CHAPTER 5 Fluid Therapy
Steady State and the Concept of Maintenance
Pathologic loss of water can result from the following:
Body Fluid Compartments
Figure 5-1 depicts the cat’s body fluid compartments. The body’s two main fluid compartments are the intracellular fluid (ICF) and the ECF. Approximately 66% of functional total body water is located within the ICF compartment, and 33% is in the ECF compartment.
The ECF space is composed of four main subcompartments: interstitial, intravascular, transcellular, and bone and dense connective tissue. The intravascular fluid is that which is contained within blood vessels; it contributes only 8% to 10% of total body water (5% of body weight) and has been estimated to be approximately 45 mL/kg in cats.10
Fluid Movement in the Extracellular Fluid Compartment
The water in the body’s ECF compartments is in a constant state of flux. Fluid moves across the capillary membrane, which is composed of endothelial cells that contain gap junctions through which fluid and solutes can flow. Solutes dissolved in fluid move from an area of higher to lower concentration along concentration gradients by the process of passive diffusion. The factors that regulate this transport of fluid and the electrolytes and other molecules it contains are called Starling forces (Box 5-1). The key factors are the hydrostatic and colloid oncotic pressure gradients between the intravascular and extravascular spaces. The hydrostatic pressure is greater in the capillary than in the interstitium, and the gradient favors fluid movement (filtration) out of the capillary. The colloid oncotic pressure, determined by protein concentration, is also greater in the capillary, and this tends to draw fluid into the capillary. Simplistically, at the beginning of the capillary the high hydrostatic pressure results in fluid egress into interstitium. As the fluid leaves along the length of the capillary, the hydrostatic pressure falls and the colloid oncotic pressure increases, resulting in fluid reentry into the capillary lumen toward the end of the capillary. Fluid also leaves the interstitial compartment by way of the lymphatics.
Salt Balance: Disorders of ECF Volume
Regulation of Sodium Balance
Regulatory mechanisms exist to control both Na+ content and Na+ concentration ([Na+]), and they are interrelated. Body Na+ content is regulated by mechanisms that control the renal excretion of Na+ and which operate in response to body fluid volume and not [Na+].9 Control of [Na+] is determined by the osmoregulatory control mechanisms.
Evolutionarily, it appears that salt was a scarce commodity. Thus the kidney has evolved mechanisms to conserve salt. Na+ excretion in the urine can vary over 500-fold depending on Na+ intake and body need. The homeostatic mechanisms that control Na+ content are poorly understood. Regulation is generally a comparatively slow process. For example, many hours will pass before excesses in Na+ content (e.g., when isotonic saline is infused) are corrected by increased renal Na+ excretion. In contrast, excesses or deficiencies of water relative to Na+ (changes in [Na+]) activate the osmoregulatory mechanisms and are dealt with very rapidly. Many physiologists believe that a set point for Na+ regulation does not exist. Rather, Na+ is retained in low volume states until the volume deficit is corrected.12
The following factors are known to affect renal Na+ excretion:
1 Diuresis (e.g., diuretic phase of acute tubular necrosis, postobstructive diuresis, or the use of diuretic medications)
2 Intrinsic renal disease (e.g., chronic renal failure, Fanconi syndrome, Bartter syndrome)
3 Defects in the RAAS system (e.g., hypoadrenocorticism, hyporeninemic hypoaldosteronism)
Water Balance: Disorders of Sodium Concentration
Understanding Fluid Losses
Sensible and Insensible Fluid Losses
Insensible losses are those that cannot directly be measured. They include largely solute-free water in evaporative respiratory, sweat, and salivary losses. This classical definition of insensible losses is sometimes replaced with a clinical definition that includes fecal water loss. This is because the amount of normal daily fecal water loss is small and is rarely measured.16
As a gross approximation, sensible fluid losses account for half of a healthy animal’s daily fluid requirement and insensible losses account for the other half. However, this partitioning is variable and is species and environment dependent. For example, dogs as a species may have a higher percentage of insensible losses compared with cats because of the greater use of panting for thermoregulation. However, cats may have considerable salivary losses if they have increased grooming or lick their fur to promote evaporative cooling in hot weather.7
Insensible fluid losses are generally considered to be solute-free water because respiratory losses are the major contributor in small animal species, including cats. Insensible losses are estimated to be 12 to 30 mL/kg/day, depending on the study and definition.16
Relationship to Lean Body Mass
Because lean body mass is so important in determining an animal’s daily fluid need, resting energy requirement (RER), or daily caloric requirement, is used to calculate an animal’s metabolic water requirements: To metabolize 1 kilocalorie of energy, 1 mL of water is consumed. As such, calculation of an animal’s RER can be extrapolated to determine the volume of fluid in mL required in a 24-hour period. Several equations may be used to calculate RER and hence water requirement.5 The following is one of the most commonly used:
Assessment of Fluid loss
The body responds to fluid loss by redistributing the functional ECF volume—that is, by pulling fluid into the intravascular space from the interstitial space to maintain circulating blood volume as the first priority. When the interstitial space can no longer replenish intravascular volume depletion, clinical signs of hypovolemia will result. In decompensated shock, severe hypovolemia results in a marked worsening of perfusion parameters. Hypotension, bradycardia, prolonged CRT, pale pink to gray or cyanotic mucous membranes, hypothermia, decreased central venous pressure, altered mentation, and decreased urine output will be present in decompensated shock. Table 5-1 gives examples of the types of fluid losses that would be expected with selected medical problems.
TABLE 5-1 Examples of the Types of Fluid Losses that Would Be Expected with Selected Medical Problems
Condition | Dehydration | Hypovolemia |
---|---|---|
Blood loss | X | |
Vomiting | X | X (if severe) |
Diarrhea | X | X (if severe) |
Sepsis/vasodilation | X | |
Hypoadrenocorticism | X | |
Polyuria | X (depending on cause) | X (depending on cause) |
Hypodipsia or water deprivation | X |
Body Response to Hypovolemia
Cats are unique in that the vasoconstrictor response to volume loss is blunted in the presence of hypothermia.13,14 For this reason, cats are more prone than other species to fluid overload when they have been volume resuscitated while hypothermic. Once body temperature returns to normal, the vasoconstrictor response returns and intravascular pressure rises. For this reason, hypothermia in cats is a potentiator of shock, as well as a result of shock. Cautious fluid resuscitation must coincide with aggressive rewarming efforts to prevent volume overload. Specific therapy and therapeutic endpoints for resuscitation are discussed in subsequent sections.
General Considerations for Fluid Therapy
As fluid bag sizes are not scaled down to the size of feline patients, it is helpful to use fluid pumps, burettes, and other devices to prevent fluid overload and pulmonary edema or pleural effusion (Figure 5-2).
Fluid Types
Crystalloids
Isotonic High-Sodium Crystalloids
General Characteristics and Indications for Isotonic High-Sodium Crystalloids
Isotonic high-sodium fluids are commonly referred to as replacement fluids because they are often used for rapid replacement of ECF volume deficits caused by vomiting and diarrhea. They have a [Na+] near that of ECF, ranging from approximately 130 mEq/L (e.g., lactated Ringer’s solution [LRS]) to a high of 154 mEq/L (e.g., 0.9% saline). Table 5-2 includes additional examples of replacement fluids, highlighted in red.
TABLE 5-2 Composition of Common Crystalloids*
* High-sodium “replacement” fluids are in red. Low-sodium “maintenance” fluids are in green.
Sick cats that have been anorexic for 2 to 3 days or longer should receive nutritional support. Generally, the provision of enteral or parenteral nutrition sufficient to meet the patient’s caloric needs will also provide maintenance fluid needs. Thus the use of additional isotonic, high-sodium fluids in this setting will be to replace excessive isotonic losses, such as those associated with gastrointestinal loss or polyuria. Intravenous fluid rates can be greatly reduced in patients receiving either enteral or parenteral nutrition to a rate sufficient to meet additional ongoing losses only. In other words, the fluid therapy recipe should account for all sources of fluid intake (Table 5-3; also see Table 5-8).
Supplements
In some situations fluids must be supplemented with additional electrolytes; this decision is based on an assessment of the history, physical examination findings, and measured electrolyte values. Commonly added electrolytes are listed in Table 5-4. Electrolyte additives may be appropriate for replacing deficits, providing replacement for normal maintenance losses in anorexic patients, compensation for transcellular movement of ions, or replacement of ongoing gastrointestinal or urinary losses. Potassium and magnesium are found in some low-sodium hypotonic fluids formulated as maintenance fluids. If not, they can be added to fluids to maintain homeostasis in animals that are not depleted.
TABLE 5-4 Concentration of Common Fluid Additives
Product | Concentration per mL |
---|---|
KCl | 2 mEq each |
KPO4 | 4.4 mEq K+, 3 mM PO4 |
MgCl | 1.97 mEq each |
MgSO4 | 4.06 mEq each |
Ca gluconate 10% | 0.465 mEq Ca++ |
CaCl2 | 1.36 mEq Ca++ |
NaPO4 | 4 mEq Na+, 3 mM PO4 |
Dextrose 50% | 500 mg |
From Abbott Animal Health Fluid Therapy Module 2, courtesy Dr. Steve Haskins.
Potassium
For patients that are hypokalemic, a sliding scale is used to calculate how much potassium to add to the fluid. One such scale is shown in Table 5-5. When using the potassium-containing replacement fluids for fluid resuscitation, it must be remembered that if the K+ concentration of the fluid exceeds 5 mEq/L, the fluid must not be infused rapidly for intravascular volume restoration because of the risk of hyperkalemia.
TABLE 5-5 Sliding Scale for the Amount of KCl Added to Intravenous Fluids Depending on the Serum [K+]*
Measured Serum K+ (mEq/L) | KCl Added (mEq/L) |
---|---|
>5.5 | None |
3.6-5.5 | 20 |
3.1-3.5 | 30 |
2.6-3 | 40 |
2-2.5 | 60 |
<2 | 80 |
* If the [K+] of the fluid exceeds 5 mEq/L, the fluid must not be infused rapidly for intravascular volume restoration because of the risk of hyperkalemia.
Cats with anorexia, gastrointestinal losses, or polyuria are particularly at risk for K+ depletion. As an alternative to the sliding scale, a constant-rate infusion (CRI) is typically used to give K+ separately when the patient seems resistant to “normal” amounts of K+ supplementation, particularly in diabetic ketoacidosis (DKA) (Figure 5-3). This allows K+ to be adjusted separately from the remainder of the fluid prescription. If CRI is used, it must be monitored very carefully. The usual dose range to replace normal ongoing losses of potassium is 0.05 to 0.1 mEq/kg per hour. The dosage for cats with severe whole body potassium depletion, severe symptomatic hypokalemia, or both can be as high as 0.5 mEq/kg per hour, known as KMax. Administration at rates higher than 0.5 mEq/kg per hour can cause serious or fatal cardiac arrhythmias. Administration of undiluted KCl (2 mEq/mL) through a programmable syringe pump is possible, but should only be done with extreme care, reserved for intensive care situations in patients with life-threatening hypokalemia (generally levels below 1.5 mEq/L).
Magnesium
Replacement doses are 0.03 to 0.04 mEq/kg per hour for severe cases requiring rapid replacement and 0.013 to 0.02 mEq/kg/hour for more mild deficiency. MgCl2 or MgSO4 may be given but not mixed with calcium- or bicarbonate-containing fluids.2 Patients with reduced GFR are at greater risk for hypermagnesemia during supplementation and require more frequent monitoring. Magnesium is a cofactor for potassium homeostasis, and magnesium supplementation should be considered in any patient with refractory hypokalemia.
Hypotonic Low-Sodium Crystalloids
Maintenance
Table 5-2 lists some examples of low-sodium crystalloid fluids in green. The rate of administration of IV fluids used for maintenance is based on the metabolic body size and will only change when patients begin to eat and drink. Low-sodium crystalloids are contraindicated in patients requiring rapid administration, such as for hypovolemia, as doing so will rapidly reduce the ECF [Na+] and cause cell swelling due to rapid reduction in osmolality.
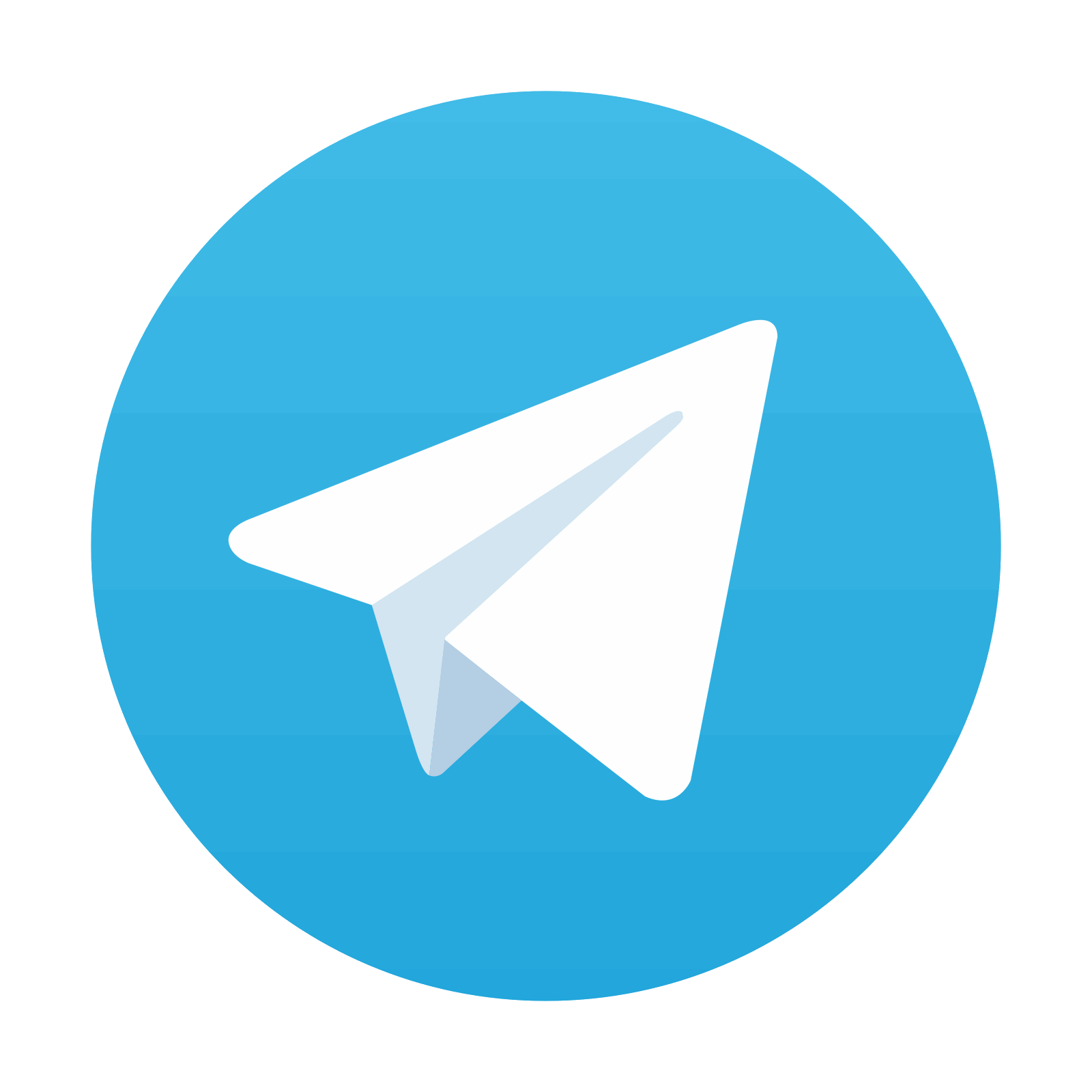
Stay updated, free articles. Join our Telegram channel

Full access? Get Clinical Tree
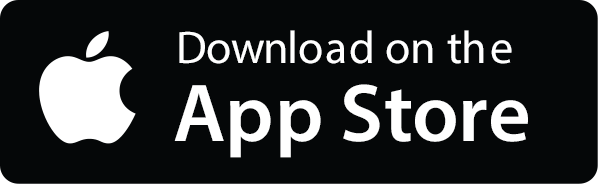
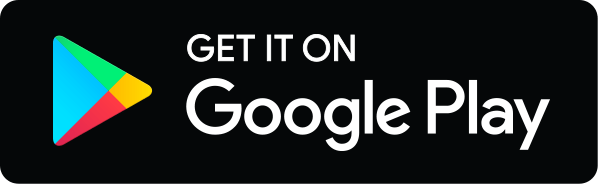