbiota along with cell‐cultured L. intracellularis also develop typical lesions of proliferative enteropathy (McOrist et al. 1994). This may be the result of provision of a microaerobic environment supportive of the pathogen. Diet may influence infection in hamsters (Jacoby and Johnson 1981). Molbak et al. (2008) used a culture‐independent approach based on the terminal restriction fragment length polymorphism technique to demonstrate that non‐pelleted diet reduced the relative amount of L. intracellularis in the total microbiota of the ileum. However, the number of animals shedding the bacteria in the feces was not influenced. The reduction in the relative amount of L. intracellularis in the ileum of these pigs might have been related to a change in the intestinal environment, such as lower pH, different microbiota, and/or different intestinal morphology (Molbak et al. 2008). The presence of intestinal microbiota modifies the ability of L. intracellularis to colonize the intestinal tract and cause proliferative lesions. Germ‐free pigs are not susceptible to infection by cell‐culture‐purified L. intracellularis (McOrist et al. 1994). However, pigs exposed to intestinal material from L. intracellularis‐infected animals develop the disease (McOrist and Lawson 1989). Gnotobiotic pigs inoculated with cultured, normal intestinal microbiota bacteria, together with cell‐cultured L. intracellularis also develop typical lesions of proliferative enteropathy (McOrist et al. 1994). Diet may influence infection in hamsters (Jacoby and Johnson 1981). Molbak et al. (2008) used a culture‐independent approach based on the terminal restriction fragment length polymorphism technique to demonstrate that non‐pelleted diet reduced the relative amount of L. intracellularis in the total microbiota of the ileum. However, the number of animals shedding the bacteria in the feces was not influenced. The reduction in the relative amount of L. intracellularis in the ileum of these pigs might have been related to a change in the intestinal environment, such as lower pH, different microbiota, and/or different intestinal morphology (Molbak et al. 2008). Proliferative enteropathy has been reported in numerous and varied animal species (Vannucci et al. 2019) but has been best described in pigs, hamsters, and horses. Various names have been used, including proliferative enteritis, porcine intestinal adenomatosis (PIA), proliferative hemorrhagic enteropathy (PHE), ileitis, and wet‐tail disease. The two major clinical forms of proliferative enteropathy in pigs are acute hemorrhagic diarrhea, sudden death of newly introduced animals and pigs close to market age, known as PHE, and chronic mild diarrhea and reduced performance in growing pigs, known as PIA (Lawson and Gebhart 2000; McOrist and Gebhart 2006). Affected hamsters have lesions comparable to the chronic form of proliferative enteropathy in pigs, with the exception that pyogranulomatous inflammation is noted in the late stages of disease (Jacoby et al. 1975). Pathological lesions in horses range from multifocal to confluent regions of mucosal hyperplasia, resulting in a protein‐losing enteropathy (Lavoie and Drolet 2007). Macroscopic lesions of proliferative enteropathy are usually located in the aboral portion of the small intestine, but can also be found from the duodenum to the rectum. Intestines affected by the acute form of porcine proliferative enteropathy (PPE) are dilated, thickened, and turgid, with a corrugated serosal surface. Formed blood clots are found in the lumen of the ileum (Vannucci et al. 2019). Chronically affected pigs usually have intestines with irregular patchy subserosal edema, mainly at the area of mesenteric insertion. The ileal mucosa is thickened with deep folds and with patches of pseudomembrane covering the mucosa. As lesions progress, mucosal destruction can lead to rapid death of animals (Vannucci et al. 2019). Hypertrophy and thickening of the muscularis mucosa may occur in animals surviving this necrotic enteritis. Histologically, acute and chronic forms of the disease have similar characteristics. Adenomatous proliferation occurs among epithelial cells in the crypts of Lieberkühn in the small intestine and mucosal glands of the large intestine, in association with the presence of curved‐shaped intracellular bacteria in the apices of these enterocytes (Rowland and Lawson 1974). The crypts are elongated, enlarged, and crowded with immature epithelial cells in a highly mitotic state. There is marked reduction or absence of goblet cells in affected areas, and infiltration of inflammatory cells is minimal (McOrist and Gebhart 2006). Intestines affected by the acute form are severely congested, with accumulation of blood in the lumen. Electron microscopic studies of experimentally infected hamsters and pigs (Johnson and Jacoby 1978; Jasni et al. 1994; Guedes et al. 2017) and naturally infected pigs (Rowland and Lawson 1974; Love and Love 1979) have shown that highly infected enterocytes usually have short, irregular microvilli. Little is known about the genetic basis for the virulence, pathogenesis, or physiology of L. intracellularis so that much of this discussion of the basis of virulence is useful speculation. Further, the molecular mechanisms for infection and virulence and the epidemiology of this organism in pigs and other species remain undetermined. Sequences in the genome of interest from a virulence standpoint include those homologous to genes encoding proteins involved in flagellar biosynthesis and assembly. The presence of a single polar flagellum in L. intracellularis also represents an important phenotypic component that potentially is involved in the early stages of infection (Smith and Lawson 2001). Flagella allow enteric pathogens to penetrate the mucous layer and reach the apical membrane of enterocytes. The whole genome sequence of a porcine L. intracellularis isolate shows various genes involved in the flagellar assembly (Vannucci et al. 2012a). As a result, this element is likely to contribute to survival or colonization, at least during extracellular stages of infection. The major pathogenic mechanism of L. intracellularis is invasion of enterocytes and induction of hyperplasia in these cells (Lawson and Gebhart 2000). Generally, no significant inflammatory response occurs, and the infection remains localized in enterocytes and, based on a recent study, likely also in macrophages (Pereira et al. 2020). Attachment and entry into immature epithelial cells occur at the apical surface. Specific adhesins or receptors for L. intracellularis have not been characterized, but attachment and entry appear to require specific bacterium‐host cell interaction (McOrist et al. 1995b). The process of invasion does not depend on L. intracellularis viability, as eukaryotic cells internalize formalin‐fixed organisms (Lawson et al. 1995; Pereira 2019). Invasion is significantly reduced by blockage of cellular metabolism and cytoskeleton rearrangement by cytochalasin D (Lawson et al. 1995). Other mechanisms of cell entry may be involved, since many cells become infected despite treatment with cytochalasin D. For instance, there was decreased internalization of nonviable L. intracellularis into IPEC‐J2 cells that had less clathrin synthesis, owing to clathrin knock down (Pereira 2019). Expression of LsaA, a gene that encodes a surface antigen, during infection in vitro and in vivo suggests that this surface antigen might be involved during infection. Phenotypic analysis indicated a role during L. intracellularis attachment to and entry into intestinal epithelial cells (McCluskey et al. 2002). L. intracellularis escapes from the membrane‐bound vacuole and lies free in the cytoplasm. In other enteric pathogens, such as Listeria, Rickettsia spp. and Shigella, the secretion of lytic toxins (cytolysins or hemolysins) is an important mechanism for escape into the host cytoplasm. Although the expression of a hemolytic protein and the cytolytic activity of L. intracellularis
22
Lawsonia intracellularis
Introduction
Characteristics of the Organism
Sources of Infection: Ecology and Epidemiology
Influence of Environmental Factors
Types of Disease and Pathologic Changes
Virulence Factors and Pathogenomics
Stay updated, free articles. Join our Telegram channel

Full access? Get Clinical Tree
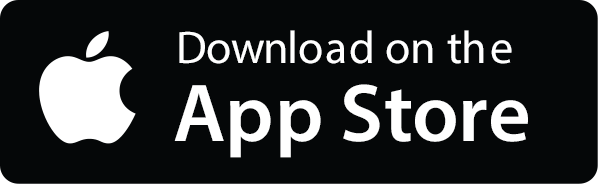
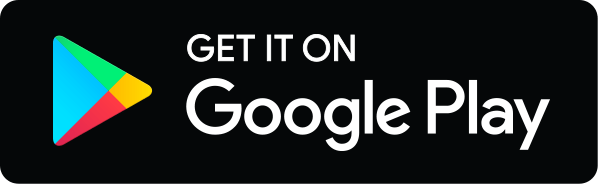