, Monika Hassel2 and Maura Grealy3
(1)
Centre of Organismal Studies, University of Heidelberg, Heidelberg, Germany
(2)
Spezielle Zoologie, Universität Marburg FB Biologie, Marburg, Germany
(3)
Pharmacology and Therapeutics, National University of Ireland Galway, Galway, Ireland
Beginning new life must be prepared in the parents. The sex organs must be fully developed and grown, and the gametes maturated. In humans the development of the sex organs commences in the embryo but the organs acquire full maturity as late as puberty. As an introduction we first consider the meaning of sexual reproduction in general.
7.1 The Essence of Sexuality
7.1.1 Sex Is Devoted to the Exchange and Recombination of Genetic Information in Offspring; As a Rule Mother and Father Contribute Genes, the Selection of Which Is Subject to Chance
Sex in the sense of genetics primarily is a process by which new combinations of alleles are assembled and assigned to descendants. Most eukaryotic organisms exist in two complementary forms, females and males, who invest genetic material in a mutual game of chance. Unlike asexual reproduction that is exclusively based on mitotic division, sexual reproduction incorporates two characteristic events enabling novel genetic combinations: (1) fusion of two genetically different cells (gametes) in fertilization, and (2) meiosis, a process that usually takes place in preparation for fertilization (in several “lower” eukaryotes meiosis occurs at some other point of the life cycle) and enables the rearrangement of individual chromosomes and genes.
Most multicellular animals are diploid (exception: bee drone). The nuclei of their cells harbour two sets of chromosomes. Each distinct chromosome is represented twice, known as a pair of homologous chromosomes, the one homologue being designated maternal, the other paternal, with respect to their provenance in the previous act of fertilization. As a rule, one female and one male each transmit one set of chromosomes, that is one haploid genome, with their gametes (germ cells, sex cells) to the next generation. Each sexual partner may only pass one homologous chromosome of each pair to the future descendant and it is subject to chance whether the originally maternal or the paternal chromosome is allocated to a distinct germ cell.
We repeat what had been said in Chap. 1. In the new gametes, any particular chromosome can come from either parent; for instance, chromosome No. 1, in the newly formed egg cell may be the originally paternal chromosome, once carried by the sperm, another chromosome, let’s say chromosome No. 2, was also of paternal origin, while chromosome No. 3 was once received from the mother of the individual who produces the new gametes. Likewise, although in the testes of the sexually mature man the newly produced sperm cells are all haploid, each sperm cell contains chromosomes partly derived from his mother, partly from his father, and the particular mixture is subject to chance.
Considering all 23 chromosomes a haploid germ cell embodies, the number of possible new combinations is huge. There are 2 23 , or 8,388,608 different possible combinations. This means, a woman could produce approximately 8.4 million egg cells each of which differs from all the others in at least one chromosome. Likewise, a man could produce 8.4 million different sperms.
Additionally, chance is involved when homologous chromosomes exchange genes by cross-over since there are no strictly fixed positions along the chromosomes where crossover occurs. Furthermore, chance is at play when two germ cells fuse in fertilization, since it is not predetermined which gametes will meet each other.
The random rearrangement of genetic material is associated with production of offspring and, therefore, is called sexual reproduction. The offspring literally embody the new combination of genes and subject it to the test of natural selection.
Gametes occur as two types of highly specialized cells, the egg and the sperm. In a number of plants and “lower” animals, each individual produces both types of gametes. This condition is called hermaphroditism, combining the mythological figures Hermes and Aphrodite; it is exhibited by monoecious species (Greek: mono = one, oikos = house). In the vast majority of animals, however, the role of producing eggs and sperm is allotted to two distinct types of individuals, and the species are dioecious (Greek: di = two, twofold, oikos = house) or heterosexual, with females and males.
As a rule, the differences between the sexes are not restricted to the reproductive organs but extend to other traits such as size, ornaments and weaponry, and always include sex-specific, reproduction-associated behaviour. Thus, the typical animal species displays sexual dimorphism.
7.1.2 Most Organisms Have a Bisexual Potential
Two understand aberrant but also normal sexual development in humans, it is important to appreciate that each individual is basically endowed with bisexual potential and possesses almost all of the genes required to develop both female and male characteristics. Bisexual potential is testified in hermaphroditism but exists in heterosexual organisms including humans as well. Otherwise, a mother could not transmit “male” genes from her father to her sons, and a father could not transmit “female” genes from his mother to his daughters.
7.2 Sex-Determining Systems and Genes
Given the fact of bisexual potential, what is the ultimate decisive event? In all organisms, beyond the sets of genes required to develop both types of gonads and sex organs, additional key genes, (selector genes, master genes) determine which of the two sets of genes is actually being used in developing an individual’s sex. Typically, one decisive key gene is present but allotted to only half of the offspring. This is referred to as
Genotypic sex determination. Alternatively, master genes are differentially activated by environmental influences. Such cases are subsumed under the heading.
Environmental or phenotypic sex determination.
7.2.1 In Phenotypic Sex Determination: Environmental Variables Take the Decision
In environmental sex determination the ultimate decision is made only after fertilization, and external cues such as temperature or presence of a sexual partner make the choice, presumably by influencing the activity of master genes. For instance, in the polychaete annelid Ophryotrocha puerilis two individuals that encounter each other by chance influence each other by means of chemical signals (pheromones) and eventually one individual plays the role of the male and one the role of the female. Environmental sex determination has been discovered in several invertebrate species but also is known to occur in fish and reptiles (all crocodilians, many turtles and some lizards). Environmental sex determination is indicated, but not proven, by sex ratios deviating significantly from 1:1.
The Mississippi-alligator make micro-organisms to produce breeding heath. The female animal establishes a compost heap to nourish the micro-organisms. At 30 °C all the eggs give rise to females, at 34 °C all give rise to males. At temperatures between 32 and 33 °C females and males arise in varying ratios. How an egg-laying crocodile manages to arrange the clutch of eggs in the pile in such a way that offspring hatch in a balanced ratio of female and male individuals, is not known. Biologists interested in evolutionary theories ask: is a ratio of 50:50 always appropriate? It is supposed, and supported by findings in the Australian lizard Amphibolurus muricatus, that fitness of a population might increase if sometimes more, sometimes less females make use of limited nutritive resources for growing large eggs. Perhaps breeding temperature serves as an environmental counterbalance. Zoologists with sense of humour like to refer to further examples of phenotypic sex determination. Adult, full grown anglerfish of the deep sea (genus Edryolichnus) are always females. To get an abiding partner without fail they catch a larva of their own species. The larva attaches onto the body of the female and both specimens grow together. The larva becomes a mini male which supplies sperm at call. There is no need for the male to look after food himself; on the other hand he is captured life long. If the larva escapes this fate it will become female and an angler. A similar destiny is granted the larvae of the annelid Bonellia viridis. The dwarf male lives in the nephridia of the green female. A change of sex, as it occurs in Ophryotrocha, is also known from the slipper snail Crepidula. Several animals form a pyramid, each younger one sitting on top of the older animals. The animal at the bottom is the oldest and largest one and female, the animal on the top is the youngest and male. In between one finds (infertile) intersexual specimens. Sex determination is thought to occur via pheromones.
7.2.2 In Genotypic Sex Determination the Decision Is Left to Chance in Fertilization; Chance Allocates a “Sex-Chromosome” Bearing Key Genes to Only Half of the Offspring
Traditional belief, offered by ancient writers such as Aristotle, ascribes sex determination even in humans to non-genetic determinants such as nutrition or the heat of passion during intercourse. How such factors ensure a sex ratio of 1:1 is usually not the subject of much attention in superstitious explanations.
But how can chance yield a ratio consistently close to 1:1 (in humans, 105 male births per 100 female births)?
In genotypic sex determination certain particular key genes (selector genes, master genes) bring about the decision whether the set of genes required for male, or the set of genes required for female development will be expressed. Such a key gene is, as a rule, found on one chromosome of a pair of homologous chromosomes while its partner lacks this gene.
Such a pair of unequal chromosomes is called
Heterosomes, also called allosomes or gonosomes, while all the other chromosomes of the species are collectively called
Autosomes; from which sex-specific differences are not known.
In haploid germ cells only one heterosome of the pair can be present, it is either the chromosome containing the key gene or the chromosome lacking this gene. As a consequence, in conception only one half of the descendants will get this key gene.
The principle may be explained more specifically using humans as the example. All humans carry 2 × 23 = 46 chromosomes in their diploid cells. Of these, 2 × 22 chromosomes are indistinguishable in males and females and, therefore, categorized as autosomes. In females, the 44 autosomes are complemented by a two X. In men, besides 44 autosomes two morphologically distinct chromosomes are found: one is an X, like those found in women, while the other is a modified X, termed the Y chromosome.
When meiosis takes place, of the complete diploid set of 44 autosomes +2 heterosomes one haploid set of 22 autosomes + 1 heterosome is parcelled out to each future egg or sperm cell. In the oocytes this set of 22 autosomes is always supplemented by one X. Spermatocytes also get a total of 23 chromosomes, but the set includes, besides 22 autosomes, either the X or the Y. On the average, 50 % of the sperm cells obtain the X chromosome by chance while the other 50 % obtain the Y chromosome. As a consequence, while all diploid offspring will have 44 autosomes, with respect to the heterosomes, 50 % of the diploid offspring will be marked by XX, the other 50 % by XY. It then will turn out that XY babies will become boys, while XX babies will become girls. With respect to the two heterosomes, in humans and other mammals, females are said to be homogametic (XX) while males are heterogametic (XY). The suffix ‘gametic’ suggests consequences in gametogenesis. With respect to decisive key genes one speaks of homozygous or hemizygous constitution.
X and Y chromosomes are often called “sex chromosomes” but this designation is highly misleading. Actually in mammals the majority of genes required for the development of sexual traits are located on the 44 autosomes. A better, but still misleading designation for the mammalian heterosomes is “sex-determining chromosomes”. As discussed in Sect. 7.3.3, it is not the entire X or Y chromosome that is of significance in sex determination but a dominant male-determining key gene Sry, located on the Y. Female development is the default program switched on by female-specific key genes such as R-spondin1 when the male-determining gene is absent or defective.
Unexpectedly, intensive research into the nature of genotypic sex determination has shown that the role of chance in distributing chromosomes or genes is the only common denominator among the various types of genotypic sex determination identified in the animal kingdom. Despite nature’s tendency to retain established “solutions” to biological problems, and despite the universality of sexual dichotomy, there is no universal, molecular mechanism of genotypic sex determination. In most animal species the mechanism is complex and includes cascades of mutually dependent events. Frequently, the sex-determining genes occur on two morphologically different chromosomes, usually called, as in mammals, the X and Y, or they are designated W and Z indicating that these two systems are different in basic features. In both cases one of the two sexes is homogametic and the other heterogametic – but in the XY and the WZ system the correlation is inverse. Despite the consistent XY or WZ formalism, the actual roles of these chromosomes vary in different organisms, and they bear different genes. By definition in systems designated XY the males are heterogametic, in systems designated WZ males are homogametic. A chromosome designated Y in mammals and a chromosome designated Y in Drosophila bear the same name signature but different genes; they merely obey a superficially similar formalism in sex-determination in that males are heterogametic. In birds, incidentally, females are (usually) heterogametic and the males homogametic. While in mammals the male-determining key gene Sry is located on the Y and males are XY, in birds the male-determining key gene (presumably) DMRT1 is located on the Z and therefore males are ZZ. Teleost fishes display an amazing diversity of genetic as well as environmental sex-determining systems, and combinations of such systems. Information about the occurrence of heterosomes is given in Table 7.1.
Table 7.1
Heterosomal Constitution
Animal group | The homo- gametic sex | becomes | The hetero-gametic sex | becomes |
---|---|---|---|---|
Mammals, humans | XX | ♀ | XY | ♂ by Y-Sry |
Birds | ZZ | ♂ by Z-DMRT1 | WZ | ♀ |
Fish Xiphophorus variatus | XX or ZZ YY | ♂ ♂ | XY or WZ XY | ♀ ♀ |
Drosophila | XX | ♀ | X (Y insignificant) | ♂ |
Butterflies | ZZ | ♂ | WZ | ♀ |
To exemplify variability only two model organisms will be presented:
In the nematode Caenorhabditis elegans
XX individuals are hermaphrodites; pure female individuals are not known.
X0 individuals are male (arisen by loss of one X in gametogenesis).
In Drosophila, the ratios between the number of X chromosomes and the two sets of autosomes (AA) in each individual diploid cell is decisive in determining the sex of each individual cell.
XX/AA (a ratio of 1:1) determines female
XY/AA (a ratio of 1:2) determines male
X0/AA (a ratio of 1:2) determines male, but the males are infertile.
The Y chromosome in Drosophila is of significance in spermatogenesis in the adult male but not in early sex development. Sexual determination is cell autonomous and not mediated by hormones. If one X is lost due to a faulty mitosis (nondisjunction) in an XX embryo, the resulting X0 cell and all its descendants become male in female surroundings (while the descendants of the corresponding XXX cells are “superfemale”). In Drosophila and other insects, individuals composed of a mosaic of female and male cells are called gynanders (Greek: “wifeman”) or gynandromorphs (Fig. 7.1). In insects unlike vertebrates, there are no sex hormones that could moderate differences in X chromosome numbers and ensure a uniform phenotype. An individual with an abnormal ratio of XX/AAA develops an intersexual phenotype with genetically uniform cells but an intersexual organismal appearance. In each somatic cell X-linked genes code for factors aptly called numerators, while genes located in the autosomes code for denominators. In the presence of two X chromosomes more numerator proteins are produced (by genes that apparently escape dosage compensation, or before dosage compensation is accomplished). When numerator proteins predominate, the master gene sxl (sex lethal) is switched on. The SEX LETHAL protein SXL directs a cascade of biochemical events. These include the correct splicing of its own pre-mRNA by which SXL makes it possible to maintain its own synthesis. The cascade of events set going by SEX LETHAL terminates in the activation of female-specific genes. In contrast, male development is the default program, resulting from insufficient levels of SEX LETHAL protein to trigger female development.
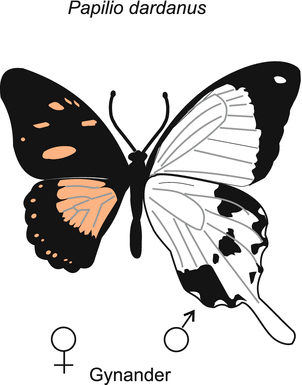
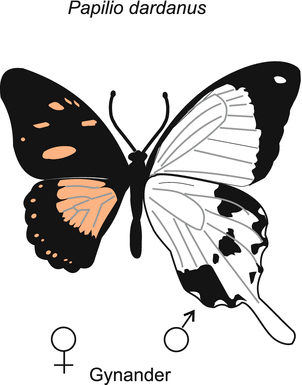
Fig. 7.1
Gynander in a sexually dimorphic butterfly
7.3 Early Sexual Development of Mammals and Humans
7.3.1 Primarily the Gonads are Sexually Indistinct; They Develop in Cooperation with the Embryonic Kidneys Resulting in the Urogenital System
In mammalian development the gonads and embryonic kidneys develop in close proximity and partially from common primordia; the primordial kidneys contribute their ducts thus giving rise to the common urogenital system (Fig. 7.2). The primordial material of the system derives from the intermediate mesoderm (segment stalk) between the somites and the lateral plates (see Fig. 5.10). Both the gonads and the embryonic kidneys arise from the tube of the holonephros with its ciliated funnel, and the adjoining nephrogenic mesenchyme plus the gonadal primordium. From the holonephros three parts segregate in temporal order:
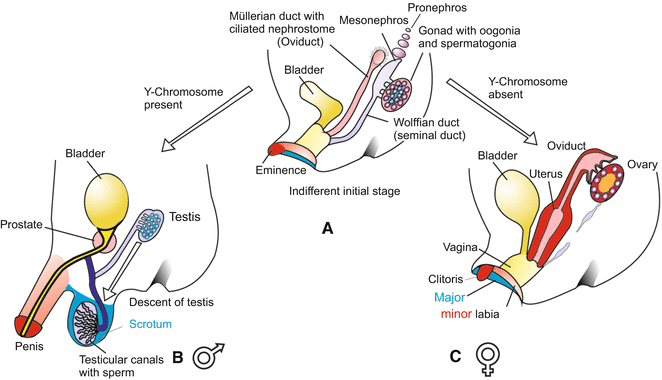
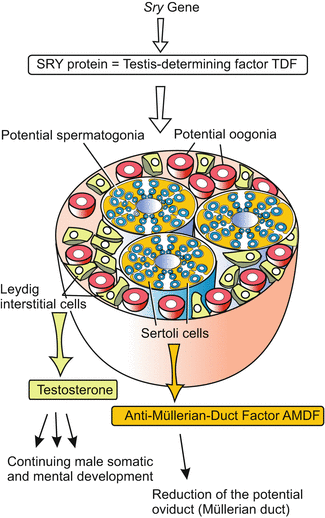
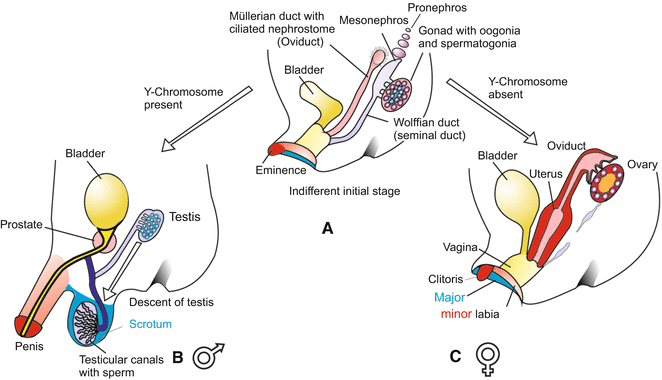
Fig. 7.2
(a–c) Sexual development in humans. (a) Divergent development starts from a common, indistinct stage (middle). (b) Development proceeding in male direction. In the male gonad, oocytes in the cortex begin to degenerate while the testis cords in the centre of the gonad continue to develop into seminiferous tubules. Under the influence of the testosterone-induced AMDF (Anti-Müllerian Duct Factor) the Müllerian duct regresses, the Wolffian duct comes to be the vas deferens. The gonad is converted into a testis. The former mesonephros is transformed into the epididymis. (c) In the female gonad, the central testis cords disappear, while the oocytes in the peripheral cortex survive and become surrounded by follicle cells; the gonad is developing into an ovary. The Müllerian duct is enlarged to become the oviduct and uterus, while the Wolffian tube is reduced. The external genitalia also arise from a common, indistinct stage
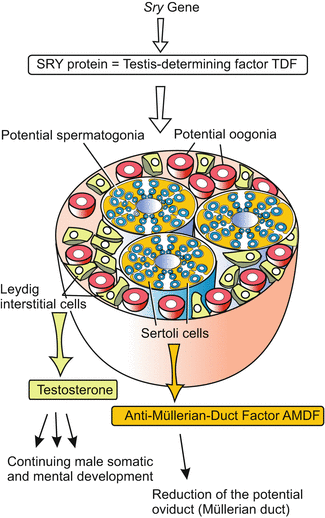
Fig. 7.3
Sexually indistinct gonad and effect of the SRY factor = TDF (Testis-Determining Factor) encoded by the Sry gene on the gonad. The Sertoli cells are induced to emit the hormonal factor AMDF, the Leydig interstitial cells are stimulated to produce and release the male sexual hormone testosterone
1.
The pronephros which uses the holonephric duct with its ciliated funnel, now called Müller’s duct; in females this duct is the precursor of the oviduct.
2.
The mesonephros which contributes the Wolffian duct, in males this duct is the precursor of the spermatic duct (vas deferens).
3.
The metanephros, the final kidney in both sexes which develops its own duct, the final urinary duct called the ureter (see Fig. 7.4).
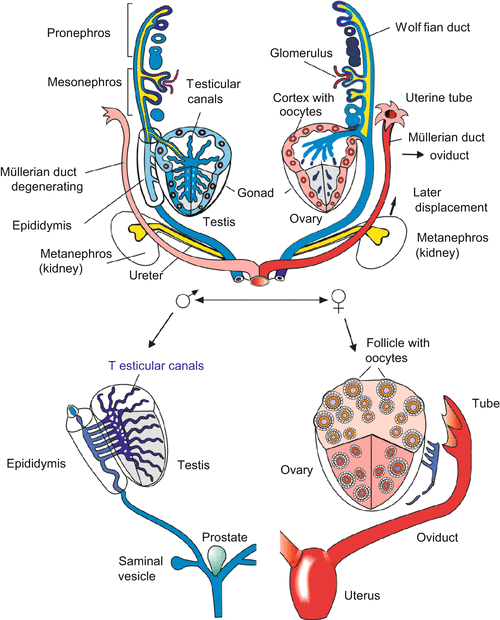
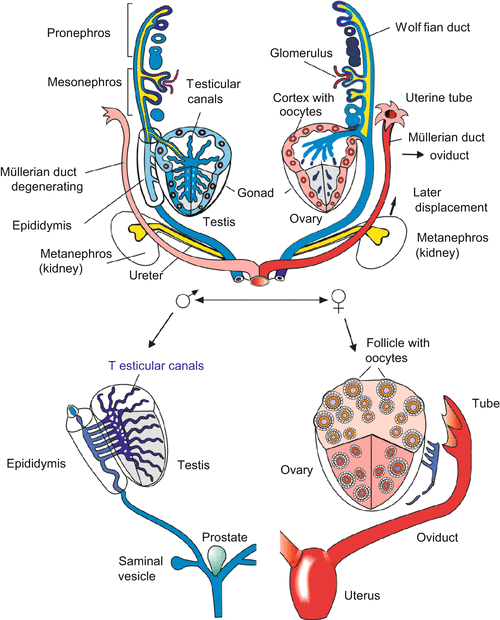
Fig. 7.4
Progressing development of the sexual organs starting from an indistinct common condition. From the paired organs only the left or the right organ is shown
It must be pointed out, that this sequence of events starts in female and male individuals from the same primordia including the precursors of the oviduct and the spermatic duct.
7.3.2 Sexual Development in Mammals Proceeds in Steps From an Indistinct Initial State Over Divergent Paths to Sex-Specific Behaviour; a Brief Introductory Overview
The basic bisexual potential that our genetic heritage harbours is morphologically expressed. Initially our somatic traits, the gonads included, are the same in both sexes. In the gonads this indistinguishable state is documented by the initial presence of both oogonia and spermatogonia. Also the primordia of the genitalia and other somatic characteristics that serve sexual propagation are prepared for both genders and initially indistinguishable (Figs. 7.2, 7.3, 7.4, and 7.5). Beginning with the activity of a male- or female-determining key gene in the gonads the further development is directed in alternative paths and eventually end up in the known male or female traits.
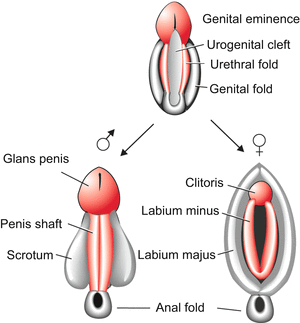
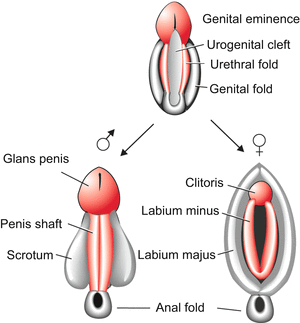
Fig. 7.5
Development of the external genitalia starting from an indistinct common condition
In mammals, particularly as studied extensively in mice and humans, sexual development is a multistep process. Several stages occur prior to birth
1.
the genetic sex,
2.
the gonadal sex,
3.
the somatic sex,
4.
the psychological sex.
Gonadal, somatic and psychological sex of humans develop in two distinct phases of life,
(a)
an embryonic phase in which the primary sexual characteristics find their expression, and
(b)
maturation in puberty, when the testes start producing sperm, ovaries bring eggs to maturity, and the secondary sexual characteristics appear.
7.3.3 The Genetic Sex: A Single, Dominating Gene Determines Whether One Becomes a Man Or a Woman
As all eggs have an X chromosome, the genetic basis of sex depends on the genotype of the sperm cell. If the sperm contributes another X, the embryo will be XX and eventually become female. If the sperm cell contributes a Y, the embryo gets the constitution XY and will become male. The decisive significance of the Y chromosome arises from the fact that it carries a master gene Sry ( Sex-determining region of the Y chromosome ).
Sry activity generates the transcription factor SRY, also known as TDF (Testis-Determining Factor). TDF contains a DNA-binding domain of the HMG (High Mobility Group) class that enables the factor to direct the activity of subordinate genes (most of which remain unidentified). A 14 kb DNA fragment containing the Sry sequence and no other gene, was sufficient to cause XX mouse embryos to develop male traits. It appears that a single gene ultimately determines whether an individual will be a man or a woman. This does not mean that other genes are not involved in the realization of the sex; but one particular gene’s activity tips the scale. However, with a Sry gene alone, male mice are infertile. One additional Y-resident gene, called the spermatogonial proliferation factor Eif2s3y suffices to provide the animals with the capabability to produce sperm.
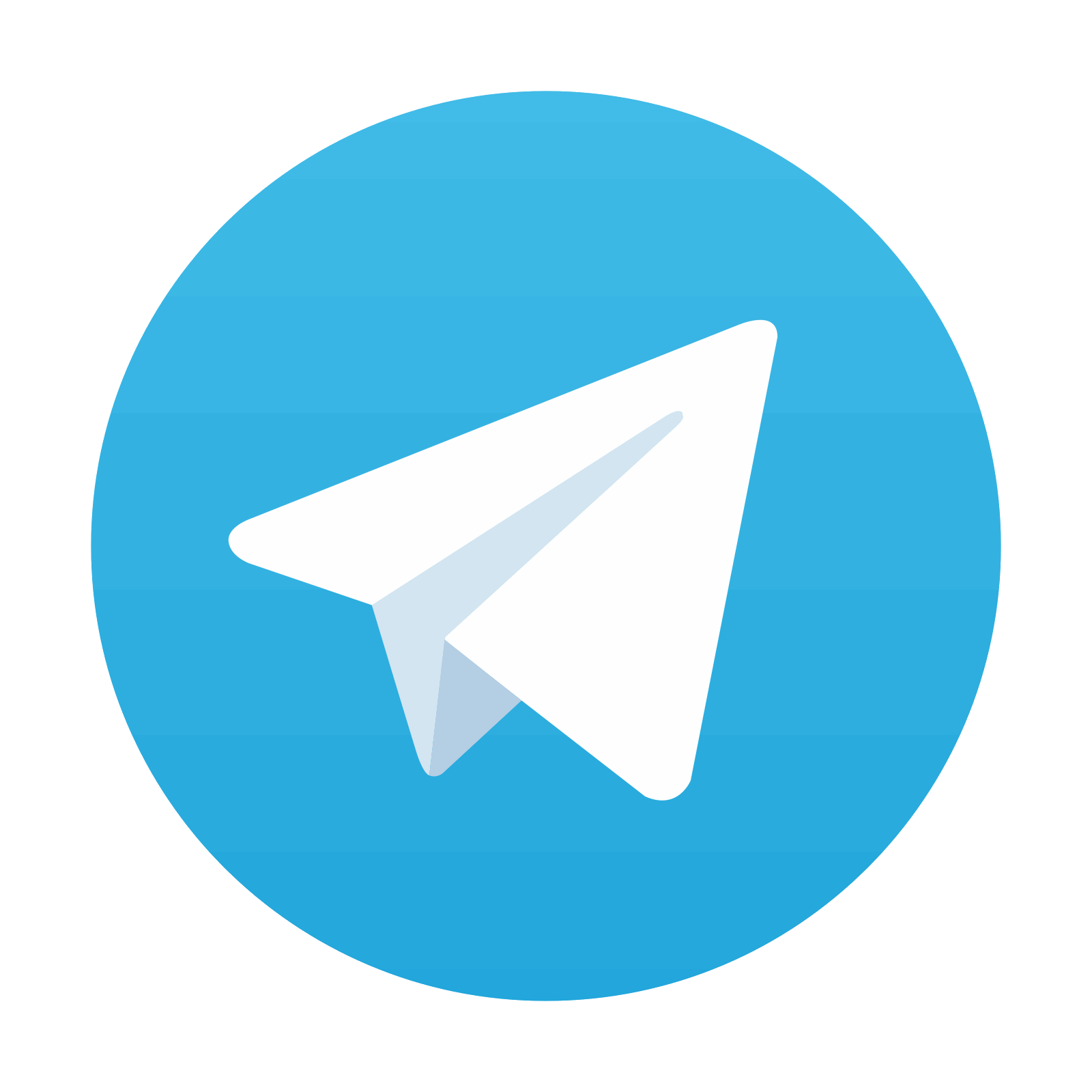
Stay updated, free articles. Join our Telegram channel

Full access? Get Clinical Tree
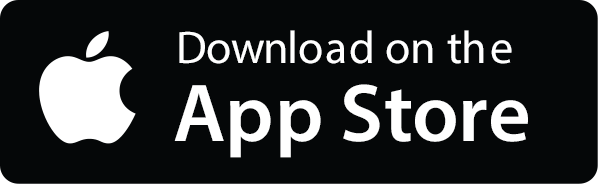
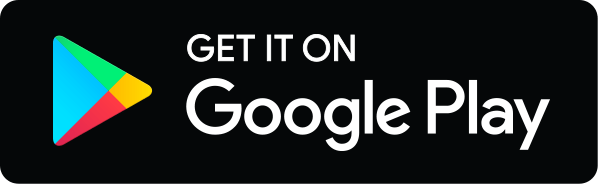