CHAPTER 4 Gametogenesis
At fertilization, the maternal and paternal genomes are united in the one-cell fertilized ovum, forming the zygote. In order to carry the two genomes to the site of unification in the oviduct, specialized cells known as the gametes have developed. The maternal gamete, the oocyte, is the largest cell of the body and has an inherent competence to initiate embryonic development once it has undergone a process known as activation, described under fertilization in Chapter 5. This particular ability of the oocyte is used biotechnologically, especially in cloning by nuclear transfer. Cloning involves removing the oocyte’s own genome and then having its remaining cytoplasm initiate embryonic development based on the genome of a nuclear donor cell fused to the oocyte (see Chapter 21).
PRIMORDIAL GERM CELLS
The primordial germ cells are the predecessors of the female and male gametes. When the embryo differentiates into the somatic germ layers (ectoderm, mesoderm and endoderm) during the process of gastrulation (see Chapter 7), most cells lose their pluripotency (the ability to develop into all cell types of the mammalian body). However, one set of cells remains pluripotent. These are the primordial germ cells which, at least in the pig, first become recognizable in the posterior rim of the embryonic disc at gastrulation. From here they move into the newly formed mesoderm and endoderm (Fig. 4-1). A few days later, the primordial germ cells are found in the visceral mesoderm surrounding the yolk sac and the allantois outside the embryo proper. Presumably, the primordial germ cells are brought to this location outside the forming embryo in order to ‘rescue’ them from the differentiation signals driving gastrulation within the embryo proper. By the time the first somites are formed, the primordial germ cells can be found in the mesoderm of both the yolk sac and the allantois, but also in the mesoderm of the incipient genital ridge where they are ready to populate the developing gonad (Figs 4-2, 4-3, 4-4). Thus, actively or passively, the primordial germ cells are brought along the caudal aspect of the yolk sac and the allantois into the primitive mesentery and then into the still undifferentiated, but developing, gonad. During their relocation, the primordial germ cells can be recognized by their relatively large size and by the use of special staining techniques such as those for alkaline phosphatase activity or the transcription factor OCT4 which is involved in maintaining cellular pluripotency (Figs 4-3, 4-4).
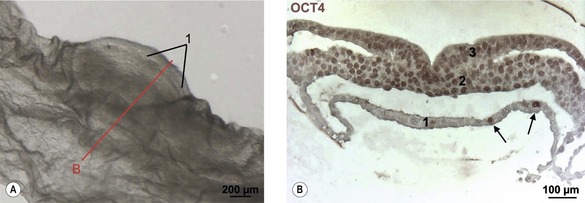
Fig. 4-1: Positioning of primordial germ cells in the endoderm. A: Embryonic disc of pig embryo at Day 14 of development. Note the primitive streak (1). The line ‘B’ indicates plane of section for Fig. 4-1B. B: Transverse section of the embryonic disc stained for Oct4. Note the individual Oct4 stained nuclei in the endoderm (1) identifying the primordial germ cells (arrows). 2: Mesoderm; 3: Ectoderm;.
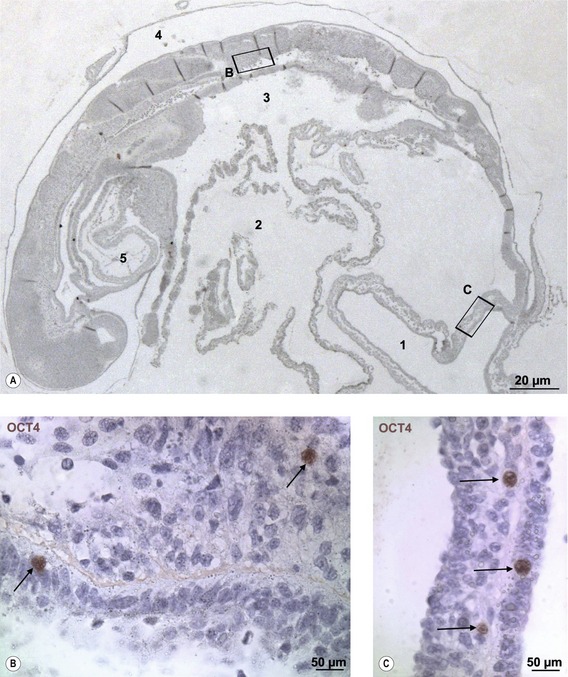
Fig. 4-4: Sagittal section of a porcine embryo at Day 16 of development (A). The section is stained for Oct4. The boxes ‘B’ and ‘C’ are enlarged. B: The nucleus of two primordial germ cells (arrows) located in the region of the developing gonadal ridge. C: The nuclei of three primordial germ cells (arrows) in the wall of the allantois (1). 2: Yolk sac; 3: Primitive gut; 4: Amniotic cavity; 5: Heart.
Modified from Vejlsted et al. (2006). Reprinted with permission of John Wiley & Sons, Inc.
During and after their migration, the primordial germ cells proliferate by mitoses. The female (XX) and male (XY) primordial germ cells become engaged in sex-specific differentiation of the gonads and become surrounded by somatic cells (see Chapter 15). They are now referred to as oogonia and spermatogonia, respectively. These cells undergo further proliferation before they enter gametogenesis and, thus, meiosis.
THE CHROMOSOMES, MITOSIS AND MEIOSIS
The traits of a new individual are determined by specific genes identified as nucleotide sequences on the deoxyribonucleic acid (DNA). It is important to keep in mind that it is not the gene sequences themselves, but the balanced and controlled expression of genes controlled by epigenetic regulation that is crucial for the behaviour of the cells and, thus, for the development of the conceptus. Together with numerous proteins, DNA makes up the chromosomes. The chromosomes of an individual are inherited from the mother and the father. In humans, where the genes have been extensively mapped through the Human Genome Project (HUGO), it is estimated that there are about 25 000 genes on the 46 chromosomes. The number of functional genes in the domestic animals is probably similar.
In somatic cells, chromosomes appear as homologous pairs to form the diploid chromosome complement. The diploid chromosome number is designated 2n as it includes a maternal and a paternal copy of each chromosome. The diploid chromosome numbers in the domestic animals are listed in Table 4-1. One pair of chromosomes comprises the sex chromosomes, while the others are referred to as autosomes. If the sex chromosome pair is XX, the individual is genetically a female; if XY, genetically a male. One chromosome of each pair is inherited from the mother through the oocyte and the other from the father through the spermatozoon. Thus, in order to result in a normal diploid chromosome complement at fertilization, the gametes must contain only one chromosome from each pair, referred to as the haploid chromosome complement and designated 1n. Gametes, then, contain only half the number of chromosomes present in somatic cells.
Mitosis
Mitosis is the process whereby a cell divides its chromosome complement evenly between its daughter cells; karyokinesis describes the complete process, including mitosis, through which the nucleus of a cell gives rise to a nucleus in each of its daughter cells. Normally, karyokinesis is accompanied by cytokinesis, the division of the cytoplasm (including organelles and inclusions) between the daughter cells. Thus, karyokinesis and cytokinesis in combination give rise to two daughter cells that in principle are genetically identical to the parent cell, each receiving the complete diploid chromosomal complement (Fig. 4-5). Mitosis is one phase of the somatic cell cycle, the other phase being interphase. Interphase is subdivided into a gap phase 1 (G1), a DNA-synthetic phase (S) and a gap phase 2 (G2). During the S-phase, each chromosome replicates its DNA to produce two chromatids. As already mentioned, the diploid chromosome complement is referred to as 2n. Before the S-phase, each chromosome consists of a single DNA strand, and so each gene is present in two copies (2c), one of maternal and one of paternal origin. After the S-phase, because each chromosome now consists of two chromatids, each gene will be present in four copies (4c) although the chromosome number is maintained at 2n. During the G1-, S- and G2-phases the chromosomes are extremely long, spread through the nucleus in particular domains, and cannot be recognized with the light microscope.
Mitosis can be subdivided into pro-, meta-, ana- and telo- phases. When the cell moves from G2 of the interphase into the prophase of mitosis, the chromosomes coil, contract, condense and thicken (Fig. 4-5 B). They become visible with the light microscope and each can be seen to consist of two chromatids, joined at a narrow region known as the centromere. Also during the prophase, the centriole pair duplicates in the cytoplasm adjacent to the nucleus and the resultant two pairs become arranged at opposite poles of the nucleus. As the cell moves from pro- towards metaphase (a stage often referred to as pro-metaphase), microtubules starts to form from the two pairs of centrioles and the nuclear envelope begins to dismantle (Fig. 4-5 C). Some of the microtubules from each centriole pair then attach to structures on the chromatids, the kinetochors, that are found in the centromere of each chromosome. This establishes the mitotic spindle with a centriole pair at each pole. Other microtubules pass continuously from one centriole pair to the other without attaching to the chromosomes. As the spindle forms, the chromosomes line up in its equatorial plane, defining the metaphase of the cell division (Fig. 4-5 D). Subsequently, the centromere of each chromosome divides, the two chromatids separate and, pulled by the microtubules, they start to migrate towards the spindle poles during anaphase (Fig. 4-5 E). By this stage, each chromatid has transformed into a new chromosome. Arrangement of the chromosomes into two groups, one at each spindle pole, establishes the telophase of the division (Fig. 4-5 F). Further into telophase, the chromosomes uncoil and lengthen, and a nuclear envelope re-forms in each of the nascent daughter cells (Fig. 4-5 G). This event completes the division of the nucleus and its DNA (karyokinesis) into two daughter nuclei that contain the same DNA sequences and the same number of chromosomes as the initial parental nucleus (2n, 2c). Karyokinesis is followed by cytokinesis to divide the cytoplasm equally between the daughter cells.
Meiosis
Crossover and genetic recombination
As in mitosis, maternal and paternal germ cells (oogonia and spermatogonia) undergo an S-phase before initiating meiosis. This forms two chromatids in each chromosome (2n, 4c) as in mitosis. This is the status of the germ cell when it enters the leptotene stage of the prophase of meiosis I (Fig. 4-6). In contrast to mitosis, however, the homologous chromosomes align in pairs during the prophase of meiosis I. Each chromosome pair therefore consists of four chromatids (4c) and is therefore referred to as a tetrad.
During diakinesis, the homologous chromosomes are gradually released from each other. With the transition from diakinesis to metaphase of meiosis I, the nuclear envelope disappears and meiotic spindles are formed. In the male germ cell, the poles of the meiotic spindle are constituted by centriole pairs as during mitosis. However, in at least the large domestic species and in contrast to those of mice, the oocyte lacks centrioles and the spindle poles are made up by unknown material ultrastructurally recognizable as small clusters of vesicles. The microtubules of the spindle attach to each homologous chromosome in a tetrad in meiosis I instead of to the chromatids as they do in mitosis. During the metaphase of meiosis I the chromosomes align in the equatorial plane but, during anaphase, it is the homologous chromosomes, not their chromatids, that are pulled apart to become grouped at the poles of the spindle during telophase. Thus, in contrast to mitosis, meiosis I separates homologous chromosomes rather than chromatids. Upon completion of meiosis I, the germ cell is haploid, containing only one chromosome (1n) from each chromosome pair. It must be underlined, however, that the DNA content of the germ cell at this stage is still 2c because each chromosome consists of two chromatids.
Spermatocytes, spermatids, oocytes and polar bodies
At the end of telophase of meiosis I, the primary spermatocyte divides into two secondary spermatocytes by cytokinesis (Fig. 4-6). In the oocyte, however, the spindle is located at the periphery of the large spherical cell, and telophase is associated with a very uneven cytokinesis resulting in one of the two daughter cells being much larger than the other. The larger daughter cell is now referred to as a secondary oocyte while the small daughter cell, which is almost devoid of organelles, is the first polar body.
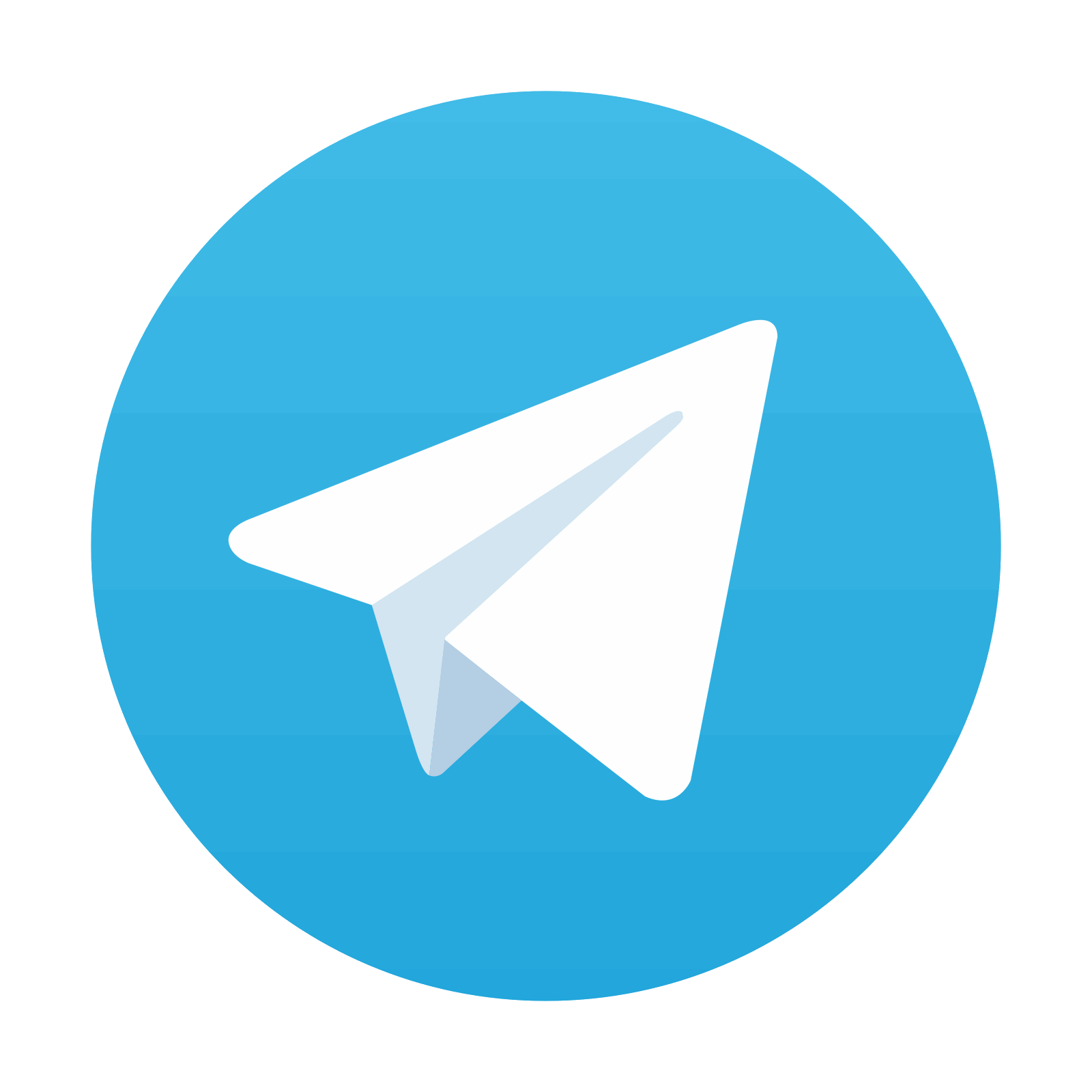
Stay updated, free articles. Join our Telegram channel

Full access? Get Clinical Tree
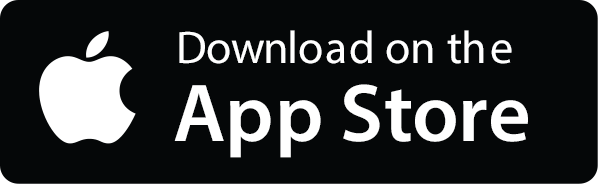
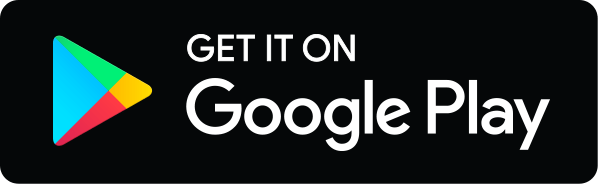