Chapter 3 Hematopoiesis
Overview
Sites of Blood Cell Production
In mammals, primitive hematopoiesis begins outside the body of the embryo in the yolk sac and shortly thereafter within the aorta-gonad-mesonephros (AGM) region of the embryo.42,130,139 Small clusters of hematopoietic stem cells (HSCs) have been identified attached to the endothelium of the yolk sac and the dorsal aorta. These HSCs and the associated endothelial cells are produced by common embryonic stem cells known as hemangioblasts.34,74,150 In addition to HSCs, committed erythroid and megakaryocytic progenitor cells, primitive erythrocytes (large nucleated cells containing embryonal hemoglobin), large primitive reticulated platelets, and rare primitive macrophages are also produced in the yolk sacs of rodents and humans.98,136 Notably, these primitive macrophages appear to develop directly from progenitor cells in the yolk sac without passing through a monocyte stage.14 Definitive erythropoiesis, prominent megakaryocytopoiesis, and limited leukocyte production also occur in the yolk sacs of cats, with hematopoiesis persisting longer during gestation than it does in rodents and humans.134 The AGM region transiently supports the development of HSCs and some committed hematopoietic progenitor cells (HPCs), but recognizable blood cells are not produced in the AGM.93
Sites of blood cell production shift during embryonic and fetal development as optimal microenvironments are produced in various tissues (Fig. 3-1).102 The liver and, to a lesser extent, the spleen become the major hematopoietic organs by midgestation in the fetus.129,134 Current evidence suggests that the AGM is more important than the yolk sack in providing HSCs to seed the liver and spleen, but the relative importance of each area in embryonic and fetal hematopoiesis remains to be clarified.102 Blood cell production begins in bone marrow and lymphoid organs during midgestation in mammals, with nearly all blood cells being produced in these organs at the time of birth.134 Blood cells are produced in the bone marrow of adult birds20; the bone marrow and sometimes the spleen of adult reptiles30; the kidney, liver, spleen, and/or bone marrow of amphibians5,42; and the kidney and/or spleen of fish.42,48
Organization of Bone Marrow
Bone marrow develops in mammals during the second trimester.21 Rudimentary fetal bone is initially filled with cartilage. Chondrocytes hypertrophy and promote mineralization of the cartilage matrix in the center of the rudimentary bone. This is followed by the entry of progenitor cells, which develop into chondroclasts that partially degrade the mineralized cartilage and form bone marrow spaces colonized by incoming blood vessels.23,131 Osteoblast progenitors enter the space created, adhere to remaining cartilage, develop into mature osteoblasts, and begin the formation of bony trabeculae. Vascular sinuses and extravascular mesenchymal cells subsequently form a connective tissue meshwork within which HSCs originating from the liver (and probably the spleen) bind, proliferate, and differentiate, ultimately producing circulating blood cells.131 When these structures are fully developed, blood is supplied to the bone marrow by nutrient arteries and periosteal capillaries (Fig. 3-2).7
The stroma of the marrow is a connective tissue consisting of stromal cells (fibroblast-like cells, also called reticular cells), adipocytes, vascular elements (endothelial cells and myocytes), neural elements, and extracellular matrix (ECM), with the arrangement creating both intravascular and extravascular spaces (Figs. 3-3, 3-4).146,147 In postnatal mammals, blood cells are continuously produced within the extravascular spaces of bone marrow. Leukocytes are also produced within the extravascular spaces of bone marrow in birds, but erythrocytes and thrombocytes are produced within the vascular spaces of the avian marrow.20
This specialized arrangement of the marrow vasculature is important in the organization of intramedullary hematopoietic microenvironments, as marrow endothelial cells are actively involved in the regulation of transendothelial (not interendothelial) movement of hematopoietic cells and blood cells between the extravascular hematopoietic space and peripheral blood.92,114 Together, endothelial cells and stromal cells produce the ECM, which consists of collagen fibers, various macromolecules capable of binding cells, and basal laminae of the sinuses.100,109 The marrow stromal cells have extensively branched cytoplasmic processes and, along with the fibers that they produce, provide structural support for the marrow (Fig. 3-5).147 These stromal cells have generally been considered to be fibroblast-like, but they also display smooth muscle characteristics in culture and have been classified as myofibroblasts by some investigators.122 The particular stromal cells that support the endothelium of the venous sinuses are termed adventitial stromal cells (Fig. 3-6).147 Granulopoiesis also occurs primarily on the surface of stromal cells.125 Adipocytes develop from mesenchymal stem cells and may share common hematopoietic functions with stromal cells.47 Autonomic nerves occur in bone marrow. Their function is not clear, but direct and indirect effects of the sympathetic nervous system on hematopoietic stem cell and hematopoietic progenitor cell proliferation and motility have been described.61
In addition to hematopoietic cells and developing blood cells, a number of accessory cells involved in regulating hematopoiesis reside within the extravascular space of mammalian bone marrow. These accessory cells include macrophages, mature lymphocytes, and natural killer (NK) cells.12,31,33,85 Erythrocyte development occurs in close association with marrow macrophages.26
Hematopoietic Stem Cells and Progenitor Cells
Beginning in midgestation and continuing throughout postnatal life, mammalian blood cells are produced continuously from HSCs within the extravascular spaces of the bone marrow. HSCs are capable of proliferation; they exhibit long-term self-renewal and differentiation. HSCs replicate only once every 8 to 10 weeks.2 The term hematopoietic progenitor cell (HPC) refers to cells that form colonies in bone marrow culture like HSCs but do not have long-term self-renewal capacities. HSCs and HPCs are mononuclear cells that cannot be distinguished morphologically from lymphocytes. The presence of a transmembrane glycoprotein termed cluster of differentiation antigen 34 (CD34) has been used to identify HSCs and early HPCs, but some HSCs (possibly inactive ones) lack CD34.43 In addition, CD34 is also present on the surface of nonhematopoietic stem cells and vascular endothelial cells.72,149 CD34 is believed to play a role in cell adhesion.43
The most primitive HSC has the capacity to differentiate into HPCs of all blood cell lineages and several cell types in tissue. The frequency of HSCs in the marrow is estimated to be less than 0.01% of nucleated marrow cells in adult mice and less than 0.0001% of nucleated marrow cells in adult cats.2 HSCs produce HPCs that can give rise to one or more blood cell types. Thus, HPCs are much more numerous in marrow than are HSCs. Less than 2% of nucleated bone marrow cells in adult dogs are CD34+, but up to 18% CD34+ cells have been reported in neonatal pups.38,128
The HSC produces a common lymphoid progenitor (CLP) and a common myeloid progenitor (CMP), as shown in Figure 3-7. The CLP is believed to give rise to B lymphocytes, T lymphocytes, and NK cells.16 The CMP is believed to give rise to all nonlymphoid blood cells (see Fig. 3-7) as well as macrophages, dendritic cells, osteoclasts, and mast cells.66,89 HPCs proliferate with higher frequency than do HSCs, but the self-renewal capabilities of HPCs decrease as progressive differentiation and cell lineage restrictions occur. When measured in an in vitro cell culture assay, HPCs are referred to as colony-forming units (CFUs). HPCs that rapidly proliferate, retain their ability to migrate, and form multiple subcolonies around a larger central colony in culture are called burst-forming units (BFUs).
The CMP (also called a colony-forming unit-granulocyte-erythrocyte-monocyte-megakaryocyte [CFU-GEMM]) gives rise to the megakaryocyte-erythrocyte progenitor (MkEP) and the granulocyte-monocyte progenitor (GMP). The MkEP produces megakaryocyte progenitors (MkP) and erythrocyte progenitors (EP). The GMP produces the granulocyte progenitor (GP), the monocyte-dendritic cell progenitor (MDP), the basophil-mast cell progenitor (BMaP), and the eosinophil progenitor (EoP) in mice (see Fig. 3-7). However, in humans, the EoP may develop from the CMP, rather than the GMP.89
Mesenchymal Stem Cells
Mesenchymal stem cells (MSCs) are estimated to occur in bone marrow at a frequency of 0.001% to 0.0002% of nucleated marrow cells.84 Evidence suggests that the MSC lineage differentiation pathways are less strictly delineated (exhibit greater plasticity) than the HSC pathways.115 MSCs have the ability to differentiate into multiple lineages, including marrow stromal cells, adipocytes, osteoblasts, chondrocytes, fibroblasts, and myoblasts.95 Progenitor cells for a variety of peripheral tissue cell types are also present in bone marrow. Some studies suggest that MSCs may also produce epithelial cells, hepatocytes, and neuronal cells.81,115 Endothelial progenitor cells are present in bone marrow and blood; however, their origin remains to be clarified. Evidence has been presented suggesting an association with both MSCs and HSCs.24,118
Homing of Hematopoietic Stem Cells and Progenitor Cells to the Marrow
Homing is the process by which circulating HSCs and HPCs bind to the luminal surface of bone marrow endothelial cells, migrate through the endothelial cells, bind selectively to sites in the extravascular space, and begin the process of proliferation and differentiation. Homing of HSCs/HPCs is mediated by chemoattractants produced by endothelial cells and other cells in the microenvironment and by adhesion molecules expressed on the surfaces of HSCs/HPCs that bind to proteoglycans and glycoproteins on the surfaces of various marrow cells and the extracellular matrix.27
The chemokine (chemoattractant cytokine) CXCL12, also called stromal cell-derived factor-1 (SDF-1), is especially important in the homing of HSCs/HPCs, but other chemoattractants are also involved in this process. SDF-1 is produced by both marrow endothelial cells and stromal cells, and migration of HSCs/HPCs from blood to bone marrow occurs toward an SDF-1 gradient by virtue of an SDF-1 receptor CXCR4 expressed on these migrating cells. SDF-1 promotes the expression of CXCR4 and other adhesion molecules on the surface of HSCs/HPCs and induces transendothelial migration.27
HSCs/HPCs must be activated by locally produced factors (including SDF-1) for optimal transendothelial migration to occur. P- and E-selectin molecules (membrane-spanning, sugar-binding glycoproteins), expressed on bone marrow endothelial cells, bind to glycosylated ligands on HSCs/HPCs to promote an initial loose, rolling-type adhesion between HSCs/HPCs and endothelial cells in blood. Tight adhesion and migration through endothelial cells is dependent on integrin molecules—particularly the α4β1-integrin (very late antigen-4, VLA-4) on the surfaces of migrating cells—binding to their counterreceptors, especially vascular cell adhesion molecule-1 (VCAM-1), on endothelial cells.27
The first successful bone marrow transplants were done experimentally in dogs in the late 1950s.6 Because of the homing properties of HSCs, bone marrow transplants are performed by injecting bone marrow cells into the blood. In addition, HSCs/HPCs naturally circulate in blood. The physiologic mechanisms involved in the release of these hematopoietic cells from the bone marrow are not well defined, but HSC and HPC numbers can be increased markedly in blood following injection of growth factors such as granulocyte colony-stimulating factor (G-CSF).113 In fact, intravenous injection of growth factors is one approach used to collect increased numbers of stem cells from blood for human bone marrow transplantation.46
Hematopoietic Microenvironment
Blood cell production occurs throughout life in the bone marrow of adult animals because of the unique microenvironment present there. The hematopoietic microenvironment is a complex meshwork composed of stromal cells, endothelial cells, adipocytes, macrophages, subsets of lymphocytes, NK cells, osteoblasts, ECM components, and glycoprotein growth factors that profoundly affect HSC and HPC engraftment, survival, proliferation, and differentiation.1
Stromal cells and endothelial cells produce components of the ECM, including collagen fibers, basement membranes of vessels and vascular sinuses, proteoglycans, and glycoproteins. In addition to providing structural support, the ECM is important in the binding of hematopoietic cells and soluble growth factors to stromal cells and other cells in the microenvironment so that optimal proliferation and differentiation can occur by virtue of these cell-cell interactions (Fig. 3-8).1,106
Collagen fibers produced by stromal cells may not have direct stimulatory effects on hematopoiesis but rather are permissive, promoting hematopoiesis by forming a scaffolding around which the other elements of the microenvironment are organized. Hematopoietic cells can adhere to collagen types I and VI.22
Adhesion molecules (most importantly β1-integrins) on the surface of hematopoietic cells bind to ECM glycoproteins such as VCAM-1, hemonectin, fibronectin, laminin, vitronectin, and thrombospondin. The spectrum of the expression of adhesion molecules on hematopoietic cells that differentially bind to ECM glycoproteins varies with the type, maturity, and activation state of the hematopoietic cells. In addition to anchoring cells to a given microenvironmental niche, the binding of adhesion molecules on hematopoietic cells also plays a role in cell regulation directly by activating signal pathways for cell growth, survival, and differentiation or indirectly by modulating the responses to hematopoietic growth factors.22
A proteoglycan consists of a protein core with repeating carbohydrate glycosaminoglycans (GAGs) attached. Major proteoglycans in the marrow include heparan sulfate, chondroitin sulfate, hyaluronic acid, and dermatan sulfate. Proteoglycans enhance hematopoiesis by trapping soluble growth factors in the vicinity of hematopoietic cells and by strengthening the binding of hematopoietic cells to the stroma.28
Hematopoietic cells develop in specific niches within the marrow. During steady-state conditions, quiescent HSCs are concentrated near endosteal and trabecular bone, where osteoblasts help to regulate their numbers.152 HSCs and HPCs are also located near vascular sinuses, where they appear more active. HSCs and HPCs in this vascular niche likely have homeostatic roles during steady-state conditions.94 Erythroid cells develop around macrophages and megakaryocytes form adjacent to sinusoidal endothelial cells; granulocyte development is associated with stromal cells located away from the vascular sinuses.1,63,66
Hematopoietic Growth Factors
Proliferation of HSCs and HPCs cannot occur spontaneously but requires the presence of specific hematopoietic growth factors (HGFs); these may be produced locally in the bone marrow (paracrine or autocrine) or more remotely by peripheral tissues and transported to the marrow through the blood (endocrine). All cells in the hematopoietic microenvironment, including the hematopoietic cells themselves, produce HGFs and/or inhibitors of hematopoiesis.69 Some HGFs have been called poietins (erythropoietin [EPO] and thrombopoietin [TPO]). Other growth factors have been classified as colony-stimulating factors (CSFs) based on in vitro culture studies. Finally, some HGFs have been described as interleukins (ILs).67
Hematopoietic cells express receptors for more than one HGF on their surfaces. The number of each receptor type present depends on the stage of cell activation and differentiation. Binding of an HGF to its receptor results in a series of enzymatic reactions that generate transcription factors; these promote the synthesis of molecules that inhibit apoptosis, the formation of cell-cycle regulators (cyclins), and the synthesis of additional HGFs and their receptors.22,67 The pathways involved in generating lineage-restricted transcription factors is complex and beyond the scope of this text.22
HGFs vary in the type(s) of HSCs and/or HPCs that they can stimulate to proliferate. Factors are often synergistic in their effects on hematopoietic cells. In some instances, an HGF may not directly stimulate the proliferation of a given cell type, but may potentiate its proliferation by inducing the expression of membrane receptors for HGFs that do directly stimulate proliferation. Some glycoproteins, such as IL-1 and tumor necrosis factor-α (TNF-α), can modulate hematopoiesis indirectly by stimulating marrow stromal cells, endothelial cells, and T lymphocytes to produce HGFs. Different combinations of HGFs regulate the growth of different types of HSCs and/or HPCs.66
Intermediate-acting HGFs have broad specificity. IL-3 (multi-CSF), granulocyte-macrophage-CSF (GM-CSF), and IL-4 support proliferation of multipotent HPCs. These factors also interact with late-acting factors to stimulate the proliferation of a wide variety of committed progenitor cells. Late-acting HGFs have restricted specificity. Macrophage-CSF (M-CSF), G-CSF, EPO, TPO, and IL-5 are more restrictive in their actions. They have their most potent effects on committed progenitor cells and on later stages of development when cell lines can be recognized morphologically.67 TPO appears to be an exception. In addition to stimulating platelet production, it is important in maintaining a population of HSCs in their osteoblastic niche.8
Erythropoiesis
Primitive Erythropoiesis
Primitive erythropoiesis begins and predominates in the yolk sac but also occurs later in the liver. Primitive erythrocytes are large (more than 400 fL in humans), generally nucleated cells with high nuclear:cytoplasmic ratios. Their nuclei have open (noncondensed) chromatin and their cytoplasm contains predominantly embryonal hemoglobin (Hb) with a high oxygen affinity.117,133,138 In mammals as in nonmammalian species, primitive RBCs enter the blood as nucleated cells, but in contrast to nonmammalian species, enucleation can eventually occur in the circulation.70 These extruded nuclei circulate for a short time in the blood. They are surrounded by a small amount of cytoplasm and have been called pyrenocytes.97
A switch to definitive erythropoiesis occurs during fetal development. This results in the production of smaller cells that generally extrude their nuclei before entering the blood, produce fetal Hb (in some species) and adult Hb, and are highly dependent on EPO for proliferation.138
Hematopoietic Progenitor Cells and the Bone Marrow Microenvironment
The CMP gives rise to the MkEP, which can differentiate into megakaryocyte progenitors (MkPs) or erythroid progenitors (EPs). The production of EPs is stimulated by SCF, IL-3, GM-CSF, and TPO.67,78 The earliest EP is the burst-forming-unit erythrocyte (BFU-E), which differentiates into the colony-forming-unit erythrocyte (CFU-E). EPO is the primary growth factor involved in the proliferation and differentiation of CFU-Es into rubriblasts, the first morphologically recognizable erythroid cells. CFU-Es are more responsive to EPO than BFU-E cells because CFU-Es exhibit greater numbers of surface receptors for EPO.116
Marrow macrophages are important components of the hematopoietic microenvironment involved with erythropoiesis. Both early and late stages of erythroid development occur with intimate membrane apposition to central macrophages in “erythroid islands.” Several adhesion molecules on erythroid cells and macrophages, and extracellular matrix glycoproteins are important in forming these erythroid islands.26 Direct contact with these macrophages enhances the proliferation of erythroid precursors under basal conditions. Central macrophages may promote basal erythrocyte production by producing positive growth factors, including EPO; however, they may inhibit erythropoiesis by producing negative factors such as IL-1, TNF-α, transforming growth factor-β (TGF-β), and interferons (IFNs)-α, -β, and -γ in inflammatory conditions.25,145,154 The finding that EPO can also be produced by erythroid progenitors suggests that these cells may support erythropoiesis by autocrine stimulation.126 Although some degree of basal regulation of erythropoiesis occurs within the marrow microenvironment, humoral regulation is also important, with EPO production occurring primarily within peritubular interstitial cells of the kidney and various inhibitory cytokines being produced at sites of inflammation throughout the body.
Nutrients Needed for Erythropoiesis
Tetrahydrofolic acid, the active form of folic acid (a B vitamin), is needed for the transfer of single carbon-containing molecules in DNA and RNA synthesis. The physiologic mechanism of B12 involvement in erythrocyte production is not well understood, but it is related to folate metabolism. Cobalt is essential for the synthesis of B12 by ruminants.51
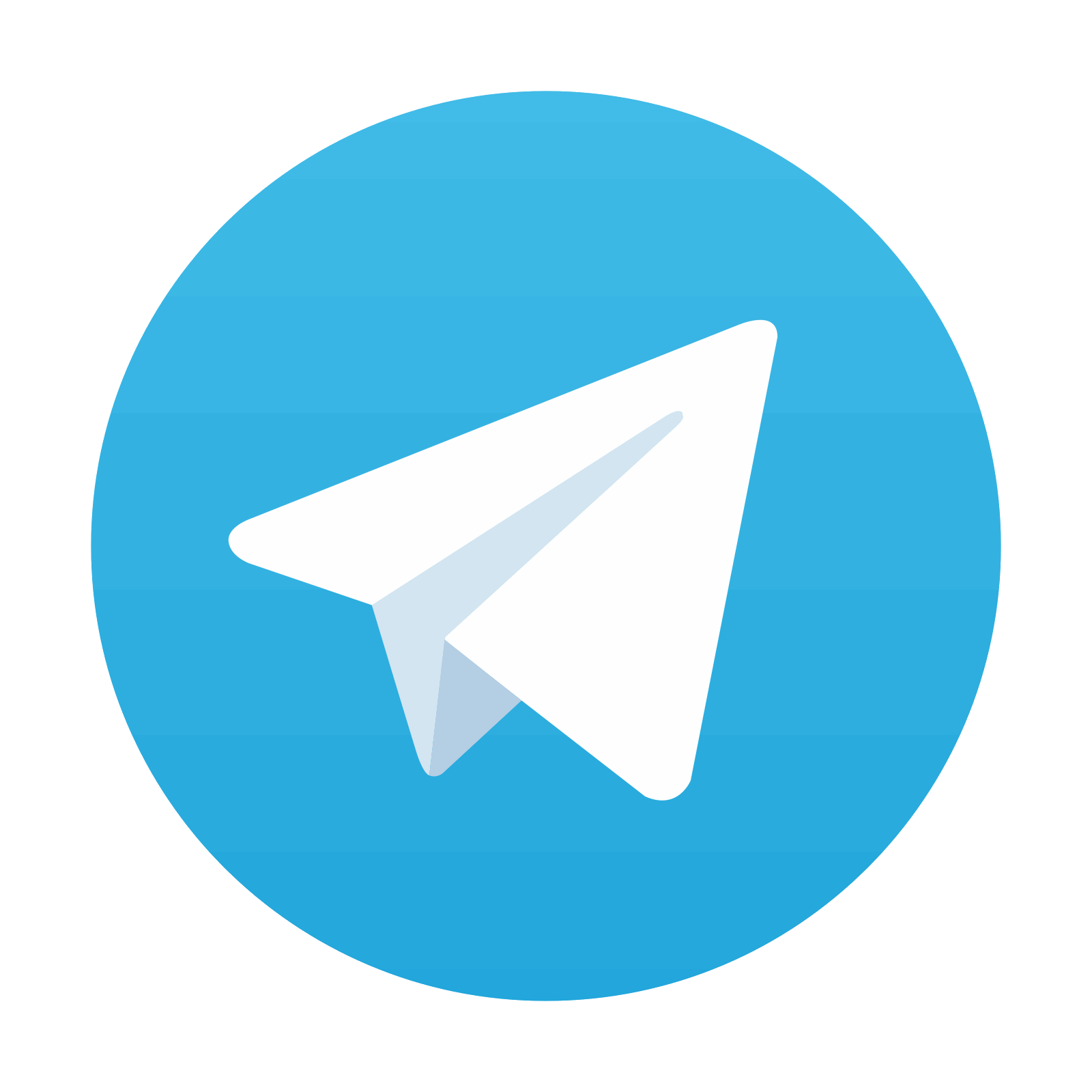
Stay updated, free articles. Join our Telegram channel

Full access? Get Clinical Tree
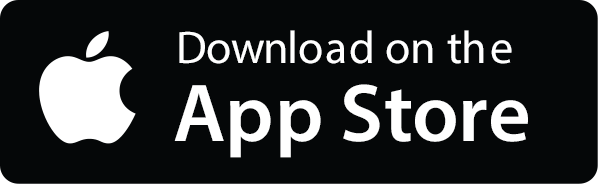
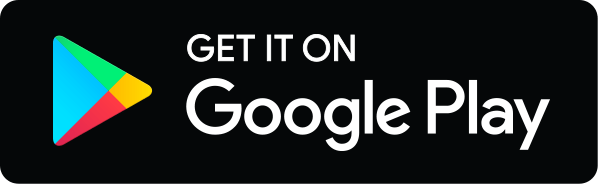