3.1 Background and development
The gastrointestinal system extends from the oral cavity to the anus and includes the intestines and associated glandular organs. The intestines, liver and spleen arise from the primitive endoderm. The oesophagus, cranial duodenum, stomach and spleen develop from foregut endoderm; liver, gall bladder and pancreas from foregut and midgut junctional endoderm; caudal duodenum, jejunum, ileum, caecum and proximal colon from the midgut endoderm and caudal colon and rectum from the hindgut endoderm (Kaufman and Bard 1999).
Teeth develop from oral ectoderm, which leads to the formation of the epithelial components, such as ameloblasts and the neural crest derived mesenchyme, which results in the formation of odontoblasts and cementoblasts.
The pancreas develops in the embryo from endoderm by the formation of two buds from the ventral and dorsal duodenum. The ventral bud forms the cranial part of the head of the pancreas and the remainder of the pancreas originates from the dorsal bud (Slack 1995). The buds develop into highly branched structures to form the mature pancreas. The exocrine and endocrine cells both appear to arise from endoderm and differentiate during bud development. The endocrine cells are initially individual cells associated with ducts and only form aggregates or islets from day 18.5 of gestation.
3.2 Oral cavity
3.2.1 Sampling teeth
Teeth are embedded in the bones of the skull and, as both tissues are calcified, they require decalcification before paraffin-embedded sections can be made or need to be embedded in resin if they are not to be decalcified (Hand 2012; Sterchi 2012). Prior to processing, with or without decalcification, it is important that hard tissues are fixed adequately in formalin for at least 24 to 48 hours. It may be convenient to separate the mandible from the maxilla of the jaw before fixation, but further trimming—producing hemisections of the mandible and maxilla to allow for longitudinal sections—may be best left until the tissue has been softened by decalcification.
For routine examinations teeth are usually decalcified using formic acid or ethylenediaminetetraacetic acid (EDTA)-based techniques. Gooding and Stewart’s (10% formic acid and 5% formaldehyde) or Kristesons’ declacification fluids have been used for mouse teeth (Mohamed and Atkinson 1983). The end point of decalcification can be tested by a chemical test of the decalcification fluid or by trial incision of the edge of the skull. Decalcification using acid-based techniques may be complete in 2–6 days whereas immersion in EDTA may take a minimum of 2–3 weeks (Seidel et al. 2010) before tissues can be trimmed to allow sections can be made. EDTA decalcification is generally preferred if further techniques such as in situ hybridization or immunohistochemistry are required.
3.2.2 Anatomy and histology of teeth
Rodent incisors first erupt at days 10–12 and thereafter continue to erupt throughout life growing at a rate of 1 mm/week (Moinichen et al. 1996). Rodent incisors therefore have active ameloblast and odondoblast cell layers, which can be seen histologically. Ameloblasts are columnar epithelial cells that line the tooth root in a single layer with basally located nuclei (Figure 3.1). The function of the ameloblasts and the function and nature of their surrounding cell populations changes from the base or secretory region to the maturation region (Josephsen et al. 2010). In the secretory region, the ameloblasts are in contact with a layer of flat-to-cuboidal cytokeratin-positive cells, one to four cells thick, the stratum intermedium, which is surrounded by the stellate reticulum and the outer enamel epithelium. The cells of the stellate reticulum are irregular and star shaped with large intercellular spaces. In the maturation zone the ameloblasts are embedded in the papillary layer of irregularly shaped cells, which also contain a rich capillary network. Ameloblasts produce enamel on the labial surface of the incisors throughout life and on all surfaces of the molars during their growth phase, but are not present once the molar teeth have erupted. Enamel is initially produced as a proteinaceous matrix, which is then mineralized and so, in routine decalcified sections, the mineral is removed and mature enamel is not visible leaving an artefactual ‘space’ in the section—however, basophilic remnants of enamel may be seen in some sections (Figure 3.2). The outer layer of enamel of the incisors also incorporates iron salts but these only reach a high enough concentration to give the characteristic orange appearance to the maxillary incisors in mice (Moinichin et al. 1996). Odontoblasts are tall columnar cells with oval nuclei that line the pulp cavity. The nuclei tend to be at varying heights giving the layer a disorganized appearance. Odontoblasts secrete predentine and have a fine apical cytoplasmic process (Tomes fibre), which extends in a tubular space within the predentine layer. The predentine eventually mineralizes. The predentine stains more palely basophilic than the mature dentin (Figure 3.2). The pulp cavity contains a loose connective matrix with embedded blood and lymph vessels (Figure 3.2).
Figure 3.1 Continuously erupting incisor tooth in the mouse has regular layer of ameloblasts (thin arrow). Enamel is often lost during histological processing, leaving an artefactual space between ameloblasts and dentine (star).

Figure 3.2 Incisor tooth showing enamel remnants retained after processing (thick black arrow), ameloblasts (thin arrow), odontoblasts (white arrow), predentine (blue arrow), dentine (red arrow) and pulp cavity (star).

The continuous eruption of the incisors means that the process can be disrupted by trauma, nutritional deficiencies and genetic modifications. Disruptions can result in macroscopic changes, including loss of colour, changes in texture and fragility and breakage of the teeth. There is a spontaneous incidence of dental dysplasia of up to 10% in CD1 mice (Losco 1995) and periodontal inflammation is relatively common (Figure 3.3). Minor disruptions in odontoblast and ameloblast function may be detected by irregularities in enamel or dentine deposition (Figure 3.4). Rodent molars first erupt at about day 15 post natal and continue to erupt through the first four months of life. Odontoblasts remain viable within molar teeth, but are metabolically relatively inactive in adult animals (Figure 3.5).
Figure 3.4 Irregularities of predentine and dentine may be early indicators of abnormal enamel formation.

3.2.3 Sampling tongue
The small size of the mouse tongue means that complete longitudinal sections will fit into standard-size tissue cassettes. To ensure that the lingual salivary glands are present in the section, the tissue should be cut lateral to the midline (Ruehl-Fehlert et al. 2003).
3.2.4 Anatomy and histology of tongue
The tongue is covered by papillae, covered in a stratified squamous epithelium that is keratinized on the dorsal surface (Figure 3.6). Taste buds consist of outer supporting sustentacular cells and the inner elongate chemoreceptor (taste) cells (of which there are three types) (Figure 3.7) connected to nerve fibres. The taste buds are predominantly present in the caudal circumvallate papillae of the tongue although they may also be present at other sites in the oral cavity (palate) and pharynx (Treuting and Morton 2012). Serous (von Ebner’s glands) and mucous salivary glands are present in the caudal part of the tongue, with ducts emptying on to the dorsal surface (Figure 3.6). The core of the tongue is composed of skeletal muscle arranged in longitudinal and transverse bundles. The muscle is primarily of a fast-twitch type, but the predominant myosin heavy chain varies between muscle bundles (Abe et al. 2002). Heavily granulated mast cells are commonly seen in the connective tissue between the muscle bundles (Figure 3.8).
Figure 3.6 Caudal dorsal tongue showing keratinized squamous epithelium and mucous and serous glands.

Figure 3.8 (a) Heavily granulated mast cells (arrows) are commonly seen in normal rodent tongues and can be demonstrated with (b) Toluidine Blue staining.

3.3 Salivary glands
3.3.1 Sampling salivary glands
The mouse has three paired major (encapsulated) salivary glands, the largest of which is the submandibular gland, which is a prominent feature subcutaneously in the ventral neck. All the glands are composed of lobules and have a tubuloalveolar structure. The sublingual and parotid glands lie lateral to the submandibular glands with the sublingual gland in the more cranial portion. The submandibular lymph node, although usually cranial to the salivary glands, can be rather variable in position particularly if altered in size. It may be difficult macroscopically to distinguish and therefore to individually dissect the tissue of the major salivary glands and submandibular lymph node, so it is often convenient to embed the whole complex of tissue and create a single section (Ruhel-Fehlert et al. 2004). The mouse also has a number of minor salivary glands which are not distinct encapsulated structures like the major salivary glands but are embedded in their associated tissues. They are therefore usually sampled incidentally with the tissues they are associated with—for example, tongue, pharynx, palate.
3.3.2 Anatomy and histology of the salivary glands
All salivary glands are composed of ‘secretory end pieces’ which are acinar or tubular arrangements of glandular epithelium which empty via intercalated and striated (intralobular) ducts into excretory (interlobular) ducts ending ultimately in the oral cavity (Miletich 2010). In the submandibular gland of the mouse the secretory acinar cells are pyramidal with a mixed seromucous, basophilic cytoplasm and basally located nuclei. There is testosterone-dependent sexual dimorphism in the submandibular glands of mice. In males the acinar cells may contain more zymogen granules and more strikingly the cytoplasm of the convoluted ducts (which connect the intercalated and striated ducts) contains much more prominent eosinophilic granules (Figure 3.9 and Figure 3.10).
Figure 3.9 Male salivary glands with prominent eosinophilic granulation of convoluted ducts of the submandibular gland.

The sublingual gland is composed of large columnar mucous-secreting cells, which have pale basophilic cytoplasm (in H&E sections) containing large granules that compress the nuclei basally (Figure 3.11). Mucous cells can be demonstrated with alternative stains, for example Alcian blue, which stains the granules blue.
The parotid gland is composed of serous secretory cells, which have a darker basophilic cytoplasm (Figure 3.9). The parotid gland is histologically similar in appearance to both the lacrimal gland and the pancreas. Due to their close anatomic proximity, the lacrimal and parotid glands may appear in the same section but theoretically can be distinguished by the larger amount of cytoplasm in the acinar cells of the lacrimal gland. Although not anatomically co-located, the pancreas may be hard to distinguish from salivary tissue when in fixative so these tissues may be mistaken during the selection and embedding process. Pancreatic tissue can be identified by the presence of islets of Langerhans and the absence of striated ducts (Figure 3.10 and Figure 3.41).
In all of the salivary glands, the ducts are lined by simple cuboidal to columnar epithelial cells. The intercalated duct cells have a low cuboidal lining and these may be hard to appreciate, particularly in the submandibular gland. Striated ducts are often seen in circular cross section and have apically arranged nuclei due to basal invaginations of the plasma membrane, which enclose vertically arranged arrays of mitochondria and give rise to the striated appearance (Figure 3.11).
Sialoliths and foci of inflammatory cells may be found incidentally in the salivary glands of ageing mice (Figure 3.12).
3.4 Stomach and intestines
3.4.1 Sampling stomach and intestines
The presence of digestive enzymes, bile, acid, digesta and commensal bacterial flora within the intestinal tract means that it is prone to rapid autolysis. The timing of autolysis is variable and can depend on a number of intrinsic and extrinsic factors such as the environmental temperature, pyrexia and even the pathogen status of animals (Cross and Kohler 1969). Rapid fixation of the intestines is therefore very important. Immersion fixation soon after death is often adequate but injection of fixative into the lumen may be helpful in preventing changes and removing intestinal contents.
The mouse stomach wall is very thin, which can make consistent sampling difficult. Whichever method is used it is important to be able to examine the glandular and nonglandular stomach including the transitional region (limiting ridge) and the pylorus. Opening the stomach along the greater curvature and pinning it flat at necropsy (Ruehl-Fehlert et al. 2003) provides good fixation and allows easy orientation of the stomach to provide longitudinal samples through the major regions (mainly from the lesser curvature as this is in the mid line of the pinned tissue). However the small size of the mouse stomach allows for fixation of the organ as a whole, bisection along its length after fixation and embedding one complete half of the stomach, allowing examination of tissue from the greater and lesser curvatures.
As it is not easy to distinguish the different anatomical regions of the small intestine microscopically, it is important to make sure that the small intestine is sampled in a consistent way and that samples can be tracked through to the slide. The duodenum (Figure 3.13) is very short (approximately 2 mm in the mouse) and so should be sampled close to the pylorus; the jejunum can be confidently sampled by taking a section mid-way along the small intestine and the ileum can be sampled within 2 cm of the caecum. Peyer’s patches can usually be seen through the serosa (Chapter 1, Figure 1.29) allowing for sampling if necessary. As the anatomical appearance of the intestines can be altered by pathology, it is helpful to ensure that samples are placed into tissue cassettes for processing in such a way that the samples can later be accurately identified, for example by using tissue spacers, placing the tissues in a repeatable pattern (Figure 3.14) or by separating distinct areas of intestine into different blocks.
Figure 3.13 Short length of the duodenum demonstrated in subgross image. Duodenum is characterized by Brunner’s glands, which are present between the two black lines.

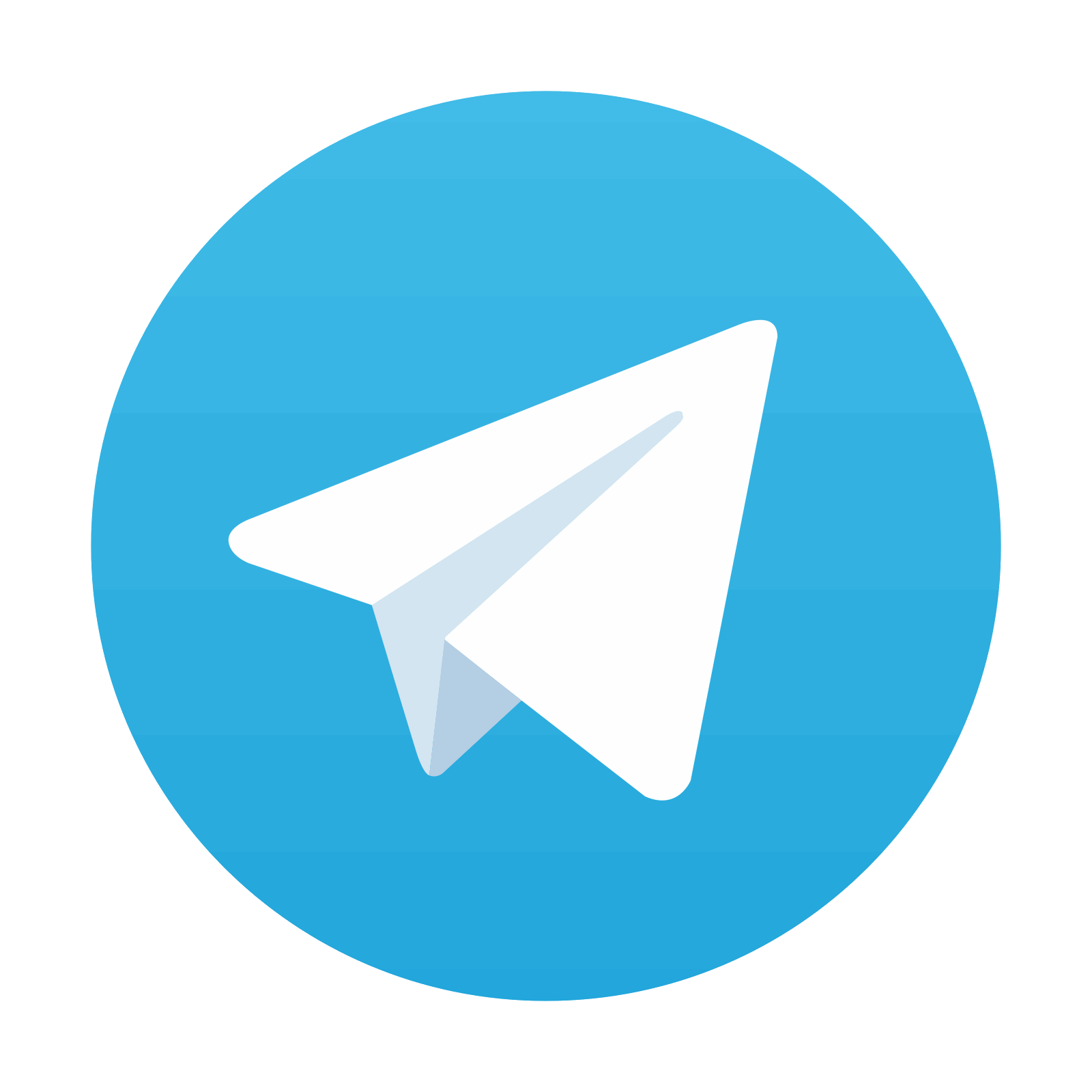
Stay updated, free articles. Join our Telegram channel

Full access? Get Clinical Tree
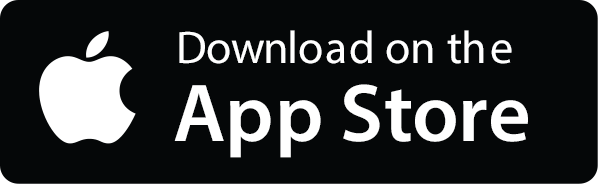
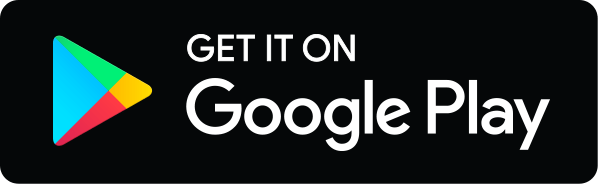