Inhalant Anesthesia and Inhalant Anesthetics
Overview
Inhalant anesthetic drugs (inhalants) act at the brain and spinal cord to produce general anesthesia. They are suitable for use in all species including reptiles, birds, fish, and zoo animals. Their safe use requires knowledge of their pharmacologic effects and physical and chemical properties. Anesthetic doses produce unconsciousness (hypnosis), immobilization (muscle relaxation, hyporeflexia), and analgesia. Inhalant anesthetics provide optimal control of anesthesia, rapid induction and recovery from anesthesia, and have minimal carryover (“hangover”) effects. Adjuncts to anesthesia (tranquilizers, analgesics) are frequently used in conjunction with inhalants to augment the quality of anesthesia and reduce the amount of inhalant anesthetic required to maintain anesthesia, thereby decreasing cardiorespiratory depression.
General Considerations
I Inhalant anesthetics are vapors or gases that are inhaled directly into the respiratory system
II To produce anesthesia, inhalant anesthetics must be absorbed from the alveoli into the bloodstream and carried by the blood to the central nervous system (CNS)
III Inhalant anesthetics are primarily eliminated by the lungs
IV The uptake and elimination of inhalant anesthetic drugs are relatively rapid, thus the depth of anesthesia can be controlled effectively, but constant monitoring is required
V The classical stages and planes of anesthesia (Fig. 9-1) were developed by Guedel for ether anesthesia and continue to be loosely applied to most inhalant anesthetics
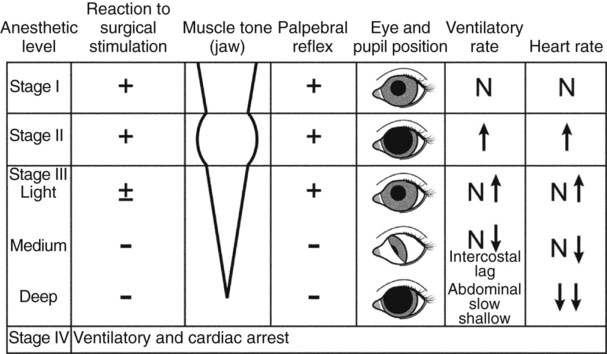
A Stage I: analgesia (from the beginning of induction to the loss of consciousness)
1. Disorientation, with normal reflexes or hyperreflexia, is the most common feature
2. Anxiety, increased heart rate, and rapid respirations may occur
B Stage II: delirium or excitement, which represents the period of early loss of consciousness
1. Potential hazards of stage II include struggling, physical injury, and increased sympathetic tone
2. Voluntary centers in the brain become depressed; the patient becomes unaware of its surroundings
3. During light anesthesia, the animal may react to stimuli (e.g., sound) with exaggerated struggling
4. Respirations are generally irregular in depth and rate, and breath holding may occur
5. The eyelids are widely open, and the pupils are dilated because of sympathetic stimulation
6. Reflex vomiting may occur unless food has been withheld; defecation and urination may occur
7. The duration of stage II can be decreased or eliminated by preanesthetic medication
1. Plane I: marked by more regular respiration
a. Carbon dioxide (CO2) retention during the preceding stages may double tidal volume for the first few minutes
b. Preanesthetic medication directly affects the rate and volume of respiration
c. Responses to pain, although depressed, are still present
2. Plane II: respiratory rate may be increased or decreased and respiratory volume (tidal volume) is decreased; cardiovascular function is mildly depressed
3. Plane III: loss of intercostal muscle activity
4. Plane IV: complete paralysis of muscles needed for breathing
D Stage IV: respiratory arrest followed by circulatory collapse; death ensues within 1 to 5 minutes
Desirable Properties of Inhalant Anesthetic Drugs
Factors Controlling the Brain Tension of Inhalant Anesthetic Drugs
I Physical and chemical properties of the drug (See Table 9-1)
TABLE 9-1
Boiling Points, Vapor Pressures, and Vaporization of Inhalant Anesthetic Drugs
Drug | Boiling Point (°C) | Vapor pressure at 20° C (mm Hg) | Maximum Concentration of Vapor (%) Delivered by Saturation Vaporizer at 20° C | Useful Ranges of Concentration (agent used alone) | |
Induction (%) | Maintenance (%) | ||||
VOLATILE ANESTHETIC DRUGS | |||||
Desflurane | 23.5 | 664 | 87.4 | 8-15 | 5-9 |
Sevoflurane | 59 | 160 | 22 | 4-5 | 2.0-3.5 |
Isoflurane | 48 | 252 | 33 | 2-6 | 1-3 |
Halothane | 50 | 243 | 32 | 1-4 | 0.5-2.0 |
ANESTHETIC GAS | |||||
Nitrous oxide* | -89 | 39,500 (50 atm) |
A Vapor pressure of the inhalant determines its volatility
1. Volatility determines the design of the vaporizer used to deliver clinically relevant concentrations of inhalants (see Chapter 12)
B Boiling points (other than for nitrous oxide [N2O] and desflurane) are higher than room temperature (70° F or 27° C)
A The concentration of inhalant delivered to the animal is determined by the type of vaporizer, the vaporizer setting, the fresh gas flow rate, and the type of anesthetic circuit (Chapter 12)
B Frequent inspection and maintenance of inhalant anesthetic equipment is necessary to prevent malfunctions such as leaks and sticky one-way valves
III Ventilation and uptake: The partial pressure of inhalant anesthetic in the brain and spinal cord depends on the alveolar partial pressure; the alveolar partial pressure of an inhalant anesthetic is the result of the inspired concentration, the alveolar ventilation, and uptake from the lungs. Rapidity of uptake of inhalants is determined by their blood-gas partition coefficients, the cardiac output or lung blood flow, and the alveolar-to-venous partial pressure difference.
1. Concentration effect: the greater the inspired concentration (vaporizer setting), the more rapid the rate of rise of the alveolar concentration
1. Generally, the greater the ventilation (minute volume), the more rapid the approach of the alveolar gas concentration of any inhalant to its inspired gas concentration, but excessive hyperventilation may slow induction by reducing cerebral blood flow
2. The rate of rise of the inhalant concentration in the alveoli may be delayed in the presence of large lung volumes because of the increased functional residual capacity (volume of gas in the lungs at the end of expiration)
3. Factors affecting ventilation
c. Increased dead space (anatomic and physiologic) that develops during anesthesia results in decreased effective alveolar ventilation; tidal volume (VT) = dead space volume (VD) + alveolar ventilation (VA): VT = VD + VA
d. Effective alveolar ventilation requires a patent airway
e. Preanesthetic and induction drugs may cause respiratory depression, slowing the onset of action of inhalant anesthesia
C Uptake of inhalant anesthetic from the lungs
1. Solubility: describes how much of a substance can be dissolved in a gas, liquid, or solid (e.g., fat); the relative solubility of inhalants is usually expressed as a partition coefficient; how an inhalant is distributed between two phases (e.g., between blood and gas, between tissue and blood); Ostwald’s partition coefficient expresses the solubility of an inhalant between blood and gas. An inhalant with a blood-gas partition coefficient of 2 has one volume in the alveoli per two volumes in the blood at equilibrium.
Agent | Blood-Gas Partition Coefficient (Ostwald’s coefficient) |
Halothane | 2.36 |
Isoflurane | 1.41 |
Sevoflurane | 0.69 |
Nitrous oxide | 0.49 |
Desflurane | 0.42 |
a. An inhalant’s blood-gas partition coefficient and potency determine its rapidity of onset of effect
b. The greater the blood-gas partition coefficient, the greater the solubility of the inhalant in blood; the tension of the inhalant in arterial blood rises slowly for drugs that are highly soluble in blood (high blood-gas coefficient); onset of the clinical effect is contingent on the tension of the inhalant developed in the blood
c. Inhalants that are more soluble in blood have longer induction and recovery times because large amounts of inhalant must be taken into the blood before their tension (partial pressure) rises sufficiently to produce anesthesia; clinically, slow induction may be overcome by raising the inspired concentration of the inhalant anesthetic to values above those necessary to maintain anesthesia (anesthesia overpressure)
d. The lower the blood-gas partition coefficient, the less soluble the inhalant is in blood (only small quantities are carried in the blood); both alveolar concentration and tension will rise rapidly and blood inhalant anesthetic tension (partial pressure) increases rapidly (Fig. 9-2); less soluble inhalants: N2O, isoflurane, and sevoflurane have comparatively short induction and short recovery times
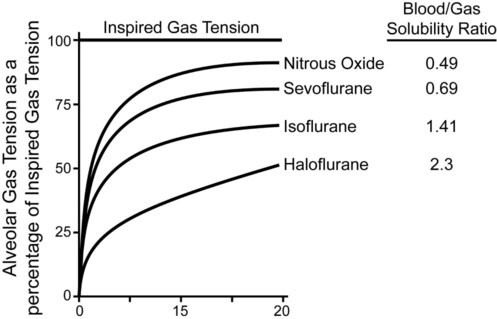
2. Cardiac output: blood carries the inhalant anesthetic away from the lungs; the greater the cardiac output, the slower the rate of rise of alveolar concentration and the lower the partial pressure of the inhalant anesthetic in the lung and blood
a. Excited, stressed animals generally have an elevated cardiac output and a slower rate of induction because of the slower rate of rise of alveolar concentration and lower partial pressure of the inhalant anesthetic in the lungs; animals with a decreased cardiac output are induced to anesthesia very rapidly
3. Alveolar-venous anesthetic tension difference: during induction, tissues remove nearly all the inhalant brought to them because of the high tissue solubility of most inhalant anesthetics; venous blood returning to the lungs contains little inhalant; as time passes, increasing tissue saturation raises the venous blood concentration; therefore, less inhalant is taken up in the lungs; inhalant uptake is a continuous process towards a steady state (plateau) concentration
a. Right-to-left intracardiac or intrapulmonary shunts (e.g., Fallot’s tetralogy) delay induction
b. Left-to-right shunts can speed the rate of induction by increasing the rate of rise of the alveolar concentration and the partial pressure of the inhalant anesthetic in the lung; this occurs very rapidly if the cardiac output is low
c. Pathologic changes in alveoli: if the alveolar membranes are affected by disease resulting in exudate, transudate, emphysema, or pulmonary fibrosis, diffusion may be impaired, and the uptake of the inhalant slowed
IV Factors governing brain and tissue uptake of inhalants
A The factors are the same as those determining uptake from the lungs
B The uptake by tissue is dependent on anesthetic tension in the blood, blood flow, and tissue capillary density
1. Tissues can be categorized into four groups according to blood supply (Fig. 9-3)
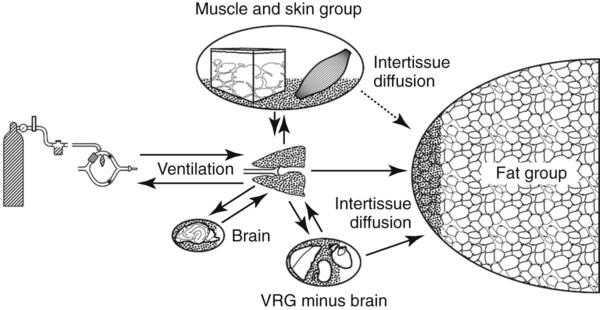
a. Vessel-rich group (VRG): 75% of cardiac output (e.g., brain, heart, intestines, liver, kidneys, spleen)
b. Vessel-moderate group, or muscle group (MG): 15% to 20% of cardiac output (e.g., muscle, skin)
c. Fat group (FG): 5% of cardiac output (e.g., adipose tissue)
d. Vessel-poor group: 1% to 2% of cardiac output (e.g., bone, tendons, cartilage)
Group | Examples | Principal points |
VRG: vessel-rich group | Brain, heart, liver | Equilibration of an inhalant anesthetic drug is complete in 5-20 minutes |
MG: vessel-moderate group/muscle group | Muscle, skin | Equilibration of an inhalant anesthetic drug is complete in 1.5-4 hours |
FG: neutral fat group | Adipose tissue | May affect recovery time after prolonged anesthesia |
C Tissue-blood partition coefficients vary less than blood-gas coefficients (except for fat)
1. Equilibration of an inhalant in the VRG is generally completed in 5 to 20 minutes; this is approximately how long it will take for anesthesia to become deep enough to do surgery, providing the anesthetic concentration (vaporizer setting) is appropriate
2. Equilibration in the MG may take 1.5 to 4 hours
3. Because of the higher blood flow, arterial-tissue partial pressure differences (and therefore uptake) decreases far more rapidly in VRG than in MG
4. The FG occupies 10% to 30% body mass but receives only about 5% of the cardiac output
a. FG tissue has a higher tissue solubility for inhalants than most other tissues and thus has a greater and more prolonged capacity to absorb them
b. FG has little effect on induction of anesthesia because of its low blood flow
c. FG may affect recovery time after prolonged anesthesia (3 hours or longer) because of the prolonged time it takes for the anesthetic to be eliminated from fat
Inhalant | Fat-Blood Partition Coefficient |
Nitrous oxide | 2.3 |
Desflurane | 27.2 |
Isoflurane | 48 |
Halothane | 65 |
Sevoflurane | 65 |
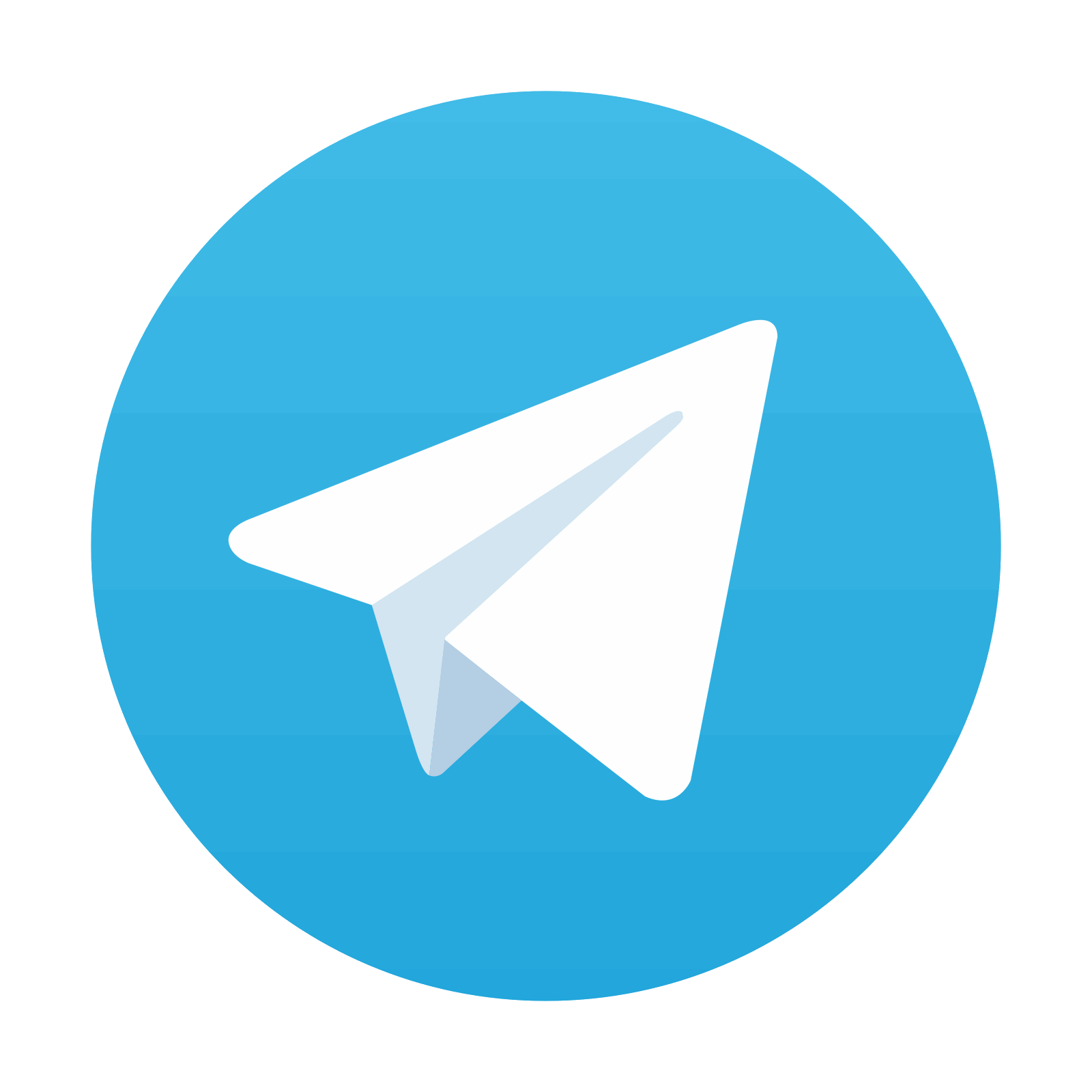
Stay updated, free articles. Join our Telegram channel

Full access? Get Clinical Tree
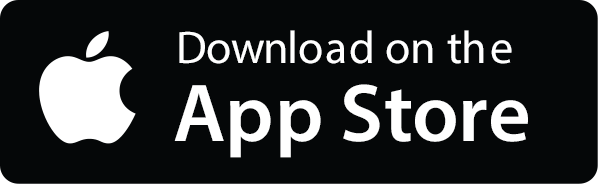
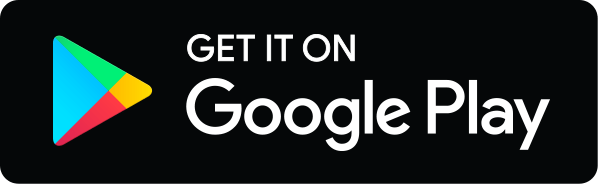