CHAPTER 8 Curtis W. Dewey & Daniel J. Fletcher Severe head trauma is associated with a high degree of morbidity and mortality in humans and animals. Death typically results from progressive increases in intracranial pressure (ICP). Brain injury in dogs and cats is most often due to automobile trauma; other causes include missile injuries (e.g. gunshot wounds), animal bites, and falls. Traumatic brain injury has been documented to occur in 25% of severe blunt trauma cases in dogs and cats and is negatively associated with survival. Considerable controversy exists concerning therapy for severely brain-injured patients and this field is one of intense research in human neurology/neurosurgery. This chapter contains recent information regarding therapy for head-trauma victims. Retrospective and prospective clinical data pertaining to the treatment of canine and feline head trauma are lacking; therefore most of the clinical recommendations in this chapter are based upon information from human head-trauma studies and experimental head-trauma investigations. Opinions differ concerning what constitutes appropriate therapy for the severely brain-injured pet. However, few would refute that treatment needs to be expedient and aggressive for the majority of these patients. The first veterinarian the brain-injured pet encounters after the traumatic incident will likely dictate the eventual outcome for that patient. Dogs and cats can function well with considerable loss of cerebral tissue, if given time to recover from a severe brain injury. The ultimate goal in head-trauma management is to return the patient to the role in society occupied prior to the injury. It is of utmost importance to alleviate brain swelling and prevent damage to vital brain-stem structures. Brain injury can be conceptually divided into primary and secondary injury. Primary brain injury occurs immediately following impact and initiates a number of biochemical processes, which result in secondary brain injury. Both primary and secondary brain injury contribute to increased ICP. A basic understanding of the mechanisms of brain-tissue damage following injury and ICP dynamics is essential to logical therapy of the severely head-traumatized patient. Primary brain injury This type of injury refers to the physical disruption of intracranial structures that occurs immediately at the time of the traumatic event. Such injury includes direct damage to brain parenchyma, such as contusions, lacerations, and diffuse axonal injury. Damage to blood vessels may result in intracranial hemorrhage (Fig. 8.1) and vasogenic edema. Skull fractures can contribute to continued trauma to the brain parenchyma and blood vessels, especially if they are unstable (Fig. 8.2). The extent of primary brain injury is a function of the force of impact. Acceleratory and deceleratory forces of both the impacting object(s) and the intracranial contents will affect overall tissue damage. Direct parenchymal damage associated with primary brain injury is generally beyond the control of the clinician. However, stabilization of skull fractures and evacuation of intracranial hemorrhage may decrease the morbidity associated with these primary injuries. Figure 8.1 Clinically important forms of intracranial hemorrhage. Subarachnoid hemorrhage would occur as diffuse hemorrhage between the pia and arachnoid layers. Figure 8.2 Three-dimensional CT reconstruction of a skull fracture in a dog that experienced severe head trauma. (Reproduced with permission from Dr. Charles Vite.) Secondary brain injury In addition to continued hemorrhage and edema, the damage caused by the primary brain injury activates a number of interrelated biochemical pathways that act in concert to perpetuate further brain-tissue damage and subsequent increases in ICP (Box 8.1). Adenosine triphosphate (ATP) depletion disrupts the maintenance of cellular ionic homeostasis. Sudden, uncontrolled intracellular influx of sodium (Na+) and calcium (Ca++) occurs. Cellular swelling (cytotoxic edema) and depolarization result. The uncontrolled depolarization leads to the release of large amounts of glutamate, an excitatory neurotransmitter, into the extracellular environment. Glutamate causes further increases in intracellular Ca++ levels. Elevated Ca++ levels activate a number of tissue-damaging pathways, including the arachidonic acid cascade (phospholipase A2 activation) and the xanthine oxidase (free-radical producing) pathway. Iron (Fe++) is a vital cofactor in the xanthine oxidase pathway, and free-radical species generated via the Fenton reaction (e.g. hydroxyl and superoxide radicals) are preferentially damaging to cell membranes containing high levels of polyunsaturated fats and cholesterol. Brain tissue is rich in both Fe++ and membranes with high levels of PUFAs and cholesterol. Intraparenchymal hemorrhage also increases the amount of Fe++ available for the perpetuation of oxidative damage. Free-radical species are thus particularly damaging to neuronal membranes and probably play a major role in secondary brain injury. Their production is also induced by ischemia, arachidonic acid metabolites, catecholamine oxidation, and activated neutrophils. Other secondary autolytic processes induced after severe head trauma include the complement, kinin, and coagulation/fibrinolytic cascades. Elevated levels of nitric oxide (NO) and various cytokines (e.g. tumor necrosis factor, interleukins) also contribute to parenchymal injury in the damaged brain. Most of the mediators of tissue damage produced by these various reactions perpetuate their own continued production as well as the production of other mediators. The maintenance of an ischemic environment perpetuates the above-mentioned processes and also leads to the accumulation of lactic acid (via anaerobic glycolysis). Lactic acid accumulation leads to further damage to brain tissue. Hypotension and hypoxemia, extracranial conditions that are common in the traumatized patient, can worsen brain ischemia and thereby enhance the events responsible for secondary brain injury. The result of these secondary processes is increased ICP. Unlike primary brain injury, the clinician has some control over secondary brain injury. Intracranial pressure (ICP) dynamics Intracranial pressure is the pressure exerted by tissues and fluids within the cranial vault. Normal ICP values for dogs and cats range between 5 and 12 mmHg. Cerebral perfusion pressure (CPP) is a primary determinant of cerebral blood flow (CBF) and hence brain oxygenation and nutritional support. CPP is defined by the following equation: The normal contents of the cranial cavity include brain parenchyma, blood, and cerebrospinal fluid (CSF). In the normal animal, these components exist in equilibrium with each other and ICP remains within normal limits. Between the MABP extremes of 50–150 mmHg, ICP remains constant. This phenomenon is called pressure autoregulation. Pressure autoregulation serves to link systemic blood pressure changes to brain vascular tone. If MABP rises, vasoconstriction occurs in the brain; if MABP falls, vasodilation occurs in the brain. In the normal animal, the former scenario prevents ICP from rising by decreasing CBF, and in the latter, prevents ICP from falling by increasing CBF. Chemical autoregulation refers to the direct responsiveness of brain vasculature to the partial pressure of carbon dioxide in arterial blood (PaCO2); elevated PaCO2 levels cause cerebral vasodilation, whereas decreased PaCO2 levels cause cerebral vasoconstriction. Both forms of autoregulation often remain intact in people with severe head injury, but pressure autoregulation may be compromised in approximately 30% of patients. In some of these individuals, the lower MABP extreme may become “reset” to a higher value, resulting in significantly decreased blood flow to the brain with even mild systemic hypotension. With severe head trauma, both intracranial hemorrhage and edema can add to the volume of the intracranial compartment. Due to the inexpansible nature of the skull, one or more components of the cranial cavity must accommodate for the increased volume, or increased ICP will result. This accommodation or volume buffering is accomplished by fluid shifts in the brain vasculature and CSF pathways and is referred to as intracranial compliance. Compliance is expressed as the change in volume per unit change in pressure. Intracranial compliance has limitations, and decreases as ICP increases. If intracranial volume increases beyond the abilities of compensatory mechanisms, progressively larger increases in ICP result per unit of volume increase (Fig. 8.3), CPP is compromised, and ischemic death of brain tissue occurs. In cases of severe head trauma, intracranial compliance often is quickly exhausted. If MABP decreases (hypotension), especially in combination with hypoxemia, the brain vasculature will vasodilate in an effort to preserve blood flow. The increase in blood volume increases ICP, but CPP remains inadequate. In addition, the secondary autolytic processes occurring in the injured brain are enhanced by hypotension and hypoxemia, and further brain injury and edema occur with a resultant rise in ICP. Figure 8.3 Typical pressure/volume curve for the intracranial compartment. (Dewey, 2000. Reproduced with permission from Elsevier.)17 Initial physical assessment of the severely brain-injured patient focuses on imminently life-threatening abnormalities. Many patients suffering severe head trauma present to the clinician in a state of hypovolemic shock. Do not be in a rush to focus initially on the patient’s neurologic status; it may well improve once the shock state is corrected. Remember that traumatized, hypovolemic patients with no appreciable brain injury often exhibit depressed mentation, due primarily to the hypotensive state. The clinician must first focus on the ABCs of trauma management (airway, breathing, cardiovascular status). In doing so, the brain will benefit as well as the rest of the patient. Quick assessment tests (QATs)—including packed cell volume (PCV), total solids (TS), Azostix (AZO), and blood glucose (BG)—are part of the initial patient assessment. Since hypovolemia and hypoxemia are strongly correlated with elevated ICP and increased mortality in human head-trauma victims, they need to be addressed immediately. Table 8.1 Intravenous fluid therapy and recommended doses for head trauma patients. There is often concern that aggressive intravenous fluid therapy to counteract hypotension in the brain-injured patient may aggravate brain edema. There is both evidence to support and evidence to refute this concern. Because of this concern, there have been recommendations to volume-limit victims of severe head trauma. Such recommendations are not only unfounded, but strictly contraindicated. There is no debate over the disastrous consequences to the injured brain if hypotension is allowed to persist. Hypotension has been repeatedly shown to be a reliable predictor of sustained elevations of ICP and increased mortality in human head-trauma victims. Blood pressure must be restored to normal levels as soon as possible. A patient with a systolic blood pressure of less than 120 mmHg is considered hypotensive. Some volume replacement fluids (hetastarch, hypertonic saline) afford some protection to the edematous brain, even if used with large volumes of crystalloids (LRS, 0.9% NaCl). Hetastarch and hypertonic saline can improve MABP and thus CPP without exacerbating brain edema. If the patient is anemic, whole-blood or packed red blood cell (pRBC) transfusion may assist in maintaining normovolemia as well as adequate tissue oxygenation by improving blood oxygen content, the major determinant of which is hemoglobin concentration. Fluid support may include one or more of the following choices: Oxygenation and hyperventilation Hyperoxygenation is recommended for most acutely brain-injured animals. Oxygenation status of a head-trauma victim can be initially assessed based upon breathing rate and pattern, mucous membrane and tongue color, and thoracic auscultation. Pneumothorax and pulmonary contusions are common sequelae of trauma, and need to be addressed if present. It should be noted that, in the face of increased respiratory rate and effort, lung sounds may not consistently be decreased on auscultation in patients with pneumothorax. A rapid, shallow breathing pattern, pale oral mucous membranes, and evidence of respiratory distress should raise the clinician’s index of suspicion for the presence of pneumothorax. Thoracentesis should be done in any trauma patient in whom there is a suspicion of pneumothorax, and should be considered a diagnostic test as well as a therapeutic intervention. If negative pressure cannot be obtained via thoracentesis, a chest tube should be placed immediately. If arterial blood gas analysis is available, the partial pressure of oxygen in arterial blood (PaO2) should be maintained at or above 90 mmHg for dogs, and 100 mmHg for cats. Pulse oximeters are extremely useful and relatively accurate estimators of oxygenation status. However, the reliability of pulse oximeters varies with model used, with the PaO2 level (pulse oximeters may overestimate oxygenation status at lower PaO2 levels), and with the patient’s hemodynamic status. In general, oxyhemoglobin saturation values (SpO2
Head-Trauma Management
Introduction1, 17, 20, 22, 39, 56, 64, 67
Pathophysiology of head trauma5, 6, 8, 17, 20, 22, 26, 27, 32, 34, 39, 49, 56, 64, 66, 67
Initial assessment and emergency treatment2, 9, 11, 17, 19, 20, 22, 24, 32, 36, 46, 50, 53, 57, 59, 62–64, 74, 78, 79 (Video 17)
Fluid type
Recommended dose
Isotonic crystalloid (0.9% NaCl preferred)
20–30 ml/kg dogs 10–20 ml/kg cats
Administered over 15–20 min
Reassess after
Synthetic colloid (e.g. 6% hydroxyethyl starch)
5–10 ml/kg
Administered over 15–20 min
Reassess after
7.5% sodium chloride
4 ml/kg
Administered over 15–20 min
Reassess after
Always follow with crystalloid therapy
3% sodium chloride
5.4 ml/kg
Administered over 15–20 min
Reassess after
Always follow with crystalloid therapy
1:2 ratio of 23.4% sodium chloride and 6% hydroxyethyl starch or other synthetic colloid
4 ml/kg
Administered over 15–20 min
Reassess after
Always follow with crystalloid therapy
Packed red blood cells
∼1 ml/kg
Administer over less than 4 hrs/unit
Target normalization of perfusion parameters and PCV = 25–30%
Whole blood
∼2 ml/kg
Administer over less than 4 hrs/unit
Target normalization of perfusion parameters and PCV = 25–30%
Fresh frozen plasma
10–15 ml/kg
Administer over less than 4 hrs/unit
Target normalization of coagulation times
Stay updated, free articles. Join our Telegram channel

Full access? Get Clinical Tree
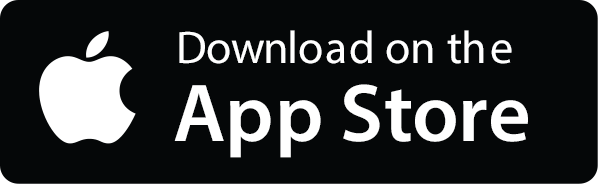
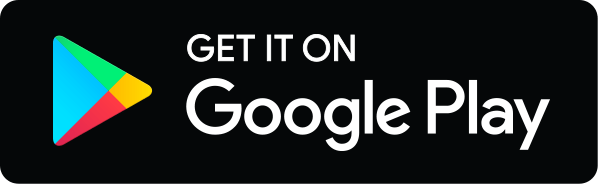