Genetics of mare fertility
After REEL and gonadal dysgenesis that are caused by chromosomal abnormalities, the most common subfertile phenotype in mares is the failure to become pregnant (Coutinho da Silva, 2008). Genetic causes of pregnancy failure are unknown. Currently, there are no molecular tools for the diagnostics of mare fertility or for proper evaluation of oocytes and embryos prior their use for in vitro reproductive technologies.
Genetics of stallion fertility
Stallions are typically selected on the basis of their ancestry, athletic performance, and overall appearance, but not for their reproductive potential (Colenbrander, Gadella, & Stout, 2003). As a result, reduced fertility among breeding stallions has emerged as one of the major problems (Leeb et al., 2005; Leeb, 2007). For example, in two retrospective studies, 36–43% of prospective breeding stallions did not pass reproductive soundness test (Blanchard & Varner, 1997; Woods et al., 2000).
Genetic studies of stallion fertility started with microsatellite heterozygosity analysis (Aurich, Achman, & Aurich, 2003) and SNP search in candidate genes (Giese et al., 2002; Hamann et al., 2007; Giesecke et al., 2009a, 2010a; Giesecke et al., 2009b). The goal was to identify genetic markers associated with various reproductive phenotypes and semen parameters (Table 13.1). Not much success accompanied microsatellite analysis: genotyping of 12 markers in 110 Noriker stallions did not reveal any correlation between heterozygosity and semen parameters such as sperm count and motility (Aurich et al., 2003).
One of the first candidate gene studies dissected the molecular organization and function of AEG1 locus, also known as cystein-rich secretory protein 1 or CRISP1, which is thought to be involved in sperm-egg fusion (Giese et al., 2002). It was determined that the gene comprises of eight exons and has three possible transcription start sites. Sequencing the exons and their flanking sequences in 16 horses of 9 different breeds identified 17 SNPs of which 3 were exonic and 1 changed the amino acid sequence. However, the role of AEG1 in stallion fertility remains unclear because no attempts have been made to correlate AEG1 polymorphism with various reproductive phenotypes.
Another group of studies analyzed association of sequence polymorphism of selected candidate genes with fertility data in a population of reproductively normal Hanoverian warmblood stallions (Giesecke et al., 2009a, 2010a; Giesecke et al., 2009b; Hamann et al., 2007). Reproductive performance of the stallions was evaluated based on pregnancy rate per estrus of mares. The genes CRISP3 (Hamann et al., 2007), PRLR (Giesecke et al., 2009a), INHBA (Giesecke et al., 2010a), and SPATA1 (Giesecke et al., 2009b) were examined and the results suggested association of sequence variants of all four genes with stallion fertility (Table 13.1).
Many more gene loci potentially involved in regulating male fertility have been proposed using extensive data mining from PubMed (http://www.ncbi.nlm.nih.gov/pubmed/) and Web of Science (http://apps.isiknowledge.com/), followed by in silico analysis of the most reliable candidate genes (Ogorevc, Dovc, & Kunej, 2010). Considering data from many mammalian species, 835 candidate genes for mammalian male fertility were identified. Of these, 33 genes were proposed as the most promising candidates because they were reported in at least two different species or using two or more approaches. According to this, one of the strongest candidate genes for male fertility in multiple species, including the horse, is prolactin receptor (PRLR). A similar data search was recently conducted to identify candidate genes specific for stallion fertility (Giesecke et al., 2010b). The authors proposed 37 genes with associated SNPs and microsatellites for linkage and GWA studies. Surprisingly, only 10 candidate male fertility genes were common between the two data sets, thus further underlining the complexity of the genetic component of male reproduction.
Due to the large number of genes involved, the molecular research of fertility requires genome-wide (GW) approaches. Thus, great expectations are placed on the use of horse whole genome sequence information (Wade et al., 2009), the SNP50/SNP74 beadchips (McCue et al., 2009; Mickelson, personal communication), and GW linkage and association analysis. However, subfertile phenotypes do not facilitate collection of large numbers of samples or pedigrees for statistically sound GWA and linkage studies. Difficulties are also encountered in precise clinical characterization of subfertile phenotypes because in most cases the causes of reduced fertility are unknown or idiopathic (Blanchard, Johnson, & Roser, 2000; Turner & Casas-Dolz, 2002). This is probably why GW molecular research has been initiated only for two conditions: cryptorchidism and impaired acrosomal reaction.
Cryptorchidism
Cryptorchidism, or failure of testes to descend, affects about 8% of live male births in horses, and is more frequent in ponies, draft horses, and breeds like Percheron, American Saddlebred, and Quarter Horse (Hayes, 1986). Cryptorchidism can occur as a single defect or as a part of complex congenital abnormalities accompanying intersex conditions or chromosomal aneuploidies (Constant et al., 1994; Chowdhary & Raudsepp, 2000). In all cases, failure of testes descent affects normal testicular functions and can result in reduced fertility or infertility. Etiology of cryptorchidism is complex, involves interaction of environmental, genetic, and epigenetic factors, and is, as yet, not well understood. Studies in mouse and human suggest complex genetic regulation of the condition involving genes for gubernaculum development and androgen signaling pathway (Ferlin et al., 2008; Foresta et al., 2008). Indeed, a missense mutation in relaxin/insulin-like family peptide receptor 2 (RXFP2) was recently associated with cryptorchidism in mouse (Harris et al., 2010). Genetic studies of the condition in horses are limited to a linkage analysis using a Thoroughbred pedigree of 23 cryptorchids and 24 unaffected horses (Diribarne et al., 2009). Gentoyping of 16 microsatellite markers for seven candidate genes, namely AR, INSL3, RXFP2, CALCA, HOXA10, NR5A1, and ESR1, did not reveal any association with the cryptorchid phenotype. In contrast, recent gene expression profiling showed significant (P < 0.001) up-regulation of RXFP2, FGF10, HOXA10, and down-regulation of INSL3, HOXA11, and HSD3B1 in cryptorchid compared to normal testes (our unpublished data). It is likely that the observed differences in gene expression reflect the consequences rather than the primary genetic causes of abnormal testicular descent. Research is currently under way to explore the epigenetic landscape of cryptorchidism by comparing global DNA methylation levels of selected candidate genes between normal and cryptorchid testes, as well as to study the possible involvement of genomic copy number variation in the etiology of cryptorchidism (our ongoing research).
Impaired acrosomal reaction
Impaired acrosomal reaction (IAR) is a reproductive disorder characterized by the inability of sperm to perform acrosomal reaction, resulting in reduced fertility. The condition has been found in Thoroughbreds, and currently efforts are made to identify the genomic region(s) associated with the condition using the equine SNP50 (McCue et al., 2009) and the 21,000-element expression oligoarray (Bright et al., 2009). GW association analysis reveals statistically significant association of the subfertile phenotype to ECA13p around 8–9 Mb position (Raudsepp et al., 2011). Notably, some of the differentially expressed genes, as detected by microarray analysis, originate from the same region. Re-sequencing of two most significant candidate genes has identified a few statistically significant (p<0.05) amino acid changing SNPs in FKBP6, suggesting this as a susceptibility locus for IAR. With the exception of the Y chromosome (see Chapter 5), this is the first report about a direct association of a genomic region with a reproductive disorder in horses.
Functional Genomics of Reproduction
Functional genomics of reproduction studies gene expression profiles in male and female reproductive tissues and the zygote, to identify protein coding genes and other functional elements (i.e., small and long non-coding regulatory RNAs) that are critical for male and female fertility. The studies seek for understanding the functions and interactions of genes during different stages of sexual development, sexual maturation, and reproduction, both in normal and subfertile individuals. One of the main goals is to identify functional signatures that can lead to the development of molecular tests for fertility evaluation. Gene expression studies typically do not require the procurement of hundreds of samples, as is necessary for linkage and GWA analyses, and are therefore more feasible for reproduction-related research. Functional analysis can be carried out on individual genes of interest or across the whole genome. The former is typically based on reverse transcriptase (RT) and quantitative real-time (qRT) PCR (Das et al., 2010a; Das et al., 2010b) or differential display (Turner & Casas-Dolz, 2002). GW analysis, on the other hand, uses whole genome expression arrays (Ing et al., 2004; Das et al., 2010b) or direct mRNA sequencing, also known as RNA-Seq (Coleman et al., 2010; Das et al., 2011c).
Gene expression studies in the mare
Similarly to molecular genetics, functional genetics of mare reproduction lags far behind that of the stallion. A few studies have analyzed the expression of selected genes in ovaries and the uterus. It has been shown that high expression level of steroidogenic acute regulatory (StAR) gene in ovaries is required during the early stages of pregnancy (Watson, Thomson, & Howie, 2000; Watson et al., 2004). Ovarian expression of dopamine receptors 1 and 2 (D1R and D2R) suggests that dopamine can act directly on ovarian tissues through its interaction with dopamine receptors during fertilization (King et al., 2005). Additionally, there are preliminary studies on differential gene expression in blastocysts (Paris et al., 2010) and the endometrium during pregnancy recognition (Bruemmer et al., 2010), and the early stages of pregnancy (Merkl et al., 2010). Despite the important role of ovarian genes in follicular maturation and early embryogenesis in human, mouse, and chicken (Juengel et al., 2004; Elis et al., 2009), no gene expression studies have, as yet, been initiated in equine oocytes.
Functional genomics of the stallion
The majority of functional studies in the stallion have focused on a small number of selected candidate genes comparing their transcription and/or protein expression profiles at different time points in reproductive and somatic tissues. For example, it was determined that AEG1 is highly expressed in epididymis but not in testis (Giese et al., 2002). Interesting findings were reported for the expression of anti-Müllerian hormone (AMH) which induces regression of Müllerian ducts during male fetal development (Ball et al., 2008). Equine AMH is highly expressed in Sertoli cells of fetal, neonatal, and prepubertal testes, but not in normal adult testes. Notably, AMH is also expressed in cryptorchid testes (in animals up to 3–4 years of age), in Sertoli cell tumors, and male intersex gonads, making it a useful biomarker for the detection of these conditions in the horse (Ball et al., 2008). A novel testis-specific equine serine/threonine kinase, STK31 was recently discovered in stallion testes (Sabeur et al., 2008). Detailed characterization of the RNA transcript (3.1 kb), the functional domains of the protein (protein kinase and putative RNA-binding domains), and the protein localization in the equatorial segment of testicular spermatozoa suggest that STK31 may have a role in reorganization of sperm chromatin during spermiogenesis. Systematic studies have also been carried out on horse Y chromosome genes (Paria, 2009) by determining their expression in testis and somatic tissues, and generating full-length cDNA sequences for selected genes of interest (see Chapter 5 for more details).
Promising solutions for functional genomics of reproduction are offered by global gene expression microarrays (Farber & Lusis, 2008) and RNA-Seq (Wang, Gerstein, & Snyder, 2009). The well-annotated 21,000-element oligoarray for the horse (Bright et al., 2009) is currently the most cost-effective tool for functional analysis of tens of thousands of genes from a small number of normal and affected individuals, providing a critical link between the genes and the phenotype. RNA-Seq technology, on the other hand, is the most powerful method for transcriptional profiling of any cell or tissue (Shendure, 2008; Wang et al., 2009; Montgomery et al., 2010). This includes the identification of novel genes, alternative transcripts, and small and long non-coding regulatory RNAs.
Testis transcriptome
Application of microarray technology for transcriptional profiling of stallion testis (Laughlin et al., 2002; Ing et al., 2004) can be considered the formal beginning of functional genomics in horses. In these studies, gene expression profiles in dark and light testicular tissues of three prepubertal colts were analyzed using a human WG cDNA array. Assessment of transcriptional profiles of 9,132 human genes showed that only 93 genes (1.3%) are differentially expressed between the light and dark testicular parenchyma.
Recently, testis transcriptome of reproductively normal stallions was studied using the 21,000-element equine whole genome expression oligoarray (Das et al., 2010b) and direct mRNA sequencing (Coleman et al., 2010). The two studies closely agree about the overall number of testis mRNA transcripts, which is between 11,000 and 15,000 according to microarray analyses and 12,013 according to RNA-Seq. Efforts are currently made to generate a comprehensive catalog of testis transcripts characteristic to a normal stallion. This includes annotations, expression profiles, and putative functions of all detected genes. The catalog will serve as an important foundation for the study of functional changes in the testis of subfertile animals, leading to the identification of genes that are critically involved in stallion fertility. Furthermore, recently optimized protocol for RNA isolation from stallion testis biopsies using a 14 gauge 22 mm deep needle and yielding about 12 micrograms of sequencing quality total RNA (Das et al., 2010a) offers an alternative to excision surgery for sample procurement.
Sperm transcriptome
Despite the achievements of testis transcriptome analysis, difficulties remain regarding the procurement of samples, because conducting excision surgery or biopsy on the testis of prospective breeding animals is potentially harmful. A promising solution is to use sperm instead of testis as the source of RNA. This builds on recent discoveries in humans showing that ejaculated sperm contain a rich repertoire of RNAs, including mRNAs, and small and long non-coding regulatory RNAs (Ostermeier et al., 2002; Krawetz, 2005; Ostermeier et al., 2005a; Ostermeier et al., 2005b; Boerke et al., 2007). Thus, the sperm’s contribution to the oocyte is not only the paternal DNA but also a complex host of RNAs. Some sperm mRNAs might be needed for early embryonic development (Boerke et al., 2007) and for protein synthesis during capacitation using mitochondrial-type ribosomes (Gur & Breitbart, 2006, 2007, 2008). Sperm microRNAs might serve as transgenerational signals for heritable epigenetic modifications (Cuzin & Rassoulzadegan, 2010). While the exact functions of sperm RNAs are still a matter of debate, most researchers agree that the majority of sperm mRNAs are historical records of spermatogenesis, and that sperm RNA profiles can be used as a measure of male fertility (Krewetz, 2005; Boerke et al., 2007; Platts et al., 2007). Most importantly, the use of sperm instead of testis as the source of RNA will grant noninvasive and feasible procurement of samples.
Sperm transcriptome studies were recently initiated in stallions. As a first step, total RNA isolation methodology from sperm was optimized for the horse (Das et al., 2010a; Sudderth et al., 2010). This was necessary because sperm typically contain only 10–20 fg of RNA; the ejaculates contain somatic cells and immature sperm that must be removed before RNA extraction; sperm are depleted of ribosomal RNAs, thereby negating the use of rRNA integrity as a marker of quality; and differences between species in sperm attributes and packaging require adjustments in RNA isolation protocols. The optimized protocol allows isolation of about 2 micrograms of hybridization- and sequencing-quality total RNA from 100 million sperm (Das et al., 2010a). Comparison of the RNA quality and quantity with different sperm-processing and storage conditions showed that fresh semen remains the gold standard for sample submission. Processing methods utilizing an extender provide a useful alternative for shipping samples from a distance for evaluation, and flash-frozen semen is the least desirable submission type, but may be utilized if necessary (Sudderth et al., 2010).
Prior to the use of sperm RNA as a diagnostic tool for stallion fertility, the sperm RNA profiles that define the normal fertile male must be determined. Preliminary data about the number, identity, biological functions, and transcriptional profiles of mRNAs and regulatory non-coding RNAs in stallion sperm were recently obtained using microarray hybridization (Das et al., 2010b) and RNA-Seq (Das et al., 2011c).
Microarray analysis of stallion sperm identified more than 6,000 mRNA transcripts, which is roughly half of the number of mRNAs in stallion testis (Coleman et al., 2010; Das et al., 2010b). Notably, about 2.4% of sperm transcripts are sperm-specific and not detected in testis (Table 13.2), while about 200 sperm mRNAs show differential expression between the sperm and the testis (Table 13.3). Many sperm transcripts are associated with membranes and are involved in molecular functions related to G-protein coupled receptor (GPCR) activities. This might be important for fertilization because GPCRs are implicated in sperm chemotaxis, capacitation, and acrosome reaction.
Table 13.2 Twenty selected genes that are present in sperm (signal-to-noise ratio SNR ≥ 2) but not in testis mRNA (Das et al., 2010b; Das et al., 2011b).
Table 13.3 Selected differentially expressed genes between stallion sperm and testis; FC – fold change (Das et al., 2010b; Das et al., 2011b).
In another study, total RNA from the sperm of one reproductively normal stallion was sequenced using SOLiD (Applied Biosystems) platform (Das et al., 2011c). Altogether, 65 million 35-base-pair reads were generated and aligned with the horse draft assembly EcuCab2 (Wade et al., 2009). Sequence map locations were determined for 19,257 reads, of which 31% were identified as dbESTs, mRNAs and 33 known microRNAs, while 69% had no annotation in EcuCab2. Like in microarray analysis, many of the transcripts detected by RNA-Seq show direct relevance to a variety of known sperm functions (Table 13.4).
Table 13.4 Selected transcripts in stallion sperm as detected by RNA-Seq. (Average coverage corresponds to sequence tag count and shows the expression level) (Das et al., 2011b).
It is anticipated that transcriptional profiling of stallion sperm will lead to the identification of functional signatures for high- and low-fertility stallions, so that noninvasive molecular tests for stallion fertility can be developed. Global transcriptional profiling of stallion sperm will also serve as a valuable non-rodent model for dissecting the genetics of male fertility in humans and other mammals.
Summary and Future Prospects
Genomics of equine reproduction is a challenging area of research that encompasses a wide range, from chromosomes to fine-tuning of gene expression. The stability and structural integrity of chromosomes is certainly a key component of normal sexual development and fertility in horses, and cytogenetic analysis will continue to be an essential part of clinical tests for subfertile animals. While the role of chromosomal abnormalities in equine fertility is well established, the knowledge about the fertility genes, their structure, function, and associated phenotypes is still limited. Furthermore, almost nothing is known about the role of structural genomic rearrangements, copy number variants (CNVs), gene expression regulation, and epigenetic mechanisms in male and female fertility. This is why great expectations are placed on the use of new genomics tools such as SNP beadchips (McCue et al., 2009; J. Mickelson, personal communication), the recently constructed WG tiling array (Qu et al., 2011), the expression microarrays (Bright et al., 2009), various next-generation sequencing platforms, and improved bioinformatics methods. It is anticipated that these tools will take equine genome analysis to levels that allow the detection of submicroscopic genomic rearrangements underlying the currently unknown causes of subfertility, will lead to the discovery of reproduction-related mutations in nuclear and mitochondrial genes, and will identify genetic and epigenetic regulatory mechanisms critical for stallion and mare reproduction. However, all the advanced tools will be of little value without the establishment of collections of phenotypically well-characterized samples. Thus, increased interaction between the geneticists and clinicians remains as one of the most critical factors for any success.
Acknowledgments
The research on the genomics of stallion fertility has been supported by the LINK Endowment and by the American Quarter Horse Research Foundation.
References
Aurich, C., Achmann, R., & Aurich, J. E. (2003). Semen parameters and level of microsatellite heterozygosity in Noriker draught horse stallions. Theriogenology, 60, 371–378.
Ball, B. A., Conley, A. J., Grundy, S. A., Sabeur, K., & Liu, I. K. (2008). Expression of anti-Mullerian hormone (AMH) in the equine testis. Theriogenology, 69, 624–631.
Bannasch, D., Rinaldo, C., Millon, L., Latson, K., Spangler, T. et al. (2007). SRY negative 64,XX intersex phenotype in an American saddlebred horse. Veterinary Journal, 173, 437–439.
Barnett, K. R., Schilling, C., Greenfeld, C. R., Tomic, D., & Flaws, J. A. (2006). Ovarian follicle development and transgenic mouse models. Human Reproduction Update, 12, 537–555.
Blanchard, T. L., Johnson, I., & Roser, A. J. (2000). Increased germ cell loss rates and poor semen quality in stallions with idiopathic testicular degeneration. Journal of Equine Veterinary Science, 20, 263–265.
Blanchard, T. L., & Varner, D. D. (1997). Evaluation breeding soundness in stallions. 5. Predicting potential fertility. Veterinary Medicine, 97, 815–818.
Blue, M. G. (1981). A cytogenetical study of prenatal loss in the mare. Theriogenology, 15, 295–309.
Boerke, A., Dieleman, S. J., & Gadella, B. M. (2007). A possible role for sperm RNA in early embryo development. Theriogenology, 68(Suppl. 1), S147–S155.
Bondy, C. A., & Cheng, C. (2009). Monosomy for the X chromosome. Chromosome Research, 17, 649–658.
Bowling, A. T., Millon, L., & Hughes, J. P. (1987). An update of chromosomal abnormalities in mares. Journal of Reproductive Fertility, 35(Suppl.) 149–155.
Bright, L. A., Burgess, S. C., Chowdhary, B., Swiderski, C. E., & McCarthy, F. M. (2009). Structural and functional-annotation of an equine whole genome oligoarray. BMC Bioinformatics, 10(Suppl. 11), S8.
Brito, L. F. C., P. L., S., Durkin, K., Chowdhary, B. P., Turner, R. M. et al. (2008). Autosomic 27 trisomy in a standardbred colt. Journal of Equine Veterinary Science, 28, 431–436.
Bruemmer, J. E., Bouma, G. J., Hess, A., Hansen, T. R., & Squires, E. L. (2010). Gene expresiion in the equine endometrium during maternal recognition of pregnancy. Animal Reproduction Science, 1215, S285–S287.
Bugno-Poniewierska, M., Jablonska, Z., & Slota, E. (2009). Modification of equine sperm chromatin decondensation method to use fluorescence in situ hybridization (FISH). Folia of Histochemical Cytobiology, 47, 663–666.
Bugno, M., Slota, E., & Koscielny, M. (2007). Karyotype evaluation among young horse populations in Poland. Schweiz Archiv Tierheilkd, 149, 227–232.
Bugno, M., Slota, E., Wieczorek, M., Yang, F., Buczynski, J. et al. (2003). Nonmosaic X trisomy, detected by chromosome painting, in an infertile mare. Equine Veterinary Journal, 35, 209–210.
Buoen, L. C., Zhang, T. Q., Weber, A. F., & Ruth, G. R. (2000). SRY-negative, XX intersex horses: The need for pedigree studies to examine the mode of inheritance of the condition. Equine Veterinary Journal, 32, 78–81.
Carnevale, E. M. (2008). The mare model for follicular maturation and reproductive aging in the woman. Theriogenology, 69, 23–30.
Carrell, D. T. (2007). The genetics of male infertility in the era of genomics. In D. T. Carrell (ed.), The genetics of male infertility (pp. 3–27). Totowa, NJ: Humana Press.
Carrell, D. T. (2008). The clinical implementation of sperm chromosome aneuploidy testing: Pitfalls and promises. Journal of Andrology, 29, 124–133.
Cederroth, C. R., Pitetti, J. L., Papaioannou, M. D., & Nef, S. (2007). Genetic programs that regulate testicular and ovarian development. Molecular and Cellular Endocrinology, 265–266, 3–9.
Chandley, A. C., Jones, R. C., Dott, H. M., Allen, W. R., & Short, R. V. (1974). Meiosis in interspecific equine hybrids. I. The male mule (Equus asinus X E. caballus) and hinny (E. caballus X E. asinus). Cytogenetics and Cell Genetics, 13, 330–341.
Chowdhary, B. P., & Raudsepp, T. (2000). Cytogenetics and physical gene maps. In A. T. Bowling and A. Ruvinsky (eds.), The genetics of the horse (pp. 171–242). Wallingford, Oxon: CABI.
Coleman, S. J., Zeng, Z., Wang, K., Luo, S., Khrebtukova, I. et al. (2010). Structural annotation of equine protein-coding genes determined by mRNA sequencing. Animal Genetics, 41(Suppl. 2), 121–130.
Colenbrander, B., Gadella, B. M., & Stout, T. A. (2003). The predictive value of semen analysis in the evaluation of stallion fertility. Reproduction of Domestic Animals, 38, 305–311.
Constant, S. B., Larsen, R. E., Asbury, A. C., Buoen, L. C., & Mayo, M. (1994). XX male syndrome in a cryptorchid stallion. Journal of the American Veterinary Medical Association, 205, 83–85.
Coutinho da Silva, M. A. (2008). When should a mare go for assisted reproduction? Theriogenology, 70, 441–444.
Cuzin, F., & Rassoulzadegan, M. (2010). Non-Mendelian epigenetic heredity: gametic RNAs as epigenetic regulators and transgenerational signals. Essays in Biochemistry, 48, 101–106.
Das, P. J., Lyle, S. K., Beehan, D., Chowdhary, B. P., & Raudsepp, T. (2011a). Cytogenetic and molecular characterization of Y isochromosome in a 63XO/64Xi(Yq) mosaic karyotype of an intersex horse. Sexual Development, 6(1–3), 117–127.
Das, P. J., McCarthy, F., Kachroo, P., Vishnoi, M., Gresham, C. et al. (2011b). Analysis of stallion sperm transcriptome by RNA sequencing. Paper presented at The 9th Dorothy Russell Havemeyer Foundation International Equine Genome Mapping Workshop, July 27–29, Minneapolis, Minnesota.
Das, P. J., McCarthy, F., Vishnoi, M., Li, G., Love, C. C. et al. (2011c). Characterizing stallion sperm transcriptome using RNA-Seq. Paper presented at Plant & Animal Genome XIX, January 15–19, San Diego, CA.
Das, P. J., Paria, N., Gustafson-Seabury, A., Vishnoi, M., Chaki, S. P. et al. (2010a). Total RNA isolation from stallion sperm and testis biopsies. Theriogenology, 74, 1099–1106.
Das, P. J., Vishnoi, M., Kachroo, P., Wang, J., Love, C.C. et al. (2010b). Expression microarray profiling of sperm and testis mRNA of reproductively normal stallions. Animal Reproduction Science, 121S, S175.
de Lorenzi, L., Molteni, L., Zannotti, M., Galli, C., & Parma, P. (2010). X trisomy in a sterile mare. Equine Veterinary Journal, 42, 469–470.
Di Meo, G. P., Neglia, G., Perucatti, A., Genualdo, V., Iannuzzi, A. et al. (2009). Numerical sex chromosome aberrations and abnormal sex development in horse and sheep. Sexual Development, 3, 329–332.
Diribarne, M., Vaiman, A., Péchayre, M., Pailhoux, E., Mata, X. et al. (2009). Polymorphism analysis of microsatellites associated with seven candidate genes for equine cryptorchidism. Journal of Equine Veterinary Science, 29, 37–41.
Ducos, A., Revay, T., Kovacs, A., Hidas, A., Pinton, A. et al. (2008). Cytogenetic screening of livestock populations in Europe: An overview. Cytogenetic & Genome Research, 120, 26–41.
Durkin, K., Raudsepp, T., & Chowdhary, B. P. (2011). Cytogenetic evaluation of the stallion. In A. O. McKinnon, W. E. Vaala & D. D. Varner (eds.), Equine reproduction (pp. 1462–1468). London: Wiley-Blackwell.
Elis, S., Blesbois, E., Couty, I., Balzergue, S., Martin-Magniette, M. L. et al. (2009). Identification of germinal disk region derived genes potentially involved in hen fertility. Molecular Reproduction and Development, 76, 1043–1055.
Farber, C. R., & Lusis, A. J. (2008). Integrating global gene expression analysis and genetics. Advances in Genetics, 60, 571–601.
Ferlin, A., Zuccarello, D., Zuccarello, B., Chirico, M. R., Zanon, G.F. et al. (2008). Genetic alterations associated with cryptorchidism. Journal of the American Medical Association, 300, 2271–2276.
Foresta, C., Zuccarello, D., Garolla, A., & Ferlin, A. (2008). Role of hormones, genes, and environment in human cryptorchidism. Endocrinology Review, 29, 560–580.
Giese, A., Jude, R., Kuiper, H., Piumi, F., Schambony, A. et al. (2002). Molecular characterization of the equine AEG1 locus. Gene, 292, 65–72.
Giesecke, K., Hamann, H., Sieme, H., & Distl, O. (2010). Evaluation of prolactin receptor (PRLR) as candidate gene for male fertility in Hanoverian warmblood horses. Reproduction of Domestic Animals, 45, 214–230.
Giesecke, K., Hamann, H., Sieme, H., & Distl, O. (2010a) INHBA-associated markers as candidates for stallion fertility. Reproduction of Domestic Animals, 45, 342–347.
Giesecke, K., Hamann, H., Stock, K. F., Woehlke, A., Sieme, H. et al. (2009b) Evaluation of SPATA1-associated markers for stallion fertility. Animal Genetics, 40, 359–365.
Giesecke, K., Sieme, H., & Distl, O. (2010b). Infertility and candidate gene markers for fertility in stallions: A review. Veterinary Journal, 185, 265–271.
Gur, Y., & Breitbart, H. (2006). Mammalian sperm translate nuclear-encoded proteins by mitochondrial-type ribosomes. Genes Development, 20, 411–416.
Gur, Y., & Breitbart, H. (2007). Protein translation in mammalian sperm. Society of Reproductive Fertility, 65(Suppl.), 391–397.
Gur, Y., & Breitbart, H. (2008). Protein synthesis in sperm: Dialog between mitochondria and cytoplasm. Molecular and Cell Endocrinology, 282, 45–55.
Hamann, H., Jude, R., Sieme, H., Mertens, U., Topfer-Petersen, E. et al. (2007). A polymorphism within the equine CRISP3 gene is associated with stallion fertility in Hanoverian warmblood horses. Animal Genetics, 38, 259–264.
Harris, R. M., Finlayson, C., Weiss, J., Fisher, L., Hurley, L. et al. (2010). A missense mutation in LRR8 of RXFP2 is associated with cryptorchidism. Mammalian Genome, 21, 442–449.
Hayes, H. M. (1986). Epidemiological features of 5009 cases of equine cryptorchism. Equine Veterinary Journal, 18, 467–471.
Haynes, S. E., & Reisner, A. H. (1982). Cytogenetic and DNA analyses of equine abortion. Cytogenetics and Cell Genetics, 34, 204–214.
Herzog, A., Höhn, H., & Hecht, W. C. (1989). Gonosomenmosaik beim maskulinen Pseudohermaphroditismus des Pferdes. Tierärztliche Praxis, 17, 171–175.
Hunt, P. A., & Hassold, T. J. (2008). Human female meiosis: What makes a good egg go bad? Trends in Genetics, 24, 86–93.
Ing, N. H., Laughlin, A. M., Varner, D. D., Welsh, Jr., T. H., Forrest, D. W. et al. (2004). Gene expression in the spermatogenically inactive “dark” and maturing “light” testicular tissues of the prepubertal colt. Journal of Andrology, 25, 535–544.
Juengel, J. L., Bodensteiner, K. J., Heath, D. A., Hudson, N. L., Moeller, C. L. et al. (2004). Physiology of GDF9 and BMP15 signalling molecules. Animal Reproduction Science, 82–83, 447–460.
Juras, R., Raudsepp, T., Das, P. J., Conant E., & Cothran, E. G. (2010). XX/XY blood lymphocyte chimerism in heterosexual dizygotic American Bashkir Curly horse twins. Journal of Equine Veterinary Science, 30, 575–580.
Kakoi, H., Hirota, K., Gawahara, H., Kurosawa, M., & Kuwajima, M. (2005). Genetic diagnosis of sex chromosome aberrations in horses based on parentage test by microsatellite DNA and analysis of X- and Y-linked markers. Equine Veterinary Journal, 37, 143–147.
King, S. S., Campbell, A. G., Dille, E. A., Roser, J. F., Murphy, L. L. et al. (2005). Dopamine receptors in equine ovarian tissues. Domestic Animal Endocrinology, 28, 405–415.
King, W. A. (2008). Chromosome variation in the embryos of domestic animals. Cytogenetic and Genome Research, 120, 81–90.
King, W. A., Bezard, J., Bousquet, D., Palmer, E., & Betteridge, K. J. (1987). The meiotic stage of preovulatory oocytes in mares. Genome, 29, 679–682.
King, W. A., Desjardins, M. R., Xu, K. P., & Bousquet, D. (1990). Chromosome analysis of horse oocytes cutured in vitro. Genetics and Selective Evolution, 22, 151–160.
Krawetz, S. A. (2005). Paternal contribution: New insights and future challenges. National Review of Genetics, 6, 633–642.
Kubien, E. M., Pozor, M. A., & Tischner, M. (1993). Clinical, cytogenetic and endocrine evaluation of a horse with a 65,XXY karyotype. Equine Veterinary Journal, 25, 333–335.
Laughlin, A. M., Forrest, D. W., Varner, D. D., Blanchard, T. L., Love, C. C. et al. (2002). DNA microarray analysis of gene expression in testicular tissue of stallions. Theriogenology, 58, 413–415.
Lear, T. L., & Bailey, E. (2008). Equine clinical cytogenetics: The past and future. Cytogenetic & Genome Research, 120, 42–49.
Lear, T. L., Lundquist, J., Zent, W. W., Fishback, Jr., W. D., & Clark, A. (2008). Three autosomal chromosome translocations associated with repeated early embryonic loss (REEL) in the domestic horse (Equus caballus). Cytogenetic and Genome Research, 120, 117–122.
Lechniak, D., Wieczorek, M., & Sosnowski, J. (2002). Low incidence of diploidy among equine oocytes matured in vitro. Equine Veterinary Journal, 34, 738–740.
Leeb, T. (2007). The horse genome project – sequence based insights into male reproductive mechanisms. Reproduction of Domestic Animals, 42(Suppl 2), 45–50.
Leeb, T., Sieme, H., & Topfer-Petersen, E. (2005). Genetic markers for stallion fertility – lessons from humans and mice. Animal Reproductive Science, 89, 21–29.
Lopes, A. M., Burgoyne, P. S., Ojarikre, A., Bauer, J., Sargent, C. A. et al. (2010). Transcriptional changes in response to X chromosome dosage in the mouse: Implications for X inactivation and the molecular basis of Turner Syndrome. BMC Genomics, 11, 82.
Madill, S. (2002). Reproductive considerations: Mare and stallion. Veterinary Clinical North American Equine Practice, 18, 591–619.
Makela, O., Gustavsson, I., & Hollmen, T. (1994). A 64,X,i(Xq) karyotype in a standardbred filly. Equine Veterinary Journal, 26, 251–254.
Makinen, A., Hasegawa, T., Makila, M., & Katila, T. (1999). Infertility in two mares with XY and XXX sex chromosomes. Equine Veterinary Journal, 31, 346–349.
Makinen, A., Katila, T., Andersson, M., & Gustavsson, I. (2000). Two sterile stallions with XXY-syndrome. Equine Veterinary Journal, 32, 358–360.
Massip, K., Bonnet, N., Calgaro, A., Billoux, S., Baquie, V. et al. (2009). Male meiotic segregation analyses of peri- and paracentric inversions in the pig species. Cytogenetic and Genome Research, 125, 117–124.
Matzuk, M. M., & Lamb, D. J. (2002). Genetic dissection of mammalian fertility pathways. Nature & Cell Biology, 4(Suppl), S41–S49.
Matzuk, M. M., & Lamb, D. J. (2008). The biology of infertility: Research advances and clinical challenges. Nature & Medicine, 14, 1197–1213.
McCue, M., Mickelson, J., Bannasch, D., Penedo, C., Bailey, E. et al. (2009). The horse gentrain project: initial evaluation of the Equine SNP50 BeadCips. Paper presented at The 8th Dorothy Russell Havemeyer Foundation International Equine Genome Mapping Workshop, July 22–25, Suffolk, UK.
Merkl, M., Otzdorff, C., Herbach, N., Wanke, R., Wolf, E. et al. (2010). Identification of differentially expressed genes in equine endometrium at day 12 of pregnancy. Animal Reproduction Science, 1215, S288–S290.
Meyers-Wallen, V. N., Hurtgen, J., Schlafer, D., Tulleners, E., Cleland, W. R. et al. (1997). Sry-negative XX true hermaphroditism in a Pasa Fino horse. Equine Veterinary Journal, 29, 404–408.
Montgomery, S. B., Sammeth, M., Gutierrez-Arcelus, M., Lach, R. P., Ingle, C. et al. (2010). Transcriptome genetics using second generation sequencing in a Caucasian population. Nature, 464, 773–777.
Naz, R. K., & Catalano, B. (2010). Gene knockouts that affect female fertility: Novel targets for contraception. Frontline Bioscience, 2(Schol ed.), 1092–1112.
Ogorevc, J., Dovc, P., & Kunej, T. (2011). Comparative genomics approach to identify candidate genetic loci for male fertility. Reproduction of Domestic Animals, 46, 229–239.
Ostermeier, G. C., Dix, D. J., Miller, D., Khatri, P., & Krawetz, S. A. (2002). Spermatozoal RNA profiles of normal fertile men. Lancet, 360, 772–777.
Ostermeier, G. C., Goodrich, R. J., Diamond, M. P., Dix, D. J., & Krawetz, S. A. (2005a). Toward using stable spermatozoal RNAs for prognostic assessment of male factor fertility. Fertility and Sterility, 83, 1687–1694.
Ostermeier, G. C., Goodrich, R. J., Moldenhauer, J. S., Diamond, M. P., & Krawetz, S. A. (2005b). A suite of novel human spermatozoal RNAs. Journal of Andrology, 26, 70–74.
Paget, S., Ducos, A., Mignotte, F., Raymond, I., Pinton, A. et al. (2001). 63,XO/65,XYY mosaicism in a case of equine male pseudohermaphroditism. Veterinary Record, 148, 24–25.
Paria, N. (2009). Discovery of candidate genes for stallion fertility. College Station: Texas A&M University, College of Veterinary Medicine.
Paris, D. B. B. P., Kuijk, E. W., Roelen, B. A. J., & Stout, T. A. E. (2010). Stage specific changes in expression of OCT4, CDX2, NANOC and GATA6 mRNA during blastocyst formation in the horse. Animal Reproduction Science, 1215, S291–S292.
Pinton, A., Calgaro, A., Bonnet, N., Ferchaud, S., Billoux, S. et al. (2009). Influence of sex on the meiotic segregation of a t(13;17) Robertsonian translocation: A case study in the pig. Human Reproduction, 24, 2034–2043.
Pinton, A., Ducos, A., & Yerle, M. (2004). Estimation of the proportion of genetically unbalanced spermatozoa in the semen of boars carrying chromosomal rearrangements using FISH on sperm nuclei. Genetics, Selection, Evolution, 36, 123–137.
Pinton, A., Raymond Letron, I., Berland, H. M., Bonnet, N., Calgaro, A. et al. (2008). Meiotic studies in an azoospermic boar carrying a Y;14 translocation. Cytogenetic and Genome Research, 120, 106–111.
Piprek, R. P. (2009). Genetic mechanisms underlying male sex determination in mammals. Journal of Applied Genetics, 50, 347–360.
Platts, A. E., Dix, D. J., Chemes, H. E., Thompson, K. E., Goodrich, R. et al. (2007). Success and failure in human spermatogenesis as revealed by teratozoospermic RNAs. Human Molecular Genetics, 16, 763–773.
Power, M. M., Gustavsson, I., Switonski, M., & Ploen, L. (1992). Synaptonemal complex analysis of an autosomal trisomy in the horse. Cytogenetics and Cell Genetics, 61, 202–207.
Qu, Z., Chowdhary, B. P., Raudsepp, T., and Adelson, D. L. (2011). Design of an equine whole genome tiling array. Paper presented at Plant & Animal Genome XIX, January 15–19, San Diego, CA.
Rambags, B. P., Krijtenburg, P. J., Drie, H. F., Lazzari, G., Galli, C. et al. (2005). Numerical chromosomal abnormalities in equine embryos produced in vivo and in vitro. Molecular Reproduction and Development, 72, 77–87.
Raudsepp, T., Durkin, K., Lear, T., Das, P. J., Avila, F. et al. (2010). Molecular heterogeneity of XY sex reversal in the horse. Animal Genetics Special Issue on Horse Genetics and Genomics.
Raudsepp, T., McCue, M. E., Das, P. J., Vishnoi, M., Fritz, K. L. et al. (2011). Genome-wide association studies and gene expression profiling in Thoroughbred stallions with acrosomal dysfunction. Paper presented at Plant & Animal Genome XIX, January 15–19, San Diego, CA.
Romagnano, A., Richer, C. L., King, W. A., & Betteridge, K. J. (1987). Analysis of X-chromosome inactivation in horse embryos. Journal of Reproductive Fertility, 35(Suppl.), 353–361.
Rothfels, K. H., Alexrad, A. A., Siminovitch, L., & McCulloch Parker, R. C. (1959). The origin of altered cell lines from mouse, monkey and man, as indicated by chromosome and transplantation studies. Proceedings of Canadian Cancer Research Conference, 3, 189–214.
Rubes, J., Vozdova, M., & Kubickova, S. (1999). Aneuploidy in pig sperm: Multicolor fluorescence in situ hybridization using probes for chromosomes 1, 10, and Y. Cytogenetics and Cell Genetics, 85, 200–204.
Sabeur, K., Ball, B. A., Corbin, C. J., & Conley, A. (2008). Characterization of a novel, testis-specific equine serine/threonine kinase. Molecular Reproduction and Development, 75, 867–873.
Safronova, L. D., & Pimentova, T. I. (1988). The karyotyping of bull (Bos taurus) and of horse (Equus caballus) on the basis of the synaptonemal complexes. Genetika, 24, 708–714.
Scott, I. S., & Long, S. E. (1980). An examination of chromosomes in the stallion (Equus caballus) during meiosis. Cytogenetics and Cell Genetics, 26, 7–13.
Shendure, J. (2008). The beginning of the end for microarrays? Natural Methods, 5, 585–587.
Short, R. V., Chandley, A. C., Jones, R. C., & Allen, W. R. (1974). Meiosis in interspecific equine hybrids. II. The przewalski horse/domestic horse hybrid. Cytogenetics and Cell Genetics, 13, 465–478.
Sudderth, A. K., Das, P. J., Varner, D. D., & Raudsepp, T. (2010). Determination of optimal semen processing methods for total RNA isolation and sperm genomic analysis. Animal Reproduction Science, 121(Suppl.), S149–S150.
Templado, C., Donate, A., Giraldo, J., Bosch, M., & Estop, A. (2011). Advanced age increases chromosome structural abnormalities in human spermatozoa. European Journal of Human Genetics, 19, 145–151.
Turner, R. M., & Casas-Dolz, R. (2002). Differential gene expression in stallions with idiopathic testicular degeneration. Theriogenology, 58, 421–434.
Varner, D. D. (2008). Developments in stallion semen evaluation. Theriogenology, 70, 448–462.
Varner, D. D., & Johnson, L. (2007). From a Sperm’s eye view – revisiting our perception of this intriguing cell. AAEP Proceedings, 53, 104–177.
Vaughan, L., Schofield, W., & Ennis, S. (2001). SRY-negative XX sex reversal in a pony: A case report. Theriogenology, 55, 1051–1057.
Villagomez, D. A., Parma, P., Radi, O., Di Meo, G., Pinton, A. et al. (2009). Classical and molecular cytogenetics of disorders of sex development in domestic animals. Cytogenetic and Genome Research, 126, 110–131.
Villagomez, D. A., & Pinton, A. (2008). Chromosomal abnormalities, meiotic behavior and fertility in domestic animals. Cytogenetic and Genome Research, 120, 69–80.
von Nett, P., Jung, H. R., & Stranzinger, G. (1996). Neue Erkenntnisse über die Chromosomenpaarung in der Meiose. Deutsche Tierärztl Wschr, 103, 369–448.
Wade, C. M., Giulotto, E., Sigurdsson, S., Zoli, M., Gnerre, S. et al. (2009). Genome sequence, comparative analysis, and population genetics of the domestic horse. Science, 326, 865–867.
Wang, Z., Gerstein, M., & Snyder, M. (2009). RNA-Seq: A revolutionary tool for transcriptomics. National Review of Genetics, 10, 57–63.
Watson, E. D., Bae, S. E., Steele, M., Thomassen, R., Pedersen, H. G. et al. (2004). Expression of messenger ribonucleic acid encoding for steroidogenic acute regulatory protein and enzymes, and luteinizing hormone receptor during the spring transitional season in equine follicles. Domestic Animal Endocrinology, 26, 215–230.
Watson, E. D., Thomson, S. R., & Howie, A. F. (2000). Detection of steroidogenic acute regulatory protein in equine ovaries. Journal of Reproduction & Fertility, 119, 187–192.
Wilhelm, D., Palmer, S., & Koopman, P. (2007). Sex determination and gonadal development in mammals. Physiology Review, 87, 1–28.
Woods, J., Rigby, S., Brinsko, S., Stephens, R., Varner, D. et al. (2000). Effect of intrauterine treatment with prostaglandin E2 prior to insemination of mares in the uterine horn or body. Theriogenology, 53, 1827–1836.
Zenteno-Ruiz, J. C., Kofman-Alfaro, S., & Mendez, J. P. (2001). 46,XX sex reversal. Archives of Medical Research, 32, 559–566.
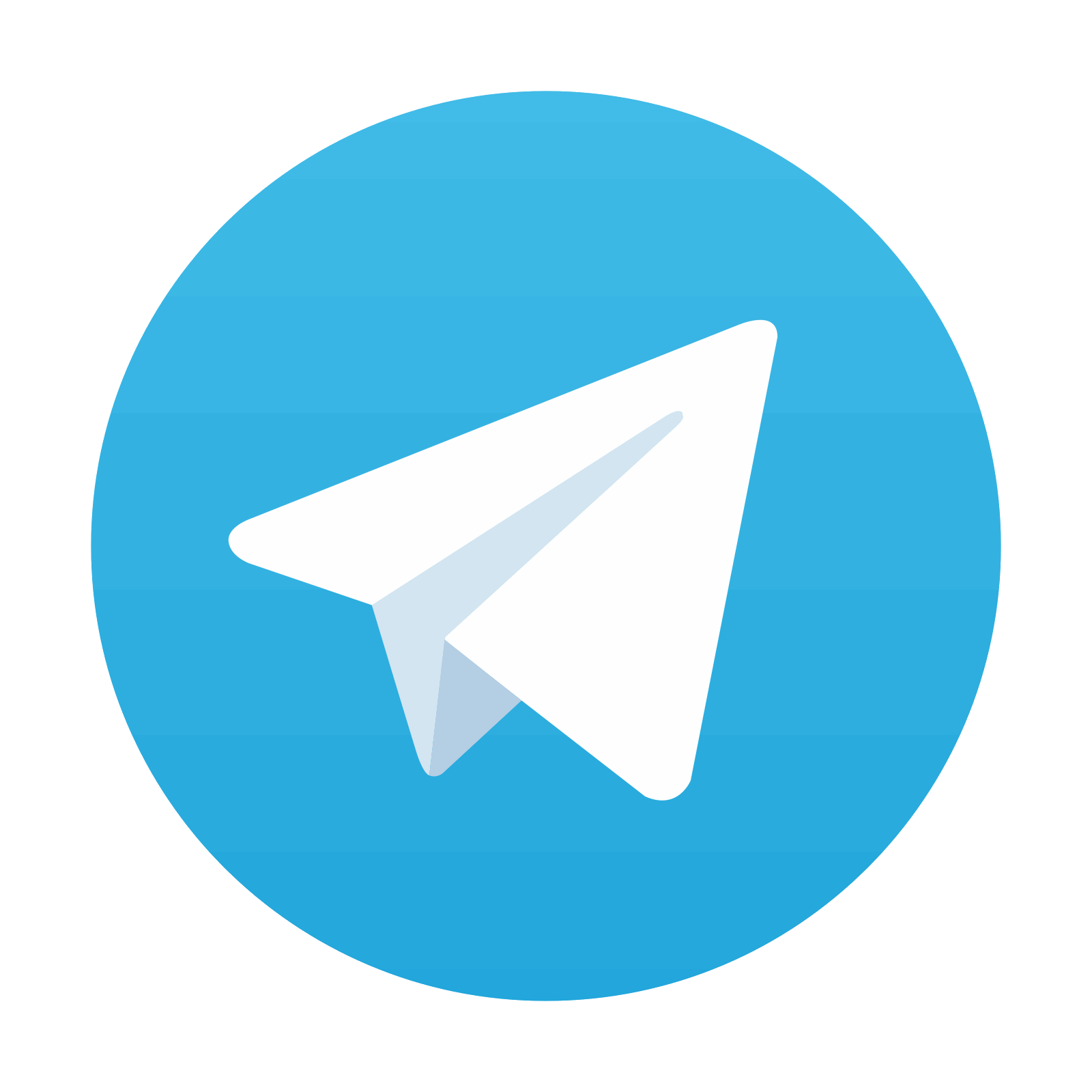
Stay updated, free articles. Join our Telegram channel

Full access? Get Clinical Tree
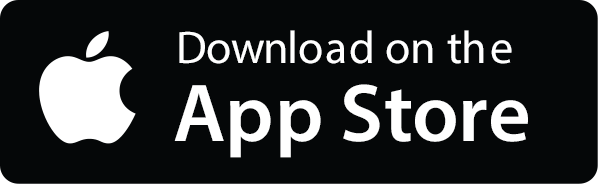
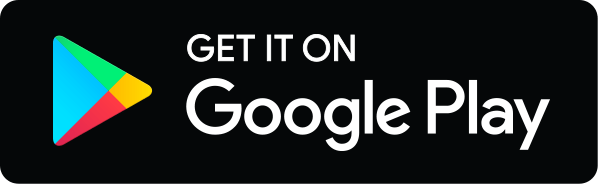