Fig. 18.1
Targets for reproductive inhibition
The main methods used for wildlife contraception will be reviewed in the following section, together with their advantages and disadvantages, particularly in relation to different species.
3 Hormonal Methods
3.1 Steroid Implants
The use of steroid hormones to inhibit female reproduction has generally been found to be successful in several species. Contraceptives, such as the synthetic progestin melangestrol acetate (MGA), have been used in zoos to control the fertility of captive large cats, while levonorgestrel (developed for human female contraception), has been found to be a suitable long-term contraceptive in tammar wallabies (Herbert et al. 2007) and koalas (Hynes et al. 2010). Levonorgestrel would seem to act in koalas by inhibiting ovulation, not by preventing follicular development and cycling. Interestingly, etonogestrel was not found to be an effective contraceptive in this species. In captive and wild populations of eastern grey kangaroos and tammar wallabies, levonorgestrel successfully prevents reproduction by inhibiting oestrus (Nave et al. 2002). It is not completely understood how levonorgestrel acts in both species, as it does not appear to inhibit follicular development. However, ovulation may be affected, as there is a suggestion that the pre-ovulatory surge of luteinising hormone is inhibited (Hynes et al. 2007). Levonorgestrel does not affect the reactivation and subsequent development of blastocysts in diapause that are conceived prior to treatment. Lactation is also unimpaired, as young were reared to weaning in both species. It would appear that levonorgestrel implants provide a safe, effective and long-term method of fertility control for macropodid marsupials and can be used for the management of overabundant captive and selected wild populations of these animals (Nave et al. 2000). However, in general it is recognised that steroid use is limited for free-ranging wildlife for a variety of reasons including: environmental toxicity, passage through the food chain, adverse effects on social behaviours, high cost of application (particularly for delivering the steroids remotely), health risks in pregnant animals and regulatory issues (Kirkpatrick et al. 2011).
3.2 Non-steroid Methods
Non-steroidal contraceptives, such as deslorelin acetate, an anti GnRH agonist, have also been used to control fertility in various wild carnivores and domestic dogs and cats. Deslorelin (Ovuplant®), was initially developed as an ovulation-inducing agent in mares (Farquhar et al. 2002), but it has more recently been used as a subcutaneous long-acting implant for the suppression of reproduction in both male and female wild and captive animals (Bertschinger et al. 2002; Johnson et al. 2003; Junaidi et al. 2003; Patton et al. 2006). It acts by down-regulating follicle stimulating hormone (FSH) and luteinizing hormone (LH), thereby suppressing ovulation and sperm production. For example, it has been found to be effective in female tammar wallabies in Australia (Herbert et al. 2005) and semi-captive male cheetahs in South Africa (Bertschinger et al. 2006). In the latter, deslorelin implants (6 mg) reliably suppressed fertility for a year, without side effects.
3.3 Unwanted Side Effects of Hormonal Methods
Although, steroids have proved an effective contraceptive in many species, some have also induced unwanted side effects. levonorgestrel, for example, has been implicated in side effects associated with the reproductive tract and also metabolic changes (Monier 1988; Zook et al. 2001). For example, in rabbits, those with levonorgestrel implants developed endometrial decidualisation or deciduosarcomas, especially with increased doses (+233 μg/day) of levonorgestrel, or if oestrogens were also included at doses over 60 μg/day (Janne et al. 2001). In primates, levonorgestrel combined with ethinyl oestradiol has some effect on female basal metabolic rate (Edelman et al. 2011). It also seems likely that metabolic changes seen in women who take oral contraceptives, such as increased blood pressure, insulin insensitivity and raised insulin and lipid levels, could be induced in animals.
Deslorelin implants appear to be safe for administration to males, although, in some species there is evidence of adverse reactions in females. In bitches there have been cases of prolonged oestrus, ovarian cysts and pyometra after deslorelin implants (Arlt et al. 2011). The risk of induction of oestrus can be reduced when such implants are administered at concentrations of progesterone in plasma of ≥16.0 pmol/L. More detailed studies are required in female dogs to confirm safety and currently it is suggested that a complete gynaecological examination be performed before implanting deslorelin. Additionally, it is recommended that the implant should be positioned subcutaneously, close to the umbilicus, in order to allow relocation and excision if necessary.
In the female tammar wallaby, the duration of contraception induced by deslorelin is highly variable amongst individuals and has been associated with a significant reduction in basal LH concentrations and a cessation of oestrous cycles (Herbert et al. 2005). Also, there is some evidence to suggest that aspects of blastocyst survival, luteal reactivation, pregnancy or birth may be affected by deslorelin treatment in some animals. Interestingly, in the male, deslorelin appears to have no contraceptive effect (Herbert et al. 2004). In this study, there was no evidence of a treatment-induced decline in plasma testosterone concentration or basal LH concentrations. These studies highlight the problems related to choosing a suitable long-acting contraceptive for a particular application, given the variation in contraceptive effects on individuals, species and gender.
Regarding the use of long-acting steroid and hormonal contraceptives in conservation scenarios, there are specific matters that need to be considered, particularly for those animals that live in groups. Altering hormone levels, both male and female, has the potential to alter social behaviour, including dominance hierarchies, which can influence access to food. If used for a lengthy period, therefore, contraception can result in a breakdown of the group structure, safety, health and ultimately negate the conservation goals (Pukazhenthi et al. 2006; Druce et al. 2011).
Practically, the use of contraceptive implants such as steroids and non-steroids like deslorelin are generally limited to small populations and captive animals rather than large scale population control. This is due mainly to the contraceptives being expensive and also, in wildlife situations (such as for the wallaby and kangaroo), often cannot be applied without first anaesthetising the animal. It is hoped such contraceptives will become cheaper and more easily applied to wildlife situations, if methods can be developed for reliably darting wild free-ranging animals from a distance without the need for anaesthesia.
A list of the types of contraception used for the control of various wildlife species is indicated in Table 18.1.
Table 18.1
Contraceptives used to control both wild and captive wildlife populations
Species | Contraceptive | Reference |
---|---|---|
Cheetah, Acinonyx jubatus | Deslorelin | Bertschinger et al. (2002) |
Domestic cat, Felis catus | Anti-GnRH Melengestrol | |
Domestic dog, Canis lupus familiaris | Anti-GnRH vaccine Testosterone | |
Elk, Cervus canadensis | Anti-ZP vaccine Diethyl stillboestrol (DES) | |
Elephant (African), Loxodonta africana | Anti ZP Progesterone | |
Leopard (African), Panthera pardus pardus | Deslorelin | |
Baboon, Papio cynocephalus | Levonorgestrel Anti-GnRH Anti-LDH | |
Rabbit, Oryctolagus cuniculus | Meloxicam (Cox 2 inhibitor) Anti-PH20 Anti-LDH | Naz et al. (1984) |
Deer, Odocoileus virginianus | DES Anti-ZP vaccine | |
Grey squirrel, Sciurus carolinensis | Anti-sperm | Moore et al. (1997) |
Mink, Neovison vison | DES | Asa et al. (1996) |
Rhesus monkey, Macaca mulatta | Anti-FSH Norethindrone Anti-FSH GnRH analogue |
4 Immunocontraception: Review of Current Vaccines and Uses
Immunocontraception is the use of vaccines to prevent the process of fertilisation. This involves harnessing the immune system to disrupt the reproductive process by targeting key components of the reproductive system, such as the reproductive hormones and gametes (sperm and oocyte). Research has mainly focussed on three areas: the reproductive hormones GnRH (Herbert and Trigg 2005; Schneider et al. 2006) and FSH (Moudgal et al. 1992; Delves and Roitt 2005; Yang et al. 2011); the disruption of fertilisation by preventing sperm–egg binding and thirdly, the inhibition of sperm function and motility (Suri 2004; Naz 2011). The latter two areas have involved the identification of oocyte and sperm proteins involved in sperm function (e.g. sperm–egg binding and sperm motility), which have then been used as targets for vaccine development. The three individual immunocontraceptive research areas referenced above have been extensively reviewed and will not be covered in detail in this chapter.
Although the research to develop safe and effective immunocontraceptives for animal use is ongoing, only two are currently marketed for animal use: GonaCon™ and SpayVac™. As such, the use of immunocontraceptives for animal population control has centred on both of these: GonaCon™, an anti-GnRH vaccine and SpayVac™, which targets a specific oocyte zonapellucida (ZP) protein, thereby preventing fertilisation. They have proved successful in a large variety of species, both at the population level and for individual animals and have been used in both captive and free-ranging species. In particular, they have been used for the population management of African elephants, wild horses, bison and deer. However, although both immunocontraceptives have been used to control feral cat populations, only anti-GnRH vaccines have been successful in this species (Levy 2011). Immunisation against GnRH has resulted in long-term contraception in both male and female cats following a single dose. GnRH is an ideal contraceptive target for feral cats, as it regulates both pituitary and gonadal hormone responses in males and females. This, therefore, has the added benefit of suppressing nuisance behaviours associated with sex hormones (spraying, fighting, mating calls) in addition to preventing pregnancy. In contrast, the use of anti-ZP vaccines such as SpayVac™ has shown little success in controlling feral cat fertility.
Although not applicable to all areas of population control, GonaCon™ and SpayVac™ have shown that immunocontraception is a feasible and effective tool for controlling fertility in animals. However, research continues to develop additional immunocontraceptives that may have more ideal profiles for fertility control. New targets are being found, such as the discovery of vesicle-associated protein 1 (a novel target isolated from the vesicle-rich hemisphere of the brushtail possum oocyte) (Nation et al. 2008), or a uterine-secreted protein CP4 that can affect conceptus development (Menkhorst et al. 2008). Preventing fertilisation is currently the most popular research area, as it offers a method that specifically prevents fertilisation without effects on the endocrine system and other physiological processes. This has advantages in that it does not affect reproductive hormone levels, negating effects on social behaviour, and the resultant breakdown of the group structure, safety and health. Anti-sperm vaccines have the additional benefit of potentially being effective in both males and females, although the majority of current research has been in the female. The anti-sperm vaccine targets are also sperm specific, thereby limiting side effects, and highly immunogenic which enhances their efficacy and likelihood of being more robust, long-acting and with less response variability between individuals. They are also more suitable for being administered in bait to a population, as they are active in both males and females, potentially reducing populations more quickly.
Numerous sperm proteins have been investigated for contraceptive purposes, many of which are listed in Table 18.2. More recent novel sperm targets include CatSper, Eppin (Chen et al. 2011), Izumo (Wang et al. 2009) and also the epididymal target, SFP2 (Khan et al. 2011). Although still at the research stage, the sperm protein targets potentially provide exciting possibilities for future wildlife contraception.
Table 18.2
Sperm proteins researched for potential immunocontraception
Sperm target | Reference | Sperm function affected |
---|---|---|
PH20 | Primakoff et al. (1988) | Sperm–egg interaction |
SP17 | Lea et al. (1998) | Sperm motility |
LDH C4 | Goldberg and Herr (2000) | Sperm motility |
SP10 | Herr et al. (1990) | Sperm–egg binding |
SAMP14 | Shetty et al. (2003) | Sperm–egg binding |
SPAG9 | Shankar et al. (1998) | Sperm–egg fusion, fertilisation |
TSA-1 | Santhanam and Naz (2001) | Sperm motility, capacitation |
hCRISP1 | Ellerman et al. (2010) | Sperm–egg binding |
Izumo | Wang et al. (2008) | Sperm–egg fusion, fertilisation |
CatSper1 | Li et al. (2012) | Intracellular Ca (2+) concentration, motility |
Current immunocontraceptive research is also investigating novel vaccine approaches, for example, the use of more than one target in an immunocontraceptive. This has the potential to make it more effective with fewer non-responders within a population. In addition, there is the opportunity to develop immunocontraceptives that combine contraception with disease control. Modern vaccine technology enables the development of combined vaccines and scientists are currently investigating the possible development of a GnRH vaccine, that also provides rabies protection for use in feral dog and fox populations (Wu et al. 2009).
The goal for the ideal immunocontraceptive includes a wide margin of safety for target animals and the environment, fast acting with a long duration of activity following a single treatment in males and females of all ages. The development, therefore, of improved anti-GnRH and anti-ZP immunocontraceptives, together with anti-sperm and combined vaccines, offers exciting new possibilities for effective, safe, long-acting practical wildlife fertility control. However, the barrier to success is a current key limitation for the funding of well-designed research programmes to develop immunocontraceptive fertility control products.
4.1 Immunocontraceptive Challenges
The challenges in the application of the current marketed vaccine-based wildlife contraceptives are similar to those of the steroid and hormonal contraceptives: that is differences in efficacy across species, safety and the need for practical and cost effective delivery systems for wild and free-ranging animals. Promisingly, to date, both GonaCon™ and SpayVac™ have been shown to be successful in controlling particular species, such as deer (Locke et al. 2007b; Miller et al. 2008) and elk (Killian et al. 2009), with few issues. Indeed, for wild and feral applications, immunocontraception has the potential to be a more practical and cost-effective method of fertility control than that of steroids and hormones. However, there are problems concerning immunity. For example, can sufficiently strong long-acting immune responses be provoked against the vaccine targets (immunogens) of gametes or reproductive hormones to cause contraception in a sufficient number of animals to produce effective population control? One problem relates to long-term use of immunocontraception in particular populations, whereby a resistant population of low or non-responders arises. This has led to research into understanding the immune responses in species such as brushtail possums, which has shown that there are different major histocompatibility complex (MHC) haplotypes that correspond to non-responsiveness (Holland et al. 2009).
New adjuvants that enhance the mucosal immune response are now available and more are being developed (Zaman et al. 2013). In particular, adjuvants that specifically target the reproductive tract immune response to enable good immune responses against sperm and egg target proteins have made preventing fertilisation using vaccines more effective (Zhang et al. 2007; Zaman et al. 2013). In some cases, combination of drug delivery systems with inherent adjuvant properties, also have proven advantages.
The development of improved anti-GnRH and anti-ZP immunocontraceptives, together with anti-sperm and combined vaccines, offers exciting new possibilities for effective, safe, long-acting, practical wildlife fertility control. However, the current lack of funding for well designed research programmes is a major barrier to success and a current key limitation for the development of advanced immunocontraceptive products.
Apart from the scientific questions, immunocontraception will need to meet the regulatory requirements for use in the environment and on the biological and economical feasibility of their use. In addition, widespread use will also depend on the health and safety requirements of individual countries and scenarios and on public acceptance of the techniques. To date, the success of GonaCon™ and SpayVac™ has gone someway to paving the way for further immunocontraceptives to be used for wildlife population control.
5 The Future of Immunocontraception: New Technologies
The drive in developments incorporating wildlife specifications has come from a pressing need to control disease such as rabies and tuberculosis. For these diseases as with immunocontraception of free-roaming populations, oral delivery using bait, remains the most convenient and cost-effective means of delivering vaccines to large or widely spread populations of animals. It is non-invasive and so concerns about injection site adverse effects are negated. Recently oral vaccination field trials of badgers to prevent tuberculosis have been reported (Gormley and Corner 2013). Nasal or inhalation delivery may emerge in the future, but currently there are only a limited number of reports of technologies with aerosolised potential (Corner et al. 2001; Corner et al. 2008).
5.1 Bait Delivery Related to Different Species
Based on a highly successful vaccine that has been established for many years and used extensively in the prevention of rabies, RABORAL V-RG®, demonstrates the success of vectors in vaccines, utilizing a vaccine-strain of Vaccinia virus as the live vector. This vector system allows delivery of a thermostable rabies vaccine contained in edible baits. Prior to being licensed by the USDA, the vaccine was approved for environmental release and a range of safety tests were carried out in non-target animals.
To be effective, oral formulations need to be stable under different field conditions (temperature, moisture, pH effects), but can be contained in weather-proof packaging (Cross et al. 2009). Flavouring or chewing stimulants to encourage species-specific and effective sampling may also be an option (Bergman et al. 2008; Ballesteros et al. 2009c), while baits can be placed underground or in trees to limit the species that have access to them (Buddle et al. 2011). Selective feeders have also been designed to reduce non-target consumption of bait (Ballesteros et al. 2011; Telford et al. 2011). Changing the consistency of an oral vaccine, such as using a viscous material that also acts as an adjuvant can improve vaccination rate in some species. Again, the latter has been demonstrated with inclusion of chitosan and methylated derivatives, with RABORAL V-RG® (Fry et al. 2012). Examples of various baits designed for use in different species include synthetic grit consisting of plasticizer and cross-linking agents targeted at Mallards (Hurley and Johnston 2002), lipid-based baits for a variety of potential TB reservoir species (Nol et al. 2008; Corner et al. 2010; Buddle et al. 2011) and cereal-based products to attract pigs and wild boar (Ballesteros et al. 2009a). Over-dosing should not endanger target or non-target species and it is also important to be able to evaluate vaccination coverage. Therefore, chemical markers such as the use of iophenoxic acid incorporated in the formulation can be detected easily in serum (Massei et al. 2009). Where baits are distributed aerially (using helicopters for example), computerised and geographical information systems are invaluable for tracking bait location (Mulatti et al. 2011), although the cost to benefit ratio must be evaluated carefully.
Thus it can be seen that creative designs are an essential part of wildlife vaccine formulation. However, effectiveness is key. To further enhance immunogenicity, different types of delivery system may be required and these include living and non-living technologies.
5.2 Living Vectors
Many reported successful studies vaccinating wild animals against infectious diseases use viral or bacterial vectors (Rocke et al. 2008; Ballesteros et al. 2009b). Therefore, it stands to reason that similar vector application could also provide an important strategy for contraceptive antigen delivery. This approach was initially investigated in Australia for the control of population in rabbits. As such, the strategy was to select a target molecule capable of inducing an immunocontraceptive response and insert the gene encoding the target into the myxoma virus for distribution into the rabbit population. This raised several concerns regarding safety and the possible transmission to other non-targeted species (Tyndale-Biscoe 1994). In the current research, species-specific viruses are now being genetically engineered to produce contraceptive vaccines for pest animals such as mice, rabbits and foxes. Laboratory experiments have shown that high levels of infertility can be induced in mice infected with recombinant murine cytomegalovirus and ectromelia virus expressing reproductive antigens as well as in rabbits using myxoma virus vectors (Gu et al. 2004; Hardy 2007; Nikolovski et al. 2009). However, safety and social concerns will need to be allayed prior to the use of these vaccines to control wildlife pests.
5.3 Non-living Particulates
In general cost of production and delivery is a prime consideration in the development of wildlife vaccines, while minor/short-term side-effects might be less of an issue. Another factor is genetics and genetic diversity of the target species, particularly as there is limited knowledge of this and impact on immune response. For example porcine ZP (pZP) vaccine tested in wild horses and deer was 85 % effective, whereas in African elephants efficacy fell by 10 % (Kirkpatrick et al. 2011). However, other studies in African elephants have indicated that the efficacy of pZP is equivalent to that in wild horses (Fayrer-Hosken et al. 1999). Although there are reported differences in vaccine efficacy between species, many particulate vaccine delivery systems are seen as a particularly attractive method. The main reasons are that they can be produced cheaply and generally target the innate immune system so do not have to involve sophisticated or knowledge-based inventions. Particulate systems include: organic (e.g. lipid-based) and inorganic (e.g. inert beads) technologies.
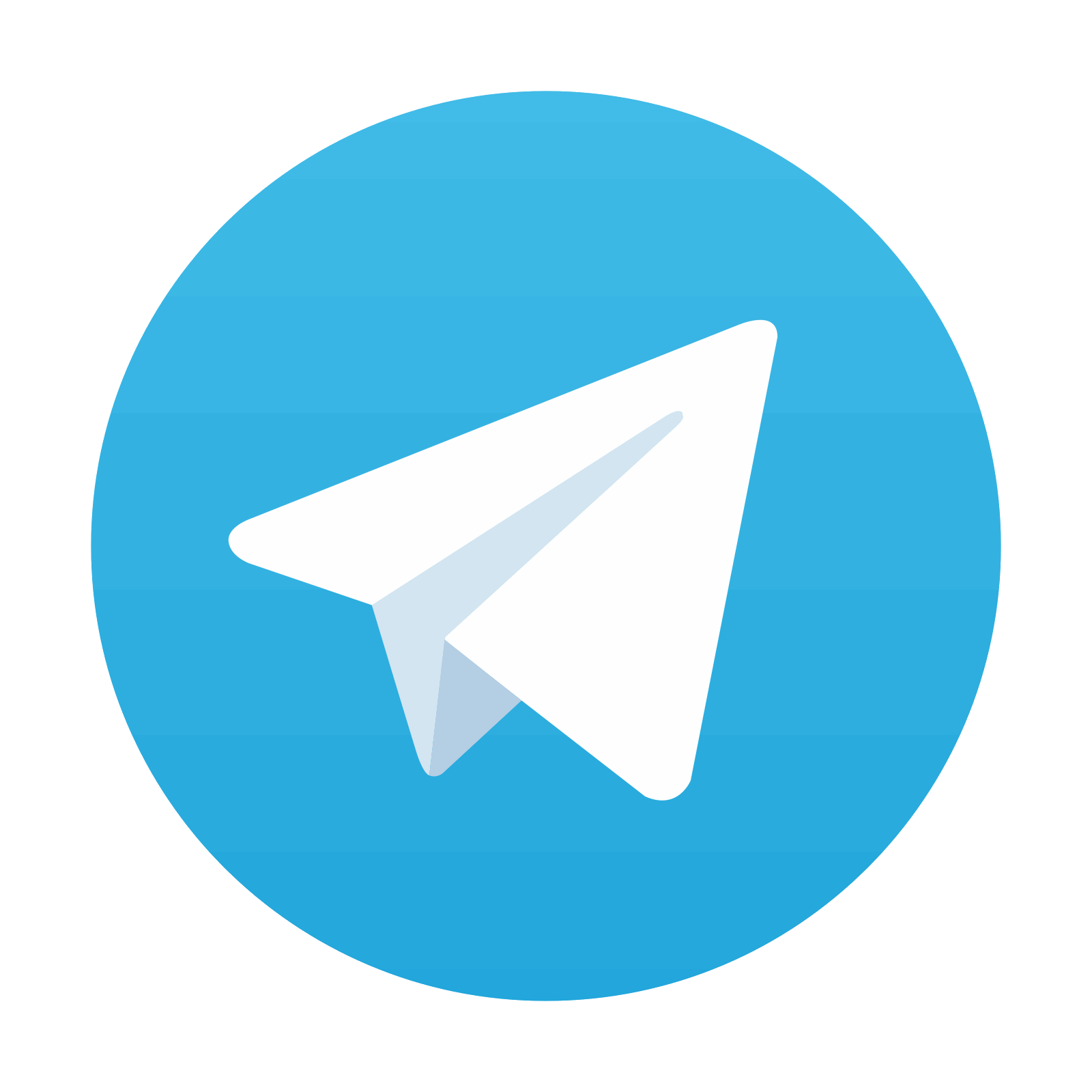
Stay updated, free articles. Join our Telegram channel

Full access? Get Clinical Tree
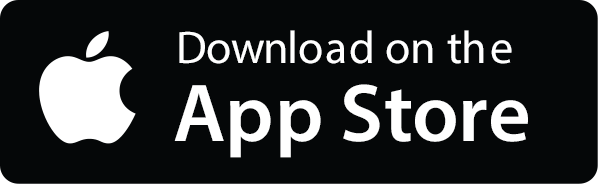
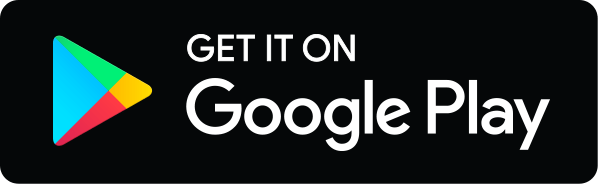