Chapter 2. Genetic Basis of Cancer
Jaime F. Modiano and Matthew Breen
It is now clear that cancer is a genetic disease. 1,2 Since the discovery and characterization of the Philadelphia chromosome as the first non-random translocation in cancer in the 1960s and 1970s, several hundred genetic abnormalities that are peculiarly associated with specific cancers have been identified. Rather than provide an exhaustive list of genes, here we will focus on contemporary issues that highlight the significance of cancer genetics in diagnosis and treatment, and how interactions between genes and environment impact the origin, progression, and response to therapy of most tumors.
GENES AND CANCER RISK
To understand the implications of cancer, one must first realize that cancer is not a simple disease. Rather, the term cancer describes a large number of diseases whose only common feature is uncontrolled cell growth and proliferation. An important concept that is universally accepted is that cancer is a genetic disease, although it is not always heritable. Tumors arise from the accumulation of mutations that eliminate normal constraints of proliferation and genetic integrity in a somatic cell. Among other causes, mutations can arise because of the inherent error rate of enzymes that control DNA replication during each division, which introduces about 1 in 1,000,000 to 1 in 10,000,000 mutations for each base that is replicated during each round of cell division. The genome consists of many millions of base pairs, so each daughter cell is likely to carry at least a few mutations in its DNA. Most of these mutations are silent; that is, they do not present any problems to the cell’s ability to function. However, others can disable tumor suppressor genes or activate proto-oncogenes that respectively inhibit or promote cell division and survival. Hence, it can be said that “being alive” is the single largest risk factor for cancer.
However, there is evidence to suggest that in some cases mutations are “directed” because of the presence of a “mutator phenotype,” where the factors that control DNA replication and repair are prone to more errors than would be expected by simple random events. This leads to different rates of cancer predisposition, which would be higher than the mean in individuals bearing this “mutator phenotype,” and might explain why not all people or animals exposed to similar environmental carcinogens develop the same forms of cancer at the same rate.
This genetic predisposition to cancer is illustrated by the existence of well-defined heritable cancer syndromes. 3 Even though they account for fewer than 5% of all human cancers, studies of families with these syndromes provided many of the initial clues to understand the genetic basis of sporadic (non-heritable) cancers. In most cases, these familial cancer syndromes show dominant patterns of inheritance and have high penetrance, although the mechanism of disease is not due to inheritance of a dominant allele, but rather is generally due to inheritance of a mutant (inactive) allele followed by inactivation of the second allele through a process called loss of heterozygosity . A curious observation is worth noting: different mutations in a single gene predispose individuals to distinct cancer syndromes, whereas independent, single mutations of different genes can result in virtually the same disease, or at least diseases with indistinguishable phenotypes. 3 This is less surprising when we consider the fact that commonly affected genes are multifunctional and are parts of complex interactive networks or circuits, 4 so a mutation may only alter gene function along one biochemical pathway, leaving its interactions with other pathways intact. Another intriguing observation is that mutations that contribute to most sporadic cancers are restricted to a small subset of genes, 5,6 many of which are also associated with heritable cancer syndromes. These observations have given rise to competing contemporary theories on the origins of cancer, which are addressed in Table 2-1 .
Property | Somatic Cell Theory | Stem Cell Theory |
---|---|---|
Origin | Any somatic cell that acquires the right complement of mutations | Stem cell with a critical transforming mutation or de-differentiated somatic cell with stem cell properties |
Self-renewal | Acquired through mutation | Inherent property of stem cells |
Genes involved | Does not predict recurrent gene involvement for different cancers—any gene that controls growth, survival, or immortalization of the affected cell can contribute | Predicts that a small number of genes that specifically regulate stem cell self-renewal will disproportionately contribute to all types of cancer |
Differentiation | Cells arrest development at a particular stage or undergo limited de-differentiation caused by mutation | Inherent property of stem cell progeny |
Metastatic potential | Acquired through mutation | Inherent property of stem cells, but also can be acquired through mutation |
Resistance to cytotoxic therapy | Acquired through mutation or selection | Inherent property of stem cells |
At least one heritable cancer syndrome (renal carcinoma and nodular dermatofibrosis [RCND] of German Shepherd dogs) has been described in dogs. 7 The heritable factor (or RCND gene) for this syndrome maps to dog chromosome 5 (CFA 5), and specifically to the folliculin gene, which was recently described as the heritable factor for the corresponding human disease (Birt-Hogg-Dube syndrome). It is probable that other syndromes comparable to those described in humans eventually will be identified in companion and laboratory animals, but it is unlikely these will account for more than 5% to 10% of all cancer cases. Yet, even with the estimated lifetime cancer risk (for humans and for dogs) of approximately 1 in 3, it is incorrect to surmise that most cancers (>90%) are due solely to environmental causes. Heritable influences affect susceptibility, but they are difficult to identify in heterogeneous outbred backgrounds such as those that occur in most human families or mixed breed dogs. On the other hand, highly inbred human populations and families, specific ethnic groups, or purebred dogs may allow for identification of factors that influence both cancer risk and phenotype. 8-10 Outside of these populations, the existence of heritable factors that are modulated by environmental influence will be labor intensive to define. For example, more than 3000 matched cases and controls would be needed to unequivocally identify the multiplicative interaction among two or more genes that increase cancer risk by two-fold when the allele frequency is 5%, and more than 1500 would still be required when the allele frequency is as high as 25%. 11
Changes in cancer incidence over the course of the 20th century, many reflecting behavior patterns (e.g., lung cancer in smokers), infectious diseases (e.g., stomach cancer in people infected with Helicobacter pylori ), or exposure to special cultural factors such as urbanization or diet (e.g., increasing breast cancer rates in the second and subsequent generations of Asian-American women), underscore the significant influence that the environment exerts on the genetic make-up of any individual. We anticipate that the combination of novel molecular and epidemiologic approaches, combined with the judicious use of laboratory animal models and naturally occurring cancers of humans and companion animals, will allow us to tease apart the relative contributions of genes and environment to the process of carcinogenesis in the near future.
IS CANCER A DISEASE OF STEM CELLS?
Unlike diseases that arise from single gene defects, cancer is a complex, multigenic disease. In fact, a sequential progression of mutations is required to produce a bona fide tumor cell. In the classical model of initiation, promotion, and progression, a genetic mutation first endows a somatic cell with limitless replicative potential or another growth or survival advantage from other cells in its environment. Alone, this mutation is not sufficient to give rise to a tumor, as the cell remains constrained by environmental factors. A second mutation (or series of mutations) further adds to the cell’s ability to outcompete its neighbors in this environment, leading to its potential expansion into a recognizable tumor mass. Finally, a third series of mutations reinforces the cell’s malignant potential (invasion, tissue destruction, and metastasis) that leads to clinical disease (see Table 2-1 ).
This model is useful to illustrate the point that no single gene is universally responsible for transformation, but rather, we can think of many genes contributing to (rather than “causing”) the origin and progression cancer. 1 It also provides a basis to set a minimum estimate of five or six mutations that are required before cancer becomes clinically evident, although empirical evidence suggests the actual number required is higher. 6 These mutations provide a cell and its progeny with each of the six hallmarks of cancer, 12 namely (1) self-sufficiency of growth signals, (2) insensitivity to anti-growth signals, (3) the ability to evade apoptosis, (4) limitless replicative potential, (5) sustained angiogenesis, and (6) the capacity to invade tissues and metastasize ( Table 2-2 ). In some instances, mutation of a single gene may provide more than one such property, but more often, mutations of multiple genes are necessary to achieve each of these “milestones.” Moreover, since interactive biochemical cascades regulate each of these six steps, mutations of different genes along shared pathways can result in equivalent phenotypes (see http://www.nature.com/nrc/journal/v2/n5/weinberg_poster/ ). Conceptually, this helps us understand how mutations of different genes can lead to similar cancer phenotypes and outcome, and conversely why mutations of the same gene can result in different cancers with distinct biological behaviors.
< div class='tao-gold-member'>
Only gold members can continue reading. Log In or Register a > to continue
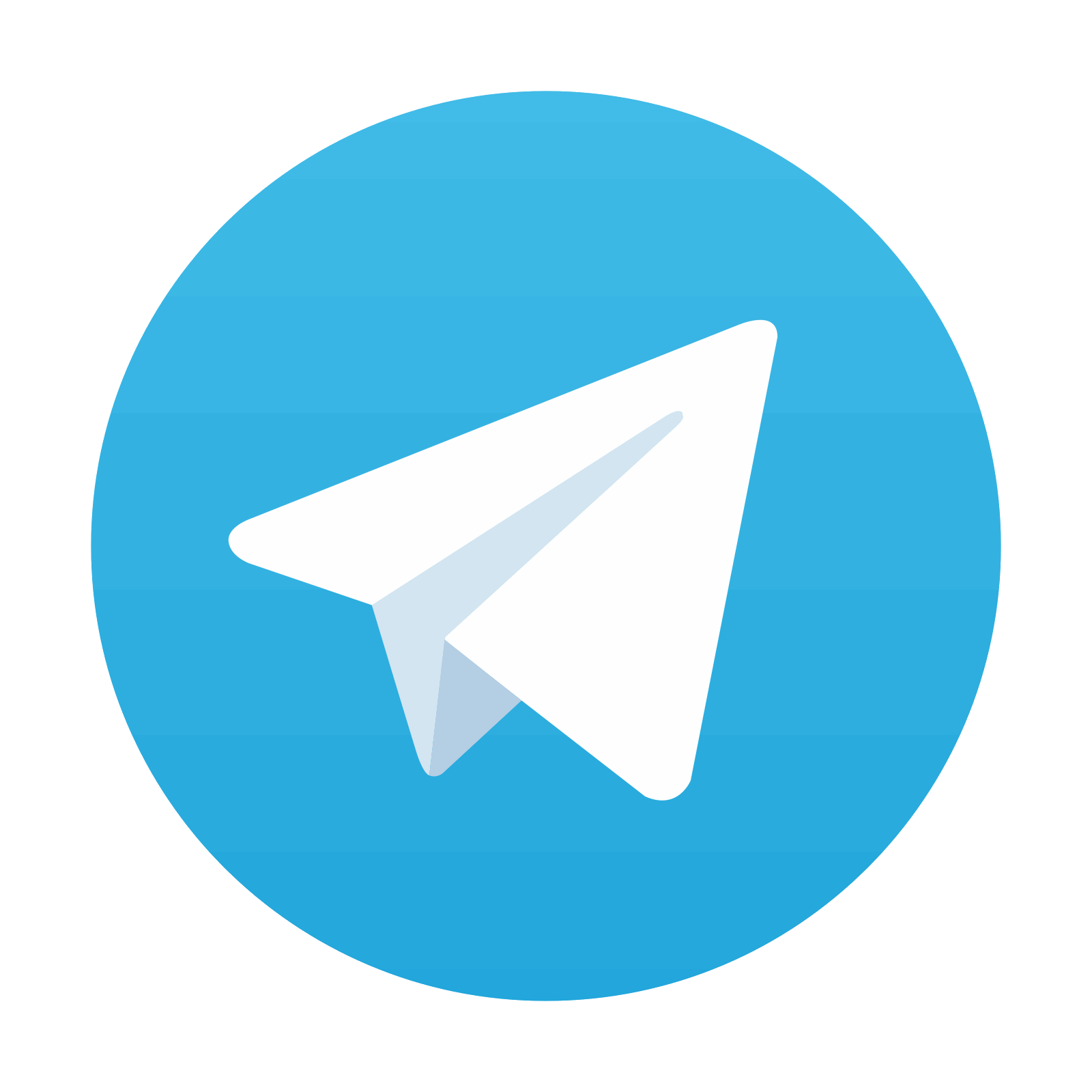
Stay updated, free articles. Join our Telegram channel

Full access? Get Clinical Tree
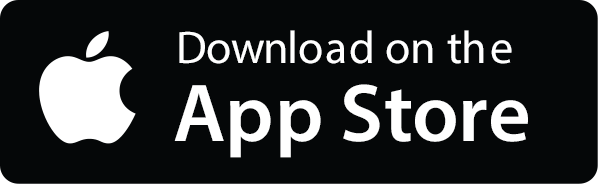
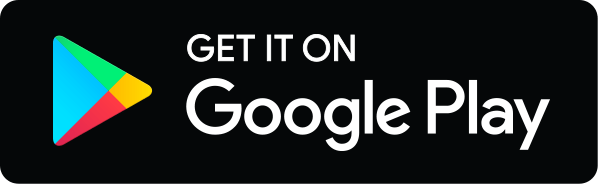