Chapter 12 FELINE DIABETES MELLITUS
The incidence of diabetes mellitus is similar for the dog and cat, with a reported frequency varying from 1 in 100 to 1 in 500 (Panciera et al, 1990). Historically, veterinarians have taken a similar approach to the diagnosis and treatment of diabetes mellitus in dogs and cats. However, research and clinical experience over the past two decades have made it obvious that there are clinically important differences between diabetic dogs and diabetic cats, and failure to recognize these differences can lead to confusion and frustration for the owner and the veterinarian. For example, cats, unlike dogs, often have a significant population of residual functional beta cells, which allows some cats to oscillate between an insulin-dependent and a non– insulin-dependent state and provides opportunities for the use of oral hypoglycemic drugs in some cats. Cats, unlike dogs, are extremely “sympathoadrenal” animals; that is, they appear to have a rapid catecholamine response during times of stress, excitement, fear, or aggression. As a consequence, the traditional reliance on blood glucose concentrations to monitor therapy is often unreliable in cats because of problems with stress-induced hyperglycemia. Cats, more so than dogs, are prone to the development of hypoglycemia and the Somogyi phenomenon at dosages of insulin assumed to be safe for the treatment of diabetes—an assumption that leads to persistent poor control of the diabetic state. Identifying an effective treatment regimen for diabetic cats can be challenging. Factors that affect the type and success of treatment include the severity of pancreatic beta cell loss, the responsiveness of tissues to insulin, the presence or absence of glucose toxicity, problems with absorption and duration of effect of exogenously administered insulin, and the presence of concurrent disease. This chapter focuses on specific aspects of diabetes as it relates to the cat. General information on various aspects of diabetes mellitus (e.g., classification of diabetes, overview of types of insulin) is provided in Chapter 11. Extensive cross-references have been provided in this chapter, where appropriate, to guide the reader to additional information on topics that are similar for the cat and dog.
CLASSIFICATION AND ETIOLOGY
Type 1 and type 2 diabetes mellitus
The etiologies of type 1 and type 2 diabetes mellitus are discussed on page 486. In humans, type 1 diabetes is characterized by a combination of genetic susceptibility and immunologic destruction of beta cells, with progressive and eventually complete insulin insufficiency. The presence of circulating autoantibodies against insulin, the beta cell, and/or glutamic acid decarboxylase (GAD) usually precedes the development of hyperglycemia or clinical signs. There is minimal evidence for the development of type 1 diabetes in cats. Lymphocytic infiltration of islets, in conjunction with islet amyloidosis and vacuolation, has been described in two diabetic cats (Nakayama et al, 1990), suggesting the possibility of immune-mediated insulitis. However, this histologic finding is very uncommon in diabetic cats. In addition, beta cell and insulin autoantibodies were not identified in 26 newly diagnosed, untreated diabetic cats (Hoenig et al, 2000a), findings that suggest that an immune-mediated process does not cause diabetes in most cats.
Insulin resistance and impaired insulin secretion have been identified in cats with obesity, and islet amyloid has been identified in insulin-dependent and non–insulin-dependent diabetes in cat (Yano et al, 1981a and 1981b; Nelson et al, 1990; Appleton et al, 2001); these findings are also associated with type 2 diabetes mellitus in humans. Low insulin sensitivity has also been identified in a group of lean cats subsequently identified to be at greater risk for developing impaired glucose tolerance with obesity, compared with lean cats with higher insulin sensitivity who subsequently developed obesity (Appleton et al, 2001). Although the role of genetics remains to be determined in cats, the findings of Appleton and colleagues suggest that similar etiologies exist for the development of type 2 diabetes in humans and in cats; that is, genetic predisposition, insulin resistance, impaired insulin secretion, islet amyloid, and environmental factors.
Just as in humans, diabetes is undoubtedly a multifactorial disease in cats. The severity of destruction of the pancreatic islets and the presence, severity, and reversibility of concurrent disorders that negatively affect insulin sensitivity are perhaps the two most important factors dictating the development of diabetes mellitus and the insulin dependency of the diabetic state in cats. Examples of concurrent insulin-resistant disorders include obesity, chronic pancreatitis and other chronic inflammatory diseases, and infection, and insulin-resistant diseases such as hyperthyroidism, hyperadrenocorticism, and acromegaly. Identification and correction of concurrent problems that affect insulin sensitivity are critical to the successful treatment of diabetes in cats. Improvement in insulin sensitivity may promote a non–insulin-dependent or transient diabetic state in cats with partial loss of pancreatic beta cells (see Transient Diabetes in Cats). As such, it is more clinically relevant and perhaps more accurate to classify diabetes in cats as insulin-dependent diabetes mellitus (IDDM) or non–insulin-dependent diabetes mellitus (NIDDM) rather than as type 1 or type 2 for reasons discussed on page 488.
Insulin-dependent diabetes in cats
The most common clinically recognized form of diabetes mellitus in the cat is IDDM. In our hospital, approximately 70% of cats have IDDM at the time diabetes mellitus is diagnosed. Cats with IDDM fail to respond to diet and oral hypoglycemic drugs and must be treated with exogenous insulin to obtain control of glycemia and prevent ketoacidosis. Common histologic abnormalities in cats with IDDM include islet-specific amyloidosis (see Non–insulin-dependent Diabetes in Cats), beta-cell vacuolation and degeneration, and chronic pancreatitis (Fig. 12-1) (O’Brien et al, 1986; Johnson et al, 1989; Goossens et al, 1998). The cause of beta-cell degeneration in cats is not yet known. Chronic pancreatitis occurs more commonly in diabetic cats than previously suspected. In one study, evidence of past or current pancreatitis was identified in 19 (51%) of 37 diabetic cats at necropsy (Goossens et al, 1998). Seemingly, pancreatitis may be responsible for islet destruction in some cats with IDDM. Still other diabetic cats do not have amyloidosis, inflammation, or degeneration of the pancreatic islets but have a reduction in the number of pancreatic islets and/or insulin-containing beta cells on immunohistochemical evaluation. This suggests that additional mechanisms are involved in the physiopathology of diabetes mellitus in cats. Immune-mediated destruction of the islets does not appear to play a significant role in the etiology of diabetes in cats (see discussion in previous section), and the role of genetics remains to be determined.
Non–insulin-dependent diabetes in cats
Clinical recognition of NIDDM is more frequent in the cat than the dog, accounting for approximately 30% of diabetic cats seen at our hospital. The etiopathogenesis of NIDDM is undoubtedly multifactorial. In humans, obesity, genetics, and islet amyloidosis are important factors in the development of NIDDM (Truglia et al, 1985; Johnson et al, 1989; Leahy, 1990). Similar factors appear to play a role in the development of diabetes mellitus in the cat. Our current understanding of the etiopathogenesis of diabetes in the cat suggests that the difference between IDDM and NIDDM is primarily a difference in the severity of loss of beta cells and the severity and reversibility of concurrent insulin resistance. Most cats with IDDM and NIDDM have islet amyloidosis, vacuolar degeneration of beta cells, or islet hypoplasia (Goossens et al, 1998; Nelson et al, 1998). The more severe the islet pathology, the more likely the cat will have IDDM, regardless of concurrent insulin resistance (Fig. 12-2). The less severe the islet pathology, the greater the role of concurrent insulin resistance in dictating whether the cat has IDDM or NIDDM. The more severe and the less reversible the cause of the insulin resistance, the more likely the cat with mild islet pathology will be insulin dependent, and vice versa. Fluctuations in the severity of insulin resistance, as occurs with chronic pancreatitis, can cause a cat with mild islet pathology to oscillate between IDDM and NIDDM as the severity of pancreatic inflammation waxes and wanes. Persistent insulin resistance can also worsen islet pathology and cause the diabetic cat to progress from NIDDM to IDDM as the progressive loss of beta cells leads to worsening insulin deficiency (see Fig. 12-2; Fig. 12-3) (Nelson et al, 1998; Hoenig et al, 2000b).
Amyloid is a common pathologic finding in the pancreatic islets of cats, with the severity of islet amyloid deposition increasing as the cat ages (Johnson et al, 1989; Lutz et al, 1994). Diabetic cats have significantly greater numbers of pancreatic islets with amyloid deposits and more extensive deposition of amyloid within islets than do nondiabetic cats, suggesting that diabetes mellitus and insular amyloid are causally related (Yano et al, 1981a and 1981b). Islet-amyloid polypeptide (IAPP), or amylin, is the principal constituent of amyloid isolated from the pancreatic tissue of humans with NIDDM and of adult cats with diabetes (Johnson et al, 1989). The amino acid sequences of human and feline IAPP are similar (Westermark et al, 1987a); immunoelectron microscopic studies have identified IAPP within beta-cell secretory granules in both cats and humans (Westermark et al, 1987b; Johnson et al, 1988); and amylin is co-secreted with insulin by the beta cell (Lutz and Rand, 1996). Stimulants of insulin secretion also stimulate the secretion of amylin. Amylin acts as a neuroendocrine hormone and has several glucoregulatory effects that collectively complement the actions of insulin in postprandial glucose control. These effects include a slowing of the rate at which nutrients are delivered from the stomach to the small intestine for absorption, suppression of nutrient-stimulated secretion of glucagon, and stimulation of satiety (Young et al, 1996; Gedulin et al, 1997). Although controversial, pharmacologic concentrations of amylin may induce insulin resistance (Johnson et al, 1990; Kassir et al, 1991). Chronic increased secretion of insulin and amylin, as occurs with obesity and other insulin-resistant states, results in aggregation and deposition of amylin in the islets as amyloid (see Fig. 12-2). IAPP-derived amyloid fibrils are cytotoxic and are associated with apoptotic cell death of islet cells and a resultant defect in insulin secretion (Lorenzo et al, 1994; O’Brien et al, 1995; Hiddinga and Eberhardt, 1999). If deposition of amyloid is progressive (e.g., persistent insulin-resistant states, such as obesity), islet cell destruction progresses and eventually leads to diabetes mellitus (Fig. 12-4). The severity of islet amyloidosis would determine in part whether the diabetic cat has IDDM or NIDDM (see Fig. 12-2, page 542). Total destruction of the islets results in IDDM and the need for insulin treatment for the rest of the cat’s life. Partial destruction of the islets may or may not result in clinically evident diabetes, insulin treatment may or may not be required to control glycemia, and transient diabetes may or may not develop once treatment is initiated. If amyloid deposition is progressive, the cat will progress from a subclinical diabetic state to NIDDM and ultimately to IDDM.
The presence and severity of insulin resistance is an important variable that influences the clinical picture in cats with partial destruction of pancreatic islets. Insulin resistance increases the demand for insulin secretion, a demand that may not be met in some cats with partial destruction of islets. The more severe the insulin resistance and the more severe the loss of islets, the more likely hyperglycemia will develop. Persistent hyperglycemia in turn can suppress function of the remaining beta cells, causing hypoinsulinemia and worsening hyperglycemia (see Transient Diabetes in Cats). A sustained demand for insulin secretion in response to insulin resistance can also lead to worsening islet pathology and a further reduction in the population of beta cells. Any chronic insulin-resistant disorder can have a deleterious impact on the population and function of beta cells and can play a role in the development of NIDDM or IDDM. Examples in the cat include obesity, chronic pancreatitis, acromegaly, hyperadrenocorticism, and chronic administration of glucocorticoids or megestrol acetate. Obesity-induced carbohydrate intolerance is the classic insulin-resistant disorder affiliated with the development of NIDDM in humans and has been identified as a potential causative factor in the development of diabetes in cats as well (Panciera et al, 1990; Scarlett and Donoghue, 1998). Obesity causes a reversible insulin resistance that is a result of downregulation of insulin receptors, impaired receptor binding affinity for insulin, and postreceptor defects in insulin action (Truglia et al, 1985). Impaired glucose tolerance and an abnormal insulin secretory response have been documented in obese cats (Nelson et al, 1990; Appleton et al, 2001). This abnormal response is characterized by an initial delay in insulin secretion, followed by excessive insulin secretion (Fig. 12-5), results similar to those identified in humans with NIDDM. The abnormalities responsible for insulin resistance are reversible with correction of obesity, which is why correction and prevention of obesity is an important component of the treatment regimen for diabetes (Fig. 12-6) (Biourge et al, 1997; Fettman et al, 1998). With weight loss, insulin becomes more effective in controlling glycemia, and some diabetic cats revert to a non–insulin-dependent, subclinical diabetic state.
Leptin is an adipocyte-specific hormone that functions as an “adipostat” to sense and regulate body energy stores primarily by modulating the expression of hypothalamic neuropeptides known to regulate feeding behavior and body weight (Havel, 2001). A positive relationship between serum leptin immunoreactivity and body fat content has been identified in several species, including cats and dogs (Backus et al, 2000; Appleton et al, 2000; Sagawa et al, 2002). High leptin concentrations in the presence of obesity suggest that obese animals are resistant to leptin. A causal relationship between leptin and the insulin resistance of obesity has been suggested by some researchers (Segal et al, 1996; Zimmet et al, 1998). An inverse relationship between leptin concentrations and insulin sensitivity was recently reported in cats undergoing glucose tolerance testing before and after gaining weight, suggesting that increased leptin concentrations may contribute to the diminished insulin sensitivity observed in obese cats (Appleton et al, 2002a). However, insulin is an important stimulant for leptin production, and it is also possible that the compensatory hyperinsulinemia found with insulin resistance in obese cats stimulated leptin production (Havel, 2000).
Adiponectin is another adipocyte-specific hormone that has been proposed to be the link between obesity and insulin resistance. Adiponectin is believed to have an important role in the regulation of insulin action and energy homeostasis (Havel, 2002). Adiponectin decreases hepatic glucose production and increases glucose utilization by muscle (Berg et al, 2001; Fruebis et al, 2001). An inverse relationship between plasma adiponectin and leptin concentrations has been identified in normal-weight and obese humans, circulating concentrations of adiponectin are reduced in obese humans, and adiponectin is negatively correlated with fasting insulin concentrations and positively correlated with insulin sensitivity (Weyer et al, 2001). Finally, a decline in circulating adiponectin coincides with the onset of decreased insulin sensitivity, insulin resistance, and onset of type 2 diabetes mellitus in monkeys (Hotta et al, 2001).
Transient diabetes in cats
Approximately 20% of diabetic cats become “transiently diabetic,” usually within 4 to 6 weeks of establishment of the diagnosis of diabetes and initiation of treatment. In these cats, hyperglycemia, glycosuria, and clinical signs of diabetes resolve, and insulin treatment can be discontinued. Some diabetic cats may never require insulin treatment once the initial bout of clinical diabetes mellitus has dissipated, whereas others become permanently insulin dependent weeks to months after resolution of a prior diabetic state. Based on findings from a recent evaluation of a group of cats with transient diabetes mellitus, we theorize that cats with transient diabetes are in a subclinical diabetic state that becomes clinical when the pancreas is stressed by exposure to a concurrent insulin-antagonistic drug or disease, most notably glucocorticoids, megestrol acetate, and chronic pancreatitis (Fig. 12-7) (Nelson et al, 1998). Unlike healthy cats, those with transient diabetes mellitus have some abnormality of the islets (e.g., amyloidosis, vacuolar degeneration) and a significant reduction in the population of beta cells compared with healthy cats, which impairs their ability to compensate for concurrent insulin resistance and results in carbohydrate intolerance. Insulin secretion by beta cells becomes reversibly suppressed, most likely stemming from a worsening carbohydrate intolerance. Chronic hyperglycemia impairs insulin secretion by beta cells and induces peripheral insulin resistance by promoting the downregulation of glucose transport systems and causing a defect in posttransport insulin action; this phenomenon is referred to as glucose toxicity (Unger and Grundy, 1985; Rossetti et al, 1990). Beta cells have an impaired response to stimulation by insulin secretagogues, thereby mimicking IDDM (Fig. 12-8). The effects of glucose toxicity are potentially reversible upon correction of the hyperglycemic state. The clinician makes a correct diagnosis of diabetes and initiates appropriate treatment for diabetes and identifiable insulin-antagonistic disorders. Treatment improves hyperglycemia and insulin resistance, the suppressive effects of hyperglycemia decrease, beta-cell function improves, insulin secretion returns, and an apparent IDDM state resolves (see Figs. 12-7 and 12-8). The future requirement for insulin treatment depends on the underlying abnormality in the islets. If the islet pathology is progressive (e.g., amyloidosis), eventually enough beta cells are destroyed that permanent IDDM results.
Diagnosis of IDDM versus NIDDM
Cats do not typically present to the clinician until clinical signs of diabetes become obvious and worrisome to an owner. Therefore at the time of diagnosis, all diabetic cats have fasting hyperglycemia and glycosuria, regardless of the type of diabetes mellitus present. Once the diagnosis of diabetes has been established, the clinician must consider the possibility of NIDDM. The significant incidence of NIDDM and transient diabetes in cats raises interesting questions concerning the need for insulin treatment. Glycemic control can be maintained in some diabetic cats with dietary changes and/or oral hypoglycemic drugs. Obviously, it would be advantageous to be able to prospectively differentiate IDDM from NIDDM. Insulin secretagogue tests have been used in humans for this purpose (Table 12-1). Unfortunately, measurements of the serum insulin concentration at baseline and after administration of an insulin secretagogue have not been consistent aids in differentiating IDDM and NIDDM in the cat (Nelson et al, 1993; Kirk et al, 1993). A fasting serum insulin concentration or any postsecretagogue insulin concentration greater than one standard deviation above the reference mean (>18 μU/ml in our laboratory) suggests the existence of functional beta cells and the possibility of NIDDM. Unfortunately, cats subsequently identified as having IDDM and many of those with NIDDM have a low baseline serum insulin concentration and do not respond to a glucose or glucagon challenge (Nelson et al, 1993; Kirk et al, 1993; Nelson et al, 1998). This apparent insulin deficiency in cats subsequently identified with NIDDM is presumably the result of concurrent glucose toxicity (see Transient Diabetes in Cats). Because of problems with insulin-secretagogue tests in identifying beta-cell function, the ultimate differentiation between IDDM and NIDDM often is made retrospectively, after the clinician has had several weeks to assess the cat’s response to therapy and to determine the animal’s need for insulin. The initial decision between insulin treatment and oral hypoglycemic drugs is based on the severity of clinical signs, the presence or absence of ketoacidosis, the cat’s general health, and the owner’s wishes.
TABLE 12-1 PROTOCOLS FOR INSULIN SECRETORY RESPONSE TESTS
Intravenous Glucose Tolerance Test (IVGTT) 2. Place indwelling catheter into jugular or medial saphenous vein to minimize stress with multiple blood samplings. Allow 24-hour recovery before performing the IVGTT. 5. Collect baseline blood samples for blood glucose and insulin concentration 5 to 15 minutes before and immediately prior to glucose administration. 6. Administer 0.5 g (dog) or 1.0 g (cat) of 50% dextrose per kilogram of body weight IV over 30 seconds. Do not use the same catheter for glucose administration and blood sampling. |
Intravenous Glucagon Tolerance Test |
Steps 1 to 5: As in IVGTT. |
Oral Glucose Tolerance Test |
Steps 1 to 5: As in IVGTT. |
Intravenous Arginine Tolerance Test |
Steps 1 to 5: As in IVGTT. 6. Administer 0.1 g of L-arginine hydrochloride per kilogram of body weight (cat) IV over 30 seconds. |
PATHOPHYSIOLOGY
The pathophysiology of diabetes mellitus is similar for the dog and cat and is discussed in Chapter 11. The only significant difference between dogs and cats appears to be a greater variability in the renal tubular threshold for excretion of glucose in the urine in cats. Spillage of glucose into the urine occurs when the blood glucose concentration exceeds 180 to 220 mg/dl in the dog. The reported mean threshold for healthy cats is 290 mg/dl. Subjectively, diabetic cats appear to have thresholds for glucose ranging from 200 to 300 mg/dl. As such, the correlation between the blood glucose concentration and the onset of clinical signs is more variable in cats than dogs; that is, some cats are asymptomatic at blood glucose concentrations that would cause clinical signs in dogs.
SIGNALMENT
Although diabetes mellitus may be diagnosed in cats of any age, most diabetic cats are older than 6 years of age (mean, 10 years) at the time of diagnosis. Diabetes mellitus occurs predominantly in neutered male cats, and there is no apparent breed predisposition, although Burmese cats may be overrepresented in Australia (Panciera et al, 1990; Rand et al, 1997).
ANAMNESIS
The history in diabetic dogs and cats is similar and is discussed in Chapter 11. A common complaint of cat owners is the constant need to change the litter and an increase in the size of the “kitty litter clumps,” problems that reflect the polyuria associated with diabetes mellitus. Additional clinical signs include lethargy; decreased interaction with family members; lack of grooming behavior and the development of a dry, lusterless, unkempt or matted haircoat; and decreased jumping ability, rear limb weakness, or the development of a plantigrade posture (Fig. 12-9). If the clinical signs associated with uncomplicated diabetes are not observed by the owner, a diabetic cat may be at risk for the development of systemic signs of illness as progressive ketonemia and metabolic acidosis develop (see Chapter 13, page 584). The time sequence from the onset of initial clinical signs to the development of diabetic ketoacidosis (DKA) is unpredictable.
PHYSICAL EXAMINATION
The physical examination findings depend on whether DKA is present and its severity and on the nature of any other concurrent disorder. The nonketotic diabetic cat has no classic physical examination findings. Many diabetic cats are obese but are otherwise in good physical condition. Cats with prolonged untreated diabetes may have lost weight but are rarely emaciated unless concurrent disease (e.g., hyperthyroidism) is present. Newly diagnosed and poorly controlled diabetic cats often stop grooming and develop a dry, lusterless haircoat. Diabetes-induced hepatic lipidosis may cause hepatomegaly. Impaired ability to jump, weakness in the rear limbs, ataxia, or a plantigrade posture (i.e., the hocks touch the ground when the cat walks) may be evident if the cat has developed diabetic neuropathy. Distal muscles of the rear limbs may feel hard on digital palpation, and cats may object to palpation or manipulation of the rear limbs or feet, presumably because of pain associated with the neuropathy. Additional abnormalities may be identified in the ketoacidotic diabetic cat (see Chapter 13, page 584).
ESTABLISHING THE DIAGNOSIS OF DIABETES MELLITUS
Establishment of the diagnosis of diabetes mellitus is similar for cats and dogs and is based on identification of appropriate clinical signs, hyperglycemia, and glycosuria (see page 493). Transient, stress-induced hyperglycemia is a common problem in cats and can cause the blood glucose concentration to increase above 300 mg/dl (see page 567). Unfortunately, stress is a subjective state that cannot be accurately measured, it is not always easily recognized, and it may evoke inconsistent responses among individual cats. Glycosuria usually does not develop in cats with stress hyperglycemia because the transient increase in the blood glucose concentration prevents glucose from accumulating in urine to a detectable concentration. For this reason, the clinician should always document persistent hyperglycemia and glycosuria when establishing a diagnosis of diabetes mellitus in cats. If the practitioner is in doubt, the “stressed” cat can be sent home with instructions for the owner to monitor the urine glucose concentration with the cat in the nonstressed home environment. Alternatively, a serum fructosamine concentration can be measured (see page 562). Documentation of an increase in the serum fructosamine concentration supports the presence of sustained hyperglycemia; however, a serum fructosamine concentration in the upper range of normal can occur in symptomatic diabetic cats if the diabetes developed shortly before presentation of the cat to the veterinarian (Elliott et al, 1999). Once the diagnosis of diabetes mellitus has been established, differentiation between NIDDM and IDDM can be considered (see Diagnosis of IDDM versus NIDDM).
CLINICAL PATHOLOGIC ABNORMALITIES
Information used to establish the diagnosis of diabetes mellitus does not provide information on the status of pancreatic islet health, the presence of glucose toxicity, the cat’s ability to secrete insulin, or the severity and reversibility of concurrent insulin resistance. Identification of a serum insulin concentration greater than 18 μU/ml in a newly diagnosed, untreated diabetic cat supports the presence of functional beta cells and partial destruction of the islets; however, low or undetectable serum insulin concentrations do not rule out partial islet cell loss because of the suppressive effects of hyperglycemia on circulating insulin concentrations (Kirk et al, 1993; Nelson et al, 1998). Simply establishing the diagnosis of diabetes does not provide the whole picture; a thorough evaluation for concurrent disorders that may affect insulin sensitivity of tissues and evaluation of response to treatment are important pieces of the puzzle when trying to determine if the diabetic cat has IDDM, NIDDM, or transient diabetes mellitus. The minimum laboratory evaluation in any diabetic cat should include a complete blood count (CBC), serum biochemical panel, serum thyroxine concentration, and urinalysis with bacterial culture. If available, abdominal ultrasound should be a routine part of the diagnostic evaluation because of the high prevalence of chronic pancreatitis in diabetic cats. Measurement of the baseline serum insulin concentration or an insulin response test is not routinely done in cats because of the problems encountered with glucose toxicity. Additional tests may be warranted after the clinician has obtained the history, performed the physical examination, or identified ketoacidosis.
Clinical pathologic abnormalities are similar for diabetic dogs and cats and are discussed on page 493 and listed in Table 11-5, page 494. Of special note in the cat is assessment of thyroid gland function, the health of the exocrine pancreas, and concerns regarding radioimmunoassays for measuring the serum insulin concentration.
TABLE 12-5 ORAL SULFONYLUREA DRUGS AVAILABLE IN THE UNITED STATES
Generic Name | Trade Name | Relative Potency |
---|---|---|
First Generation | ||
Tolbutamide | Ornase | 1 |
Acetohexamide | Dymelor | 2.5 |
Tolazamide | Tolinase | 5 |
Chlorpropamide | Diabinese | 6 |
Second Generation | ||
Glipizide | Glucotrol | 100 |
Glyburide | Micronase, DiaBeta | 150 |
Serum thyroxine concentration
The serum thyroxine (T4) concentration should be evaluated in all geriatric diabetic cats, in part because hyperthyroidism is common in older cats, small thyroid nodules (usually nonfunctional) are often palpable in older diabetic cats, and hyperthyroidism can cause insulin resistance (Hoenig and Ferguson, 1988; Hoenig et al, 1992). When interpreting serum T4 concentrations in diabetic cats, the veterinarian must consider the current status of glycemic control and the severity of any concurrent illnesses. Poorly regulated diabetic cats, especially those with concurrent disorders, typically have falsely lowered serum T4 concentrations, presumably as a result of the euthyroid sick syndrome. As a general rule, increased serum T4 concentrations (i.e., >4 μg/dl) support hyperthyroidism, and serum T4 concentrations in the lower half of the normal range or below the normal range (i.e., <2.0 μg/dl) support euthyroidism or the euthyroid sick syndrome, respectively. Serum T4 concentrations in the upper half of the normal range (i.e., 2.0 to 4.0 μg/dl) are difficult to interpret because of the potentially suppressive effects of the uncontrolled diabetic state on the pituitary-thyroid axis. Serum T4 results in this range are consistent with euthyroidism or mild hyperthyroidism with concurrent suppression of serum T4 concentration into the upper normal range. Additional diagnostic tests (e.g., serum free T4 concentration, sodium pertechnetate thyroid scan) may be warranted to determine the functional status of the thyroid gland. Chapter 4 presents a more detailed discussion of the interpretation of serum T4 concentrations in cats suspected of having hyperthyroidism.
Pancreatic enzymes
Acute and especially chronic pancreatitis are common concurrent disorders in diabetic cats. Blood tests used to assess for the presence of pancreatitis should always be considered in the newly diagnosed diabetic cat, especially if abdominal ultrasound is not available. Measurement of the serum lipase concentration and serum feline trypsin-like immunoreactivity (fTLI) are most commonly recommended. In theory, cats with concomitant active pancreatitis should have an increase in the serum lipase concentration and serum fTLI. Unfortunately, serum lipase concentrations and serum fTLI do not always correlate accurately with the presence or absence of pancreatitis, especially when the inflammatory process is chronic and mild (Swift et al, 2000; Gerhardt et al, 2001). Interpretation of serum lipase and fTLI results should always be done in context with the history, physical examination findings, and additional findings on the laboratory tests. In our hospital and using sophisticated ultrasonographic equipment, abdominal ultrasound is the single best diagnostic test for identifying pancreatitis in the cat (Fig. 12-10). As such, abdominal ultrasound is part of the routine diagnostic evaluation of any newly diagnosed or poorly controlled diabetic cat. The concomitant presence of acute pancreatitis in a newly diagnosed diabetic cat may necessitate the instigation of intensive fluid therapy and modifications in the diet that may otherwise not have been done. Identification of chronic pancreatitis also has important prognostic implications regarding the success of establishing and maintaining control of glycemia and of long-term survival (see page 528).
Measurement of serum fTLI is also used to diagnose exocrine pancreatic insufficiency, an uncommon complication of diabetes mellitus that presumably develops as a sequela of chronic pancreatitis (Steiner and Williams, 2000). Exocrine pancreatic insufficiency should be suspected in diabetic cats that are difficult to regulate with insulin and are thin or emaciated despite polyphagia (see page 528).
Serum insulin concentration
Measurement of the serum insulin concentration, either baseline or after the administration of an insulin secretagogue, is not a routine part of our diagnostic evaluation of the newly diagnosed diabetic cat. In theory, identification of increased endogenous serum insulin concentrations (i.e., >18 μU/ml) in a newly diagnosed diabetic cat would suggest a likely response to administration of oral hypoglycemic drugs and the possibility for transient diabetes mellitus, especially if an underlying insulin antagonistic disorder can be identified and treated. Unfortunately, the suppressive effects of hyperglycemia on beta-cell function (i.e., glucose toxicity) often cause low serum insulin concentrations in animals subsequently identified as having NIDDM (see Transient Diabetes in Cats) (Nelson et al, 1998). Although increased serum insulin concentrations suggest the existence of functional beta cells, finding low serum insulin concentrations (i.e., <12 μU/ml) does not rule out the existence of functional beta cells. Because the serum insulin concentration is low in most cats subsequently found to have NIDDM, routine measurement of the serum insulin concentration is not a cost-effective diagnostic procedure.
It is imperative that the radioimmunoassay (RIA) for insulin be validated for the cat. Commercial endocrine laboratories use RIAs designed for use in humans. Unfortunately, the amino acid sequences of human and cat insulins are different (see Table 11-6, page 496), and many RIAs designed for measurement of the serum insulin concentration in humans do not work in cats (Lutz and Rand, 1993). Interestingly, studies evaluating the pharmacokinetic properties of exogenously administered beef, pork, or recombinant human insulin in cats have used RIAs that do not detect cat insulin to ensure lack of interference of the cat’s insulin in the results (Wallace et al, 1990).
TABLE 12-6 ADVERSE REACTIONS TO GLIPIZIDE TREATMENT IN DIABETIC CATS
Adverse Reaction | Recommendation |
---|---|
Vomiting within 1 hour of administration | Vomiting usually subsides after 2 to 5 days of glipizide therapy; decrease dose or frequency of administration if vomiting is severe; discontinue if vomiting persists longer than 1 week. |
Increased serum hepatic enzyme activities | Continue treatment and monitor enzymes every 1 to 2 weeks initially; discontinue glipizide if cat becomes ill (lethargy, inappetence, vomiting) or the alanine transaminase activity exceeds 500 IU/L. |
Icterus | Discontinue glipizide treatment; reinstitute glipizide therapy at lower dose and frequency of administration once icterus has resolved (usually within 2 weeks); discontinue treatment permanently if icterus recurs. |
Hypoglycemia | Discontinue glipizide treatment; recheck blood glucose concentration in 1 week; reinstitute glipizide therapy at lower dose or frequency of administration if hyperglycemia recurs. |
TREATMENT OF NONKETOTIC DIABETES MELLITUS
Goals of Therapy
The goals of therapy are similar for the diabetic dog and cat; that is, elimination of owner-observed signs that occur secondary to hyperglycemia and glycosuria, maintenance of a “healthy” pet at a stable body weight, and avoidance of complications affiliated with diabetes mellitus and its treatment (see Table 11-7, page 496). These goals can usually be attained through proper insulin administration, diet, exercise, oral hypoglycemic medications, and/or the avoidance or control of concurrent inflammatory, infectious, neoplastic, and hormonal disorders. Which therapeutic regimen is ultimately successful depends in part on the number of functional beta cells remaining in the pancreas and individual variation of response to treatment.
TABLE 12-7 SAMPLE HANDLING, METHODOLOGY, AND NORMAL VALUES FOR SERUM FRUCTOSAMINE CONCENTRATIONS MEASURED IN OUR LABORATORY IN CATS
Parameter | Pertinent Information | |
---|---|---|
Blood sample | 1 to 2 ml; allow to clot, obtain serum | |
Sample handling | Freeze until assayed | |
Methodology | Automated colorimetric assay using nitroblue tetrazolium chloride | |
Factors affecting results | Hypoproteinemia and hypoalbuminemia (decreased), storage at room temperature (decreased) | |
Normal range | 190 to 365 μmol/L | |
Interpretation in diabetic cats | ||
Excellent control | 350 to 400 μmol/L | |
Good control | 400 to 450 μmol/L | |
Fair control | 450 to 500 μmol/L | |
Poor control | >500 μmol/L | |
Prolonged hypoglycemia | <300 μmol/L |
Initial Insulin Therapy
The types and species of commercial insulin preparations are discussed in Chapter 11 (page 496). Diabetic cats are notoriously unpredictable in their response to exogenous insulin. No single type of insulin is routinely effective in maintaining control of glycemia, even with twice a day administration. Since the loss of protamine zinc insulin (PZI) a decade ago, neutral protamine Hagedorn (NPH), lente, and ultralente insulin of recombinant human origin have all been used to treat diabetic cats. Ultralente insulin is the longest acting but least potent of the commonly used commercial insulins (see Fig. 11-19, page 521, and Fig. 12-11). Although considered a long-acting insulin, ultralente insulin has to be administered twice a day in most diabetic cats, and absorption of ultralente insulin is inadequate for controlling glycemia in approximately 25% of cats. Lente and NPH are more potent insulin preparations that are more consistently and rapidly absorbed after subcutaneous administration than is ultralente insulin. Unfortunately, the duration of effect of lente and especially NPH insulin can be considerably shorter than 12 hours in some diabetic cats, resulting in inadequate control of glycemia despite twice a day administration (see Table 11-8, page 497). Although the amino acid sequences of human and feline insulin differ, the prevalence of insulin antibodies causing problems with control of glycemia is uncommon in cats treated with recombinant human insulin (see page 572).
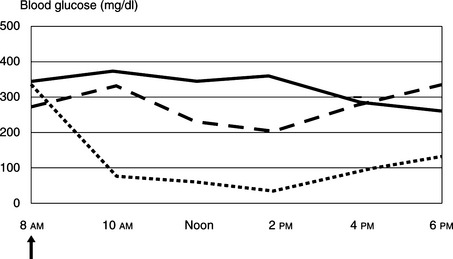
FIGURE 12-19 Initial regulation of insulin-dependent diabetes mellitus in a 6.5 kg Maine Coon cat using blood glucose measurements determined by the owner at home and using the marginal ear vein prick technique described in Figure 12-18. The cat initially was treated with 2 U of PZI administered twice daily. The cat remained symptomatic, and the blood glucose concentrations were still too high at 3 U (solid line) and 5 U (dashed line) of PZI twice daily. Clinical signs resolved and control of glycemia was attained with 6 U (dotted line) of PZI administered twice daily. The arrow indicates insulin administration and fed half of total daily caloric intake.
TABLE 12-8 SAMPLE HANDLING, METHODOLOGY, AND NORMAL VALUES FOR BLOOD TOTAL GLYCOSYLATED HEMOGLOBIN CONCENTRATIONS MEASURED IN OUR LABORATORY IN CATS
Parameter | Pertinent Information | |
---|---|---|
Blood sample | 1 to 2 ml whole blood in EDTA | |
Sample handling | Refrigerate until assayed | |
Methodology | Affinity chromatography and hemolysates derived from feline red blood cells | |
Factors affecting results | Storage at room temperature (decreased), storage at 4° C for longer than 7 days (decreased); anemia (Hct <35%) (decreased) | |
Normal range | 0.9 to 2.5% | |
Interpretation in diabetic cats | ||
Excellent control | 1.0 to 2.0% | |
Good control | 2.0 to 2.5% | |
Fair control | 2.5 to 3.0% | |
Poor control | >3.0% | |
Prolonged hypoglycemia | <1.0% |
EDTA, Ethylenediamine tetra-acetic acid; Hct, hematocrit.
PZI of beef/pork origin at a concentration of 40 U/ml (i.e., U40) and produced using the same methods as the original manufacturer is now available (IDEXX, Westbrook, Maine). PZI is a longer acting insulin that is more consistently absorbed than ultralente insulin and has a more acceptable duration of effect than NPH insulin. However, the timing of the glucose nadir is quite variable and occurs within 9 hours of PZI administration in greater than 80% of treated diabetic cats (Nelson et al, 2001). We routinely administer PZI twice a day. In a recent study, PZI was very effective in significantly improving control of glycemia in newly diagnosed diabetic cats and poorly controlled diabetic cats previously treated with ultralente or NPH insulin (Fig. 12-12) (Nelson et al, 2001). Comparison of efficacy between PZI and lente has not been reported. PZI is not indicated for diabetic dogs because of the high probability of insulin antibody production directed against the bovine insulin in the product (see page 522). Insulin antibodies may interfere with insulin action, causing erratic and poor control of glycemia.
Insulin glargine (Lantus; Aventis Pharmaceuticals, Bridgewater, NJ) is a long-acting insulin analog that forms microprecipitates at the site of injection, from which small amounts of insulin glargine are slowly released (see page 498). In humans, the slow, sustained release of insulin glargine from these microprecipitates results in a relatively constant concentration/time profile over a 24-hour period with no pronounced peak in serum insulin. Insulin glargine is currently recommended as a basal insulin (i.e., sustained, long-acting insulin to inhibit hepatic glucose production) administered once a day at bedtime in human diabetics (Rosenstock et al, 2000 and 2001). In a preliminary study involving healthy cats, most of the pharmacokinetic and pharmacodynamic properties (i.e., onset of action, glucose nadir, time for blood glucose concentration to return to baseline, mean daily blood glucose concentration, and area under the 24-hour blood glucose curve) were similar for insulin glargine and PZI (Marshall and Rand, 2002). Similar studies in diabetic cats have yet to be reported. In our experience, insulin glargine has a duration of effect ranging from 10 to 16 hours in most diabetic cats. We have not yet encountered problems with inadequate absorption of insulin glargine, as is described with ultralente insulin, although it seems likely that this problem will be encountered as we gain more experience with this insulin analog. Currently, we consider using insulin glargine in diabetic cats with problems of short duration of effect from NPH, lente, and PZI (see page 570). Based on our current experiences, we do not consider insulin glargine a first-choice insulin for the treatment of diabetes in cats.
It is impossible to predict which type of insulin will work best in individual diabetic cats. The initial insulin of choice ultimately is based on personal preference and experience. Currently, we recommend either lente insulin of recombinant human origin or PZI of beef/pork origin at a dosage of 1 U per cat administered twice daily. Dietary therapy is initiated concurrently (see below). Because greater than 80% to 90% of diabetic cats require recombinant human lente or beef/pork PZI twice a day, we prefer to start with twice a day insulin therapy. Establishing control of glycemia is easier and problems with hypoglycemia and the Somogyi effect (see page 569) are less likely when twice daily insulin therapy is initiated while the insulin dose is low; that is, when insulin treatment is started.
Dietary Therapy
The general principles for dietary therapy are discussed on page 499 (Table 12-2). Obesity, feeding practices, and content of the diet warrant discussion in diabetic cats.
TABLE 12-2 RECOMMENDATIONS FOR DIETARY TREATMENT OF DIABETES MELLITUS IN CATS
Obesity
Obesity is common in diabetic cats and results from excessive caloric intake, typically caused by free-choice feeding of dry cat food. Obesity causes reversible insulin resistance, which resolves as obesity is corrected (see Fig. 12-6) (see page 545) (Nelson et al, 1990; Biourge et al, 1997). Control of glycemia often improves and some diabetic cats may revert to a subclinical diabetic state with weight reduction. The general principles for correcting obesity are similar for dogs and cats and are discussed on page 501. Correction of obesity is difficult in cats because it requires restriction of daily caloric intake with a minimal corresponding increase in caloric expenditure (i.e., exercise). The optimum body weight for cats is generally 3.5 to 5 kg. It is very important to set realistic and obtainable goals for weight loss in order to maintain client compliance. If the ideal body weight is below 15% of the current body weight, it is crucial to use a stepwise process to gradually achieve ideal body weight. The pet’s initial goal should be set at 15% body weight loss. Once this goal has been achieved, a new target body weight can be selected until the pet has reached an ideal body weight. To achieve 15% body weight loss, cats can be fed 30 × (initial body weight in kg) kcal per day (Elliott, 2003). When fed at this level, cats achieve 15% body weight loss in 18 weeks. If the calculated amount of calories to achieve 15% body weight loss is actually less than the current daily caloric intake, the dietary history should be reevaluated to search for additional calories. If no additional daily calories are identified, the daily caloric intake of the pet should be reduced by 15% to 20%. Although several diets specifically formulated for weight reduction in cats are available, diets containing increased amounts of fiber or protein should be used in the diabetic cat for reasons discussed below.
Multicat households in which one cat is obese but the others are normal body weight or lean can present some management problems. Ideally cats should be fed in separate rooms, but this is not always possible. In general, fat cats cannot jump. Hence, it may be useful to place the healthy cat’s food on an elevated bench so that healthy cats can jump up to consume their meals. Alternatively, a large cardboard box can be obtained. A small cat hole is cut into the box that will allow the normal body weight cats to fit but that restricts the entry of the fat cat. The normal weight cats are then fed in the box (Elliott, 2003).
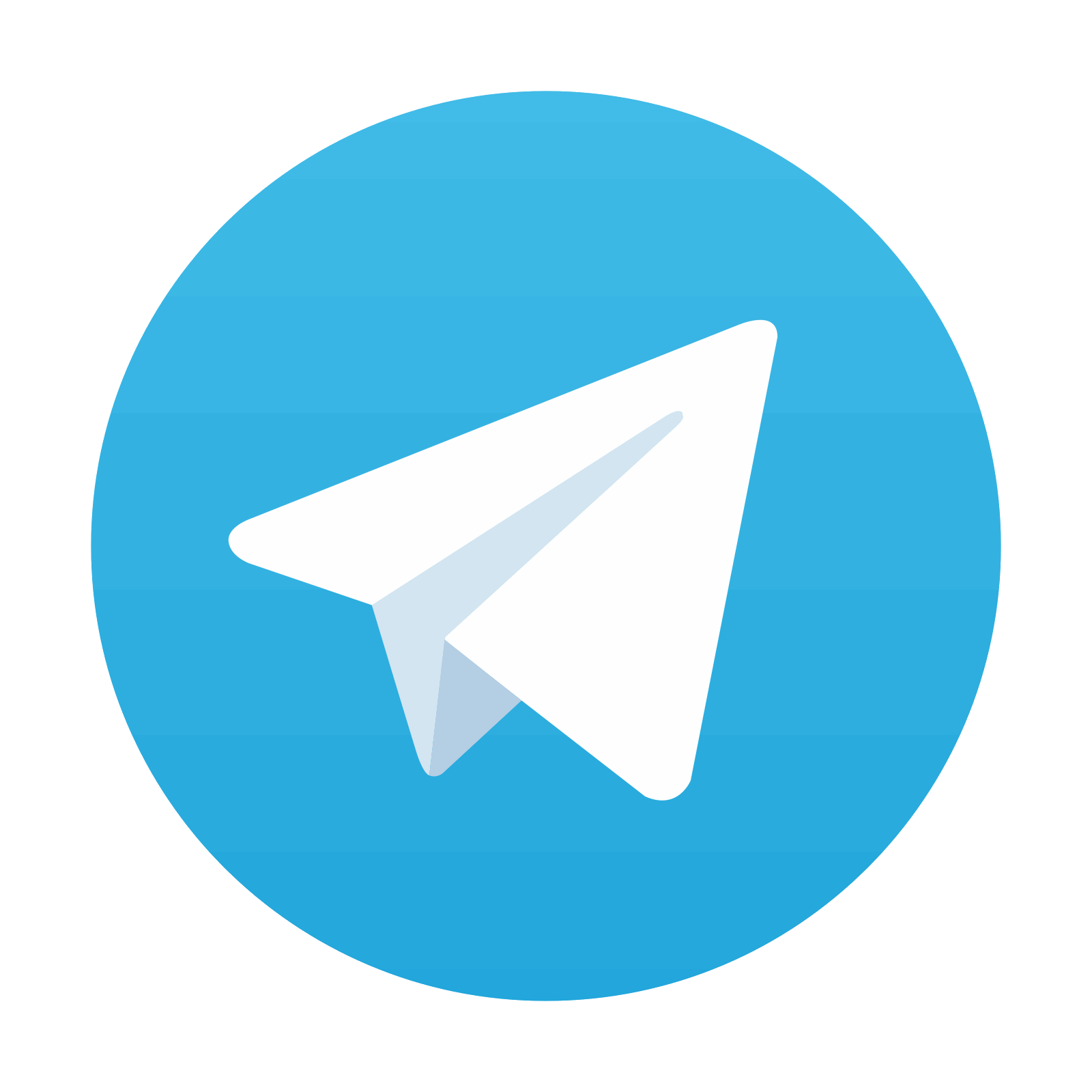
Stay updated, free articles. Join our Telegram channel

Full access? Get Clinical Tree
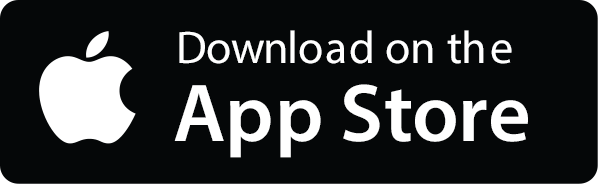
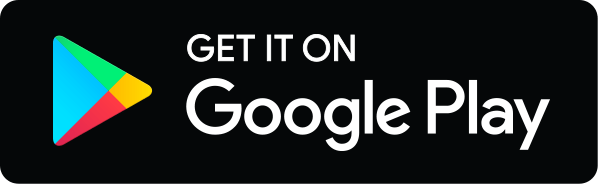