Chapter 1 General Toxicological Principles
Toxicology is the study of poisons and their effects on living organisms. In veterinary medicine, this has come to mean an understanding of sources of poisons, circumstances of exposure, diagnosis of the type of poisoning, treatment, and application of management or educational strategies to prevent poisoning.1–3 More so than many of the specialties in veterinary medicine, toxicology is based on the important principle of dose and response. That is, there is a graded and possibly predictable response based on increasing exposure to the toxicant. In the words of Philipus Aureolus Theophrastus Bombastus von Hohenheim-Paracelsus, a physician-alchemist of the sixteenth century, “All substances are poisons; there is none which is not a poison. The right dose differentiates a poison from a remedy.”2 Although alchemy has long since been abandoned, Paracelsus’ principle of what makes a poison is still true and relevant in the daily practice of nutrition, therapeutics, and toxicology. Today, with emphasis on synthetic drugs, natural or alternative therapies, and the rapidly growing field of nutraceuticals, there is increasing need to be aware of the dosage and response principle for both beneficial and detrimental effects in the daily practice of veterinary medicine. Many of the toxicants discussed in this book will provide examples of the point at which the dosage determines whether the agent is a nutrient, a remedy, or a poison.
Determinants of exposure that affect dosage may be more than simply the gross amount of material ingested or applied to the skin. Rather, the effective dosage at a susceptible receptor site determines the ultimate response. Thus species differences in metabolism, vehicle differences that promote skin penetration, specific drug or chemical interactions that potentiate response, and organ dysfunction that limits elimination can all influence the ultimate dosage.2–4 Clinicians must consider all of these possibilities when working to diagnose a potential toxicosis or apply therapeutic agents to their patients.
Toxicology literature is best understood if some basic terminology is remembered. A poison or toxicant is usually considered any solid, liquid, or gas that when introduced into or applied to the body can interfere with homeostasis of the organism or life processes of its cells by its own inherent qualities, without acting mechanically and irrespective of temperature. The term toxin is used to describe poisons that originate from biological sources and are generally classified as biotoxins. Biotoxins are further classified according to origin as zootoxins (animal origin), bacterial toxins (which include endotoxins and exotoxins), phytotoxins (plant origin), and mycotoxins (fungal origin).2–4
The terms toxic, toxicity, and toxicosis are often misunderstood or misused.3,4 The word toxic is used to describe the effects of a toxicant (e.g., the “toxic” effects of organophosphate insecticides may be described as cholinesterase inhibition; vomiting, salivation, dyspnea, and diarrhea). However, toxicity is used to describe the quantitative amount or dosage of a poison that will produce a defined effect. For example, the acute lethal dosage to cats of ethylene glycol would be described as 2 to 5 mL/kg body weight. The toxic effects of ethylene glycol are acidosis and oxalate nephrosis. Finally the state of being poisoned by a toxicant, such as ethylene glycol, is toxicosis.
Mammalian and other vertebrate toxicities are usually expressed as the amount of toxicant per unit of body weight required to produce toxicosis. Dosage is the correct terminology for toxicity expressed as amount of toxicant per unit of body weight.2–4 The commonly accepted dosage units for veterinary medicine are milligrams per kilogram (mg/kg) body weight. However, toxicity can also be expressed as moles or micromoles of agent per kilogram body weight. In some experimental studies, comparisons of large and small animals relate dosage to the body surface area, which is approximately equal to body weight.2–4 The use of body surface area dosages is advocated by some as a more accurate way to account for very different body sizes in veterinary medicine. For clinical toxicology, the examples in Table 1-1 generally show that as animals increase in weight, the body surface area increases proportionally less, and this may affect the rate of metabolism, excretion, and receptor interaction with toxicants.3
Table 1-1 Comparison of Body Weight to Surface Area for Animals of Representative Sizes
Body Weight (kg) | Body Surface (m2) |
---|---|
0.5 | 0.06 |
1.0 | 0.10 |
5.0 | 0.29 |
10.0 | 0.46 |
20.0 | 0.74 |
40.0 | 1.17 |
For many toxicants, larger animals can be poisoned by relatively lower body weight dosages than can smaller mammals.4 However, other factors, such as species differences in metabolism or excretion or specific differences in receptor sites can alter this generalization. Dose is a term for the total amount of a drug or toxicant given to an individual organism. In veterinary medicine, the extreme ranges of body weight and surface area, even within some species, generally make the “dose” approach of little practical value.
A commonly used means to compare the toxicity of compounds with one another is the median lethal dosage, also known as the acute oral LD50 in a standard animal, such as the laboratory rat. The LD50 value is usually based on the effects of a single oral exposure with observation for several days after the chemical is administered to determine an end point for total deaths. The LD50 is a standardized toxicity test that depends on a quantal (i.e., all-or-none) response to a range of regularly increasing dosages. In some cases a multiple-dosage LD50 is used to show the acute effects (typically up to 7 days) produced by multiple dosages in the same animals. Increasing dosage levels are usually spaced at logarithmic or geometric intervals. When cumulative deaths are plotted on linear graph paper, the dose-response curve is sigmoid, and the most predictable value is usually around either side of the LD50 (Figure 1-1).
The end point of an LD50 study is death, and the published LD50 value says nothing about the severity of clinical signs observed in the surviving animals or the nature of the clinical effects.2–4 Twenty or more animals may be used to arrive at a good estimate of the LD50, which limits the use of LD50 values in most animals of economic significance. In some species, such as birds and fish, the oral toxicity is often expressed on the basis of the concentration of the substance in the feed or water. The acute oral toxicity for birds is often expressed as the LC50, meaning the milligrams of compound per kilogram of feed. For fish, the LC50 refers to the concentration of toxicant in the water.
Duration of exposure can greatly affect the toxicity. The single-dose LD50 of warfarin in dogs is approximately 50 mg/kg, whereas 5 mg/kg for 5 to 15 days may be lethal. In rats the single-dose LD50 of warfarin is 1.6 mg/kg, whereas the 90-day LD50 is only 0.077 mg/kg. On the other hand, rapidly inactivated or excreted compounds may have almost the same 90-day LD50 as the single dose LD50. For example, the single-dose LD50 for caffeine in rats is 192 mg/kg and the 90-day LD50 is slightly lower at 150 mg/kg. Conversely, animals may develop tolerance for a compound such that repeated exposure serves to increase the size of the dose required to produce lethality. The single-dose LD50 of potassium cyanide in rats is 10 mg/kg, whereas rats given potassium cyanide for 90 days are able to tolerate a dosage of 250 mg/kg without mortality. The ratio of the acute to chronic LD50 dosage is called the chronicity factor.3 Compounds that have strong cumulative effects have larger chronicity factors. In the foregoing examples the chronicity factors are as follows: warfarin, 20; caffeine, 1.3; and potassium cyanide, 0.04.
From a public health and diagnostic toxicology perspective, it is essential to know the exposure level that will not cause any adverse health effect. This level is usually referred to as the no observed adverse effect level (NOAEL).2 It can also be thought of as the maximum nontoxic level. This is the amount that can be ingested without any deaths, illness, or pathophysiological alterations occurring in animals fed the toxicant for the stated period of time. Usually a NOAEL in laboratory animals is based on chronic exposures ranging from 90 days to 2 or more years, depending on the species. The no-effect level is the largest dosage that does not result in detrimental effects.
The concept of risk or hazard is important to clinical toxicology. Although toxicity defines the amount of a toxicant that produces specific effects at a known dosage, hazard or risk is the probability of poisoning under the conditions of expected exposure or usage. Compounds of high toxicity may still present low hazard or risk if animals are never exposed to the toxicant. For example, ethylene glycol antifreeze would be defined as low toxicity (2 to 5 mL/kg body weight), but because it is often readily available in homes, is voluntarily consumed by cats, and is difficult to reverse once clinical signs have developed, it is seen as a high-risk or high-hazard toxicant. Another way to define risk is to compare the ratio of the lowest toxic or lethal dosage (e.g., the LD1) with the highest effective dosage, which could be defined as the ED99. The ratio of LD1/ED99 is defined as the standard safety margin, and it is useful for comparing the relative risk of therapeutic drugs, insecticides, anthelmintics, and other agents applied to animals for their beneficial effects.2,4
Even more variability is expected because of the differences in species, age, body size, route of exposure, inherent differences in metabolism, and pregnancy and lactation effects. Remember also that the slope of the LD50 curve is important and is not revealed from the LD50 value alone. An LD50 with a very steep dose-response slope indicates a toxicant or drug has a very narrow margin between no effects and maximal lethal effects.3,4 Although such compounds may be dangerous to use as therapeutics, they could be very effective pesticides because of lower probability of survival of target animals.
FACTORS THAT INFLUENCE TOXICITY
Many factors inherent in the toxicant, the animal, or the environment can alter a toxicity value determined under defined experimental conditions. The toxicity of a compound will vary with the route of exposure. Usual routes of exposure are oral, dermal, inhalation, intravenous, intraperitoneal, and subcutaneous. In addition, the most potent routes of exposure are usually the intravenous, intrapulmonary, and intraperitoneal routes. In clinical veterinary toxicology, oral and dermal routes of exposure are the most common, and these routes generally delay the absorption and diffuse exposure over a longer period of time. A daily dosage of toxicant mixed in food and consumed over a 24-hour period may cause much less effect than that same dosage given as a bolus at one specific time. However, retention in the gastrointestinal tract, including enterohepatic cycling, and dermal or hair retention of poisons can significantly prolong the exposure or exposures.2–4 Another factor that can accentuate the toxic effects of a compound is concurrent organ damage as a result of other causes. This is most important for diseases that alter liver or kidney function, leaving the animal with insufficient resources to metabolize and excrete toxicants.
Species and breed differences exert important influences on toxicity. The familiar example of cats and their intolerance to phenolic compounds results directly from their lack of glucuronyl transferase, which is necessary to produce glucuronides for the excretion of phenolic metabolites. A common example is acetaminophen, which is quite toxic to cats partly as a result of ineffective excretion of the toxic metabolite. In addition, the amino acid and sulfhydryl content of feline hemoglobin and a relative lack of methemoglobin reductase in erythrocytes makes it more susceptible to oxidant damage. As a result, the cat is more likely to be poisoned by agents that induce methemoglobinemia.4 Occasional differences within a species can increase the probability of toxicosis. The anthelmintic ivermectin provides an example of breed susceptibility differences, with collies and individuals in other herding breeds being more susceptible.
Many environmental and physiological factors can influence the toxicity of compounds, and one should remember that such factors, or others possibly unknown, can substantially influence an individual’s response to toxicants. Entire publications are devoted to drug and chemical interactions, and the reader is encouraged to be aware of toxicological interactions that are illustrated throughout this text. Some examples of factors that alter response to toxicants are presented in Table 1-2.
Table 1-2 Factors that May Alter Response to Toxicants
Alteration or Change | Mechanism or Example |
---|---|
Impurities or contaminants | Some older phenoxy herbicides were contaminated with a highly toxic dioxin byproduct of manufacturing, leading to chronic toxicosis from the dioxin. |
Changes in chemical composition or salts of inorganic agents | Toxicity of metals may be altered by valence state. Trivalent arsenicals are much more toxic than pentavalent arsenic. Specific salts also alter toxicity (e.g., barium carbonate is cardiotoxic, whereas barium sulfate is insoluble and nearly nontoxic). |
Instability or decomposition of chemical | Some organophosphate insecticides under adverse storage conditions can decompose to form more toxic degradation products. |
Ionization | Generally, compounds that are highly ionized are poorly absorbed and thus less toxic. |
Vehicle effects | Nonpolar and lipid-soluble vehicles usually increase toxicity of toxicants by promoting absorption and membrane penetration. |
Protein binding | Binding to serum albumin is common for many drugs and toxicants, limiting the bioavailability of the agent and reducing toxicity. |
Chemical or drug interactions | Chemicals may directly bind, inactivate, or potentiate one another. One chemical may also induce microsomal enzymes to influence the metabolism of another. |
Biotransformation | Prior exposure to the same or similar chemical may induce increased metabolic activity of microsomal mixed function oxidases (MFOs). Foreign compounds activated by MFOs can then be conjugated by phase II metabolism and excreted. If toxicants are activated by MFO activity, toxicity may be increased. Liver disease, very young or very old animals, and specific breeds or strains of animal can alter ability of MFO to begin metabolism followed by phase II detoxification of foreign compounds. |
Alteration or Change | Mechanism or Example |
---|---|
Liver disease | Reduced synthesis of glutathione, metallothioneine, and coagulation factors may alter response to acetaminophen, cadmium, and anticoagulant rodenticides, respectively. |
Nutrition and diet | Natural dietary compounds, such as calcium and zinc, may affect absorption and response to lead. Vitamin C and vitamin E can aid in scavenging of free radicals and repair of cellular protective mechanisms. |
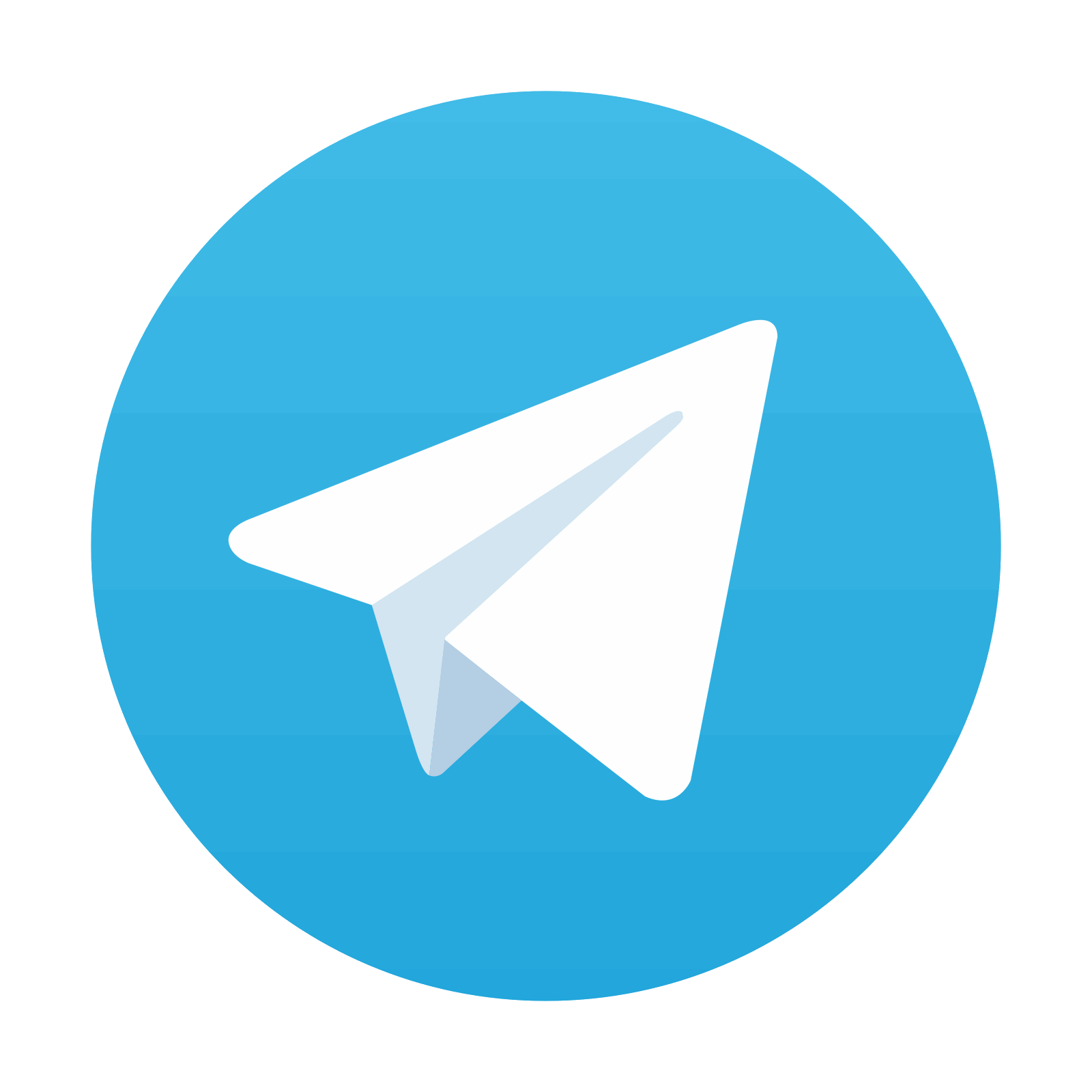
Stay updated, free articles. Join our Telegram channel

Full access? Get Clinical Tree
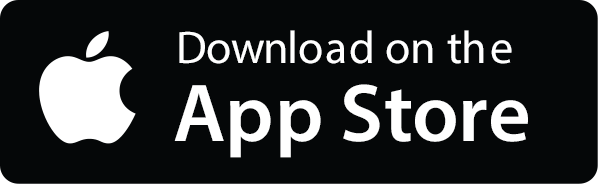
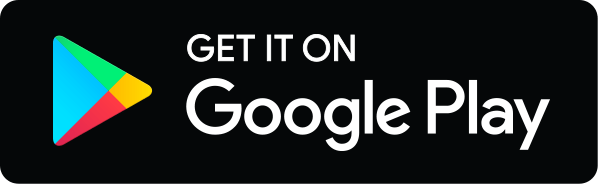