From most potent to least potent, inhalant anesthetics rank as follows: methoxyflurane, halothane, isoflurane, sevoflurane, desflurane, and nitrous oxide.
Vaporization Pressure
Concentration of inhalant anesthesia is determined by the vapor pressure. Vapor pressure is the rate at which a liquid will turn into a gas at a given temperature. Methoxyflurane has a low vaporization pressure of approximately 3% at room temperature. This allows methoxyflurane to be used in both nonprecision and precision vaporizers. Halothane, isoflurane, sevoflurane, and desflurane have vapor pressures that are over 30% at room temperature and need a precision vaporizer to deliver anesthetic to the patient (Paddleford 1999). Desflurane has a high vapor pressure and low boiling point, and it needs to have a special vaporizer to evaporate in order to be delivered to the patient (Table 15.2) (Eger et al.; Eger 1984; Paddleford, 1999).
Table 15.2. Vapor pressures of inhalant anesthetics.
Source: Eger et al. 2003.
Agent | Vapor Pressure at 20°C (mm Hg) |
Methoxyflurane | 22.8 |
Halothane | 244 |
Isoflurane | 239.5−240 |
Sevoflurane | 157−160 |
Desflurane | 664−700 |
Solubility Coefficient
The lower the solubility of the anesthetic, the more rapid gas anesthetics will go from the alveoli into the blood (i.e., gas dissolves into a liquid). If there is low solubility, this means there will be rapid induction because the gas will quickly distribute from the alveoli into the blood. During recovery from anesthesia, the anesthetic gas will be excreted out of the body. From fastest to the slowest rate of alveolar concentration anesthetics are ranked as follows: desflurane, sevoflurane, isoflurane, and halothane. Sevoflurane is twice as soluble as desflurane and nitrous oxide. Halothane is twice as soluble as isoflurane. Isoflurane is twice as soluble as sevoflurane. If delivery of the anesthetic is somehow limited, increased induction time may be seen. This can be the case in inhalant anesthetics, which are irritating and cause the patient to produce excessive saliva, hold its breath, or experience laryngospasm (Eger et al. 2003; Eger 1984). The solubility coefficient of an anesthetic is affected by increases and decreases in body temperature. When patients become hypothermic, the inhalant anesthetics become more soluble in the cooled blood, and less inhalant anesthetic is required to maintain the patient. The opposite is true for hyperthermic patients.
Inhalant anesthetic agents have the following characteristics:
- Inhalant anesthetics affect the CNS.
- The primary method of excretion of inhalant anesthesia is via respiration.
- Most inhalants decrease the rate and volume of respiration.
- Inhalants cause vasodilation in a dose-dependent manner, thereby decreasing peripheral vascular resistance (PVR) and cardiac output.
- Low blood pressure and hypothermia will result due to the vasodilation.
- The effects on heart rate and contractility are variable between agents.
- Renal and cerebral perfusion could be compromised due to vasodilation effects of inhalant anesthetics.
- Malignant hyperthermia can occur in all modern inhalants; however, it is more prevalent in halothane.
- Because some inhalant anesthetics provide only very slight analgesia, additional analgesia should be provided.
- The National Institute for Occupational Safety and Health (NIOSH) states that the principal exposure to waste gas comes from leaks in the anesthetic machine. NIOSH recommends that exposure limits for halogenated inhalant anesthetics should be less than 2 parts per million (ppm). Nitrous oxide concentration should not exceed 25 ppm, and halogenated inhalant anesthetics should not exceed 0.5 ppm if used in combination with nitrous oxide (Paddleford 1999).
- Safety concerns with the reproduction organs, liver, and kidney, and incidences of cancer need to be considered.
- Exposure of pregnant females to inhalants should be avoided, particularly in the first trimester (Thurmon et al. 1996; Paddleford 1999).
- Monitoring for exposure to waste gas should be performed periodically, and adequate scavenging of waste gas should be utilized to decrease waste gas in the areas where inhalant anesthetics are being used.
Specific Inhalants
Nitrous Oxide (N2O)
N2O is supplied in compressed gas cylinders. It does not need to be vaporized to be delivered to a patient. N2O will decrease the availability of oxygen to a patient and this can cause hypoxemia. N2O must be delivered to the patient with oxygen. The amount of N2O should not be delivered in excess of 66% so that a minimum of 33% oxygen (O2) is available to the patient (Eger et al. 2003; McKelvey et al. 2003; Thurmon et al. 1996; Paddleford 1999). Typically, N2O is used for general anesthesia by combining it with another inhalant anesthetic. This will reduce the amount of the more potent and potentially toxic inhalant anesthetics to be used (Thurmon et al. 1996).
The “second gas effect” allows for a concurrently delivered (along with the N2O) inhalant anesthetic to be delivered to the blood and tissues faster, using less of it because the N2O, in a sense, forces the concentration of the second gas. Because N2O is administered in high concentrations and taken up quickly, the second gas’s alveolar concentration increases more rapidly than when given as the sole inhalant (Thurmon et al. 1996).
At the end of anesthesia there is rapid movement of N2O out of the blood and into the lungs. This will displace oxygen and cause diffusion hypoxia. The patient must be left on 100% oxygen for at least 5 to 10 minutes to prevent diffusion hypoxia (Thurmon et al. 1996; Paddleford 1999).
Closed gas pocket disease can occur with the use of N2O in the case of a closed anatomic structure that can expand but have no escape for the gas to release if it builds up. Examples of this include GDV, colic, GI obstruction, and pneumothorax. High concentrations of N2O will continue to diffuse into those confined areas faster than N2 can diffuse out. Because there is no significant amount of N2O in the body before delivering the N2O, it will dissipate into the closed structures and could potentially double in that space, causing a great deal of pressure on the structures of the organ or the surrounding tissues (compromising respiration or cardiovascular circulation). The cuff on the endotracheal tube could also increase causing pressure on the tracheal wall (Eger et al. 2003; Eger 1974).
It’s important to remember that when utilizing N2O gas, the monitoring of CO2 could be inaccurate (Thurmon et al. 1996). There should also be some concern that nitrous oxide could be used for recreational abuse. Precautions should be taken to avoid misuse (NIDA 2009).
Halothane (classification: halogenated hydrocarbon)
The following are characteristics of halothane:
- Vapor pressure is high.
- It utilizes precision vaporizer.
- Solubility coefficient is low; fat solubility is high.
- There is rapid induction of anesthesia, but recovery is longer because of retention in the fat tissues.
- Approximately 20% metabolism of halothane occurs in the liver.
- Cardiovascular: The heart is sensitized to catecholamines, which can cause arrhythmias. Halothane depresses the myocardium, causing decreased cardiac output and cardiac contractility.
- It causes vasodilation and subsequent hypotension.
- There is increased vagal tone, resulting in bradycardia.
- It is not as irritating to the respiratory tract as isoflurane.
- Reproduction: Halothane crosses the blood placental barrier, and it may be harmful to the fetus in pregnant animals.
- It increases cerebral blood flow, which increases intracranial pressure. It is not for use in patients with head trauma or brain disease.
- Preservative thymol is used in halothane as an antioxidant. Thymol can cause halothane to become sticky and increases the need for vaporizers to be cleaned and calibrated.
- Halothane is no longer manufactured in the U.S.
Isoflurane (classification: halogenated ether)
The following are characteristics of isoflurane:
- As a result of isoflurane having a similar vapor pressure to halothane, halothane vaporizers can be professionally converted to isoflurane vaporizors (Fig. 15.1).
- Solubility coefficient is low in the tissue and the blood, which increases induction and recovery times.
- Isoflurane does not increase cerebral blood flow as long as ventilation is maintained (McKelvey et al. 2003).
- It causes dose-dependent vasodilation and subsequent hypotension.
- There is less cardiac depression than with halothane.
- It is absorbed and eliminated mainly by the lungs.
- Patients may hold their breath or resist during mask induction or anesthetic chamber induction due to the intense odor.
- It is irritating to the respiratory tract.
Figure 15.1. Sevoflurane and isoflurane vaporizers mounted side by side on an anesthesia machine.

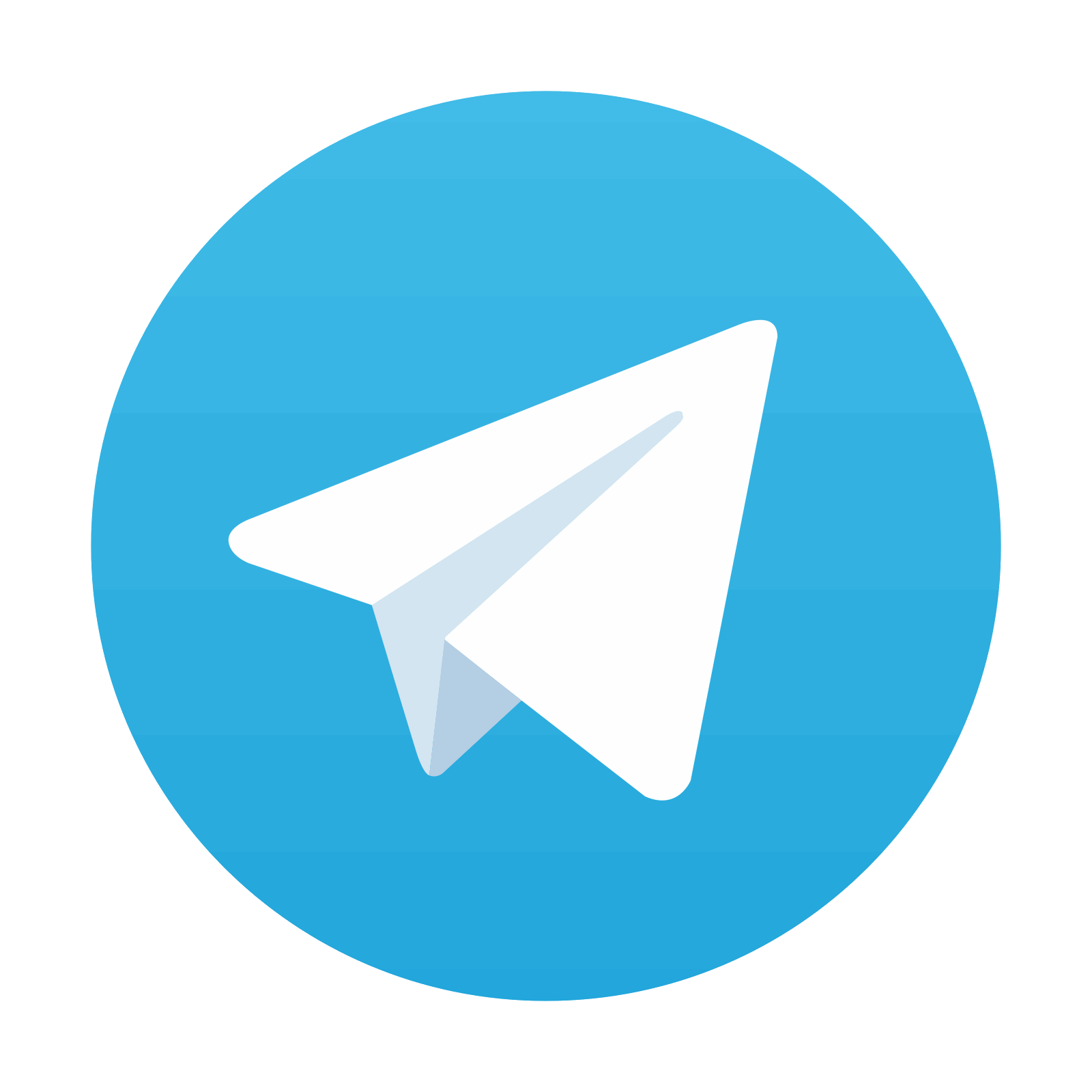
Stay updated, free articles. Join our Telegram channel

Full access? Get Clinical Tree
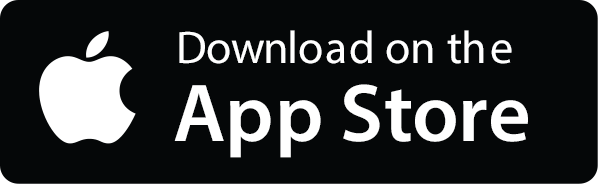
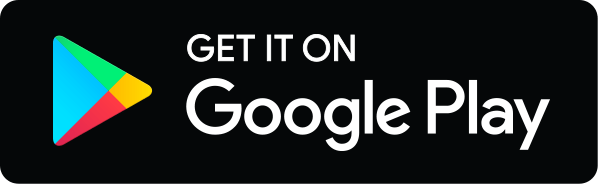