Chapter 44 External skeletal fixators were first described in 1897 by Parkhill, who designed and used a special external clamp to engage transfixation pins placed into long bones to externally stabilize the fracture. Later, during World War II, external skeletal fixation was brought into general medical use in the battlefield hospital environment to stabilize fractures in injured military field personnel. Unfortunately, a high incidence of major complications such as premature pin loosening, pin tract sepsis, and associated delayed union or nonunion fracture was commonly observed in these patients. As a result, external skeletal fixators were used on a limited basis in human orthopedics from the late 1940s through the 1970s.5,90 After World War II, the development of proper transfixation pin insertion techniques and advancements in pin design resulted in enhanced longevity of the pin-bone interface, which resulted in fewer complications than experienced with earlier external skeletal fixation technology. Further development of new materials used to create new clamp and connecting bar designs increased the structural integrity of an external skeletal fixator frame as well. Advanced biomechanical analysis of external skeletal fixator frame configurations in the mid-1980s led to enhanced knowledge of how different geometric configurations could provide predicted mechanical support in clinical veterinary patients with long bone fractures.16 Further discoveries of advanced mechanical properties using hybrid frames (e.g., type Ia/type IIb, linear/circular) and tie-ins (additional connecting bars to transfixation pins62 or directly to intramedullary pins)6,95 have enhanced the use of external skeletal fixation for complex fractures of the humerus and femur. As a result of these developments, external skeletal fixation has seen resurgence in use over the past 30 years and has become a standard of care in both human and veterinary orthopedics. External skeletal fixation uses pins or Kirschner wires to transfix bone fragments. Each pin or wire is then functionally anchored to a connecting bar by a pin-connecting bar clamp, to a connecting ring by bolts, or connected without clamps using acrylic. The combination of pins, clamps, and connecting bars create the external skeletal fixator frame (the entire apparatus) (Figure 44-1). Collectively, the frame and the transfixed bone are referred to as a construct or montage. Attempts have been made to standardize the nomenclature used to describe external skeletal fixator frame designs (especially linear systems) and associated mechanical strength resulting from the selected frame geometry and components used to create the fixator. Linear external skeletal fixation systems are created by using transfixation pins or Kirschner wires that are connected with a single connecting bar (type Ia frame [unilateral frame or unilateral fixation]); two connecting bars opposed 90 degrees (type Ib frame [unilateral biplanar frame or biplanar fixation]); two connecting bars opposed 180 degrees (type IIa or IIb frame [bilateral frame or fixation]); or three connecting bars, that is, two connecting bars opposed 180 degrees with one connecting bar opposed 90 degrees from the other two connecting bars (type III frame [bilateral biplanar frame or bilateral biplanar fixation]) (Figure 44-2, A-D). Although a large variety of linear external skeletal fixator designs are commercially available internationally, the Kirschner-Ehmer (KE) system (also known as a K-E apparatus, Kirschner apparatus, Kirschner splint, or K-E splint) has become the mainstay linear external skeletal fixator design for use in small animal veterinary orthopedics in North America. The Kirschner-Ehmer system is available in three sizes: small, medium, and large. Generally, the small system is used in cats and small dogs, the medium system is used in medium-sized and large dogs, and the large system is reserved for use in giant breed dogs and other large species. Although the original double clamp type Ia (Figure 44-2, E) was the forerunner of today’s linear external skeletal fixator frames, it was found to be mechanically inferior (less stiff)16 to frame designs used today; therefore, it has generally been abandoned for use in small animal orthopedics. Besides mechanical superiority, newer frame designs and their components provide greater modularity over their older frame predecessors. Pins and Kirschner wires used as transfixation elements generally are made of stainless steel and have a trocar tip. Various designs are available, including smooth (usually Steinmann pins) and threaded types; threaded-positive profile or enhanced pins; and threaded-negative profile pins (Figure 44-3, A-C). Subtypes of positive-profile enhanced pins and wires include cortical and cancellous threads that may be central or end-threaded in location along the length of the pin or wire. Threaded pins and wires demonstrate superior axial pull-out strength compared with smooth pins when mechanically tested acutely and several weeks following surgical placement.1,2,11,31 Negative-profile pins usually have a fine thread design and are intended for insertion into areas of high lamellar bone density, such as the diaphyseal regions of long bones. These pins have threads that are machine-cut into the shank of the pin; therefore, the inner (minor) diameter of the thread area is smaller than the shank diameter. The difference in diameter at the thread-shaft interface generates a stress riser effect, which makes negative-profile pins susceptible to high bending moments and can result in implant failure at this location on the pin.1,11,22,88 Connecting bars (fixation rods) function to externally connect, via pin-connecting bar clamps, transfixation pins or wires of the linear system to the fixator frame. Connecting bars can be composed of a variety of materials; these materials include stainless steel, aluminum, carbon fiber, titanium, or acrylic, and are commercially available in several sizes and lengths (Figure 44-4, A-C). Stainless steel fixation rods that are used with Kirschner-Ehmer clamps (IMEX Veterinary Inc., Longview, Tex) are available in two sizes: small and medium-sized, to match the size of the Kirschner-Ehmer clamp (Figure 44-5, A). Connecting bar sizes and the diameters of transfixation pins accepted by these two sizes of Kirschner-Ehmer clamps are listed in Table 44-1. The large Kirschner-Ehmer clamp has been replaced by the large SK clamp (IMEX Veterinary Inc.) (Figure 44-5, B). Pin-connecting bar clamps are also available in single-or double-clamp designs. Connecting bar-to-connecting bar clamps or double-clamp designs allow for bridging or articulation of two connecting bars (e.g., type Ia double connecting bar system; type Ia, Ib, hybrids; type III frames) or a tie-in opportunity of the linear system with an intramedullary pin. Three significant limitations to the use of Kirschner-Ehmer clamps have been noted: (1) all Kirschner-Ehmer clamps must be applied to the connecting bar prior to transfixation pin insertion, as this clamp cannot be removed without removing the entire connecting bar from the frame (modifications to transfixation pin numbers cannot be done without disassembling the external skeletal fixation frame); (2) a propensity for acute structural deformity following single-use tightening of the clamp has been noted,56,57 with associated material weakness45; and (3) Kirschner-Ehmer clamps will not accommodate the thread diameter of positive-profile pins to allow pin placement through clamps preplaced on the Kirschner-Ehmer system connecting bar. This incompatibility between pin and clamp requires preplacement of all positive-profile pins before application of the frame, or, alternatively, the frame can be partially or fully disassembled and then reassembled following placement of positive-profile pins.49 The caveat to performing either of these techniques is the potential for loss of alignment and/or displacement of bone fragments during application of the fixation. Newer clamp designs such as the SK system, the Securos external skeletal fixation clamp, and the Securos Titan ESF clamp (Securos, Sturbridge, MA) (see Figure 44-5, A through D) allow greater modularity compared with Kirschner-Ehmer clamp designs and, as stated previously, greater ease of clamp application to a fixed connecting bar and application of positive-profile pins. These clamp designs are available in both single- and double-clamp models. Component specifications for the SK and Securos Kirschner-Ehmer systems are summarized in Table 44-1. The previous practice of reusing external skeletal fixator clamps for economic purposes is discouraged because of the finding that Kirschner-Ehmer, SK, and Securos-based clamp designs demonstrate significant potential for the degradation of clamp material properties (including clamp failure), especially with regard to connecting bar slippage through the clamp even after one-time usage in a multiuse testing model.45 Table • 44-1 Linear External Skeletal Fixation System Component Comparisons ESF, External skeletal fixator; KE, Kirschner-Ehmer. *IMEX Veterinary Inc., Longview, Tex. §Based on author’s clinical experience and manufacturer’s recommendations. Acrylic-based external skeletal fixation systems use acrylic columns that function both as connecting bars and as transfixation pin-connecting bar clamps. The term free-form fixation, rather than linear fixation, is used by some authors to identify these external skeletal fixation systems and is based on the fact that transfixation pin placement can be, unlike linear systems, angled out of plane (offset) to provide appropriate external fixation in regions or locations in which it would be difficult to place a contemporary linear or circular external skeletal fixation system (e.g., mandible). As long as the acrylic column engages all transfixation pins, the construct will achieve adequate stability for fracture healing. Acrylic systems offer several advantages: (1) the surgeon can contour the connecting bar to the surface shape of any fracture configuration, or body or joint angle, (2) acrylic provides a lightweight but strong mechanical option for external skeletal fixation, (3) the acrylic connecting bar–transfixation pin junction produces a stiffer and stronger frame compared with frames created using the conventional stainless steel pin-connecting bar clamp when exposed to the same mechanical loads,49 and (4) they offer a more economical option for external skeletal fixation compared with conventional linear systems that use stainless steel connecting bars and clamps. Mandibles and joints (transarticular) are ideal locations for the use of acrylic external skeletal fixation systems, but any indication for the use of a nonacrylic fixator would allow the use of an acrylic system (Figure 44-6, A). The interface between the transfixation pin and the acrylic column can be significantly strengthened by notching the pins or using pins manufactured with a knurled shaft (Acrylic Pin External Fixator [APEF] System, Innovative Animal Products, Rochester, MN). A knurled pin shaft or a pin surface with five notches manually created onto the pin shaft will significantly increase the bond of the acrylic to the pin, thus increasing the strength of the external skeletal fixator frame.14 An acrylic-based external skeletal fixation system kit is commercially available and is labeled as the APEF System. The kit contains all the materials required for construction of an acrylic system that is comparable in size and shape to a medium-sized or small linear external skeletal fixation system. Acrylic columns can also be “handmade” by working the acrylic into a dough consistency and applying the acrylic mass directly onto the transfixation pins, forming the mass into a single column. Alternatively, other acrylics can be used in liquid state and poured directly into capped tubes that have transfixation pins or wires that have been impaled into the tubes and are then incorporated into the acrylic column following acrylic polymerization and hardening (Figure 44-6, B).32,97 More commonly, polymethylmethacrylate-based acrylics such as Technovit (Jorvet, Loveland, CO.), Jet Denture Repair (Lang Dental Manufacturing Co. Inc., Wheeling, Ill), and the APEF System are used to create acrylic-based frames. Recently, a new acrylic putty is being marketed that does not require mixing of a liquid activator and a powdered base. The putty is packaged in a soft dough stage with a premeasured volume of activator and base (FastFix Putty, Securos). The material is kneaded to initiate the polymerization process without subsequent release of volatile fumes or spilling of a liquid mixture, which can occur commonly when other polymethylmethacrylate-based acrylics are used. The product’s polymerization and handling characteristics appear to be very similar to those of commercially available acrylic putties used in the automobile body repair industry in the United States. Polymethylmethacrylate-based acrylics undergo an exothermic reaction during the polymerization phase of hardening. The potential exists for the conduction of thermal damaging temperatures to soft tissues and bone by heat transfer from the acrylic column to transfixation pins.76,110 Maintaining a gap of 1 cm between soft tissue/bony tissue and the acrylic column will prevent the conduction of thermal damaging temperatures to these tissues.76,110 Dripping saline onto the transfixation pin will maintain lower thermal conduction along the pin as well.110 Circular external skeletal fixation, or ring fixators, use supporting rings, connecting rods, bolts, and tensioned transfixation Kirschner wires (Figure 44-7). The method used for placement of circular external skeletal fixation is termed the Ilizarov method.70,106 The geometry of a circular external skeletal fixator frame produces axial dynamic skeletal fixation in a three-dimensional tubular-shaped exoskeleton compared with the static fixation created in a two-dimensional plane by linear external skeletal fixation. As with linear external skeletal fixators, circular external skeletal fixators offer unlimited geometric adaptability and allow for frame adjustments after surgical creation of the construct. All components of the circular external skeletal fixator can be tailored for the purpose of fixation and according to the anatomy of the patient. Although circular external skeletal fixation systems are commercially available from many international sources, an extensive line of circular external skeletal fixation system components and instrumentation is manufactured by IMEX Veterinary Inc., which also provides a hybrid circular-linear external skeletal fixation system that can be used effectively to stabilize many proximal or distal long bone fractures in dogs and cats.37,55 Circular external skeletal fixation can be used to stabilize a variety of long bone fractures,66,70 spinal fractures, and luxations107; to dynamically correct angular limb deformities65,71; and to provide rigid fixation for arthrodesis.66 Because of their dimensional complexity, application and use of circular external skeletal fixators and their hybrids in dogs and cats is considered to require advanced knowledge and training compared with linear external skeletal fixation systems, especially when circular external skeletal fixation is used to dynamically correct angular limb deformities that require the Ilizarov method of distraction osteogenesis.106 Tensioned Kirschner wires are used to transfix the bone to the circular external skeletal fixator frame. The bending stiffness of a tensioned wire is significantly greater than the bending stiffness of a loose wire. A tensioned 0.062″ (1.6 mm) wire has bending stiffness equivalent to that of a 5/32″ (4.0 mm) Steinmann pin used as a half pin.9 As such, circular external skeletal fixator frames can be used in larger dogs with excellent results. Two forms of Kirschner wires are used to create circular external skeletal fixator frames: smooth and olive wires (smooth wires with stoppers). Olive wires have a relatively large-diameter ball positioned at the midpoint on the wire (Figure 44-8, A-B). Olive wires can strengthen the fixation and allow translation of bone fragments with traction produced by a hand-held tensioner to improve fragment reduction at the fracture site, or they can be used to facilitate fragment alignment. Generally two wires are used; ideally they are positioned 90 degrees to each other and are secured to each ring with wire bolts. Wires are placed with one proximal and one distal on each ring. When placed in this manner, they do not interfere with each other when they cross within the bone. Rings used with circular external skeletal fixation are available in a variety of diameters and are composed of aluminum or carbon fiber. A ring of appropriate size should produce clearance 10 mm from the inside edge of the ring to the skin. Threaded rods (usually three, or a composite of three rods) are used to allow complete freedom to position the rings on the limb to maximize stability. The bone segments are transfixed with tensioned Kirschner wires, and the wires are connected to the rings. Ideally, the circular external skeletal fixator frame should be composed of four rings using the “far-near, near-far technique” for ring placement in relationship to the fracture site, with the rings spanning the entire length of bone fragments to be stabilized.70 Partial rings or arches can be used to avoid interference with joint motion when a ring must be placed close to an adjacent joint. A single ring can be used for a fracture close to a joint. To obtain adequate bone stability in this region, additional fixation in the form of an olive wire, a dropped wire, or a half pin should be used.70 Slotted or cannulated bolts can be used to secure the transfixation wire to the ring. Wires can also be connected to posts or to threaded rods using slotted washers. Nuts are used to secure the rings in position on the threaded bars and to secure slotted or cannulated bolts onto the rings. Numerous studies have been performed to evaluate the overall strength and stiffness (rigidity) of external skeletal fixation as used in veterinary and human medicine.* Knowledge of the overall mechanical strength and stiffness of the external skeletal fixation construct is important for fracture management planning. Several factors influence the resulting biomechanics of the external skeletal fixation construct, including frame geometry, number of connecting bars, and number of transfixation pins, as well as type (e.g., threaded, smooth) and size. The biomechanical behavior of the construct will also depend on the clinical and biological characteristics of the patient (e.g., size, age, behavior) and the physical and anatomic condition and section of bone to be stabilized (e.g., juvenile or adult bone, diaphyseal [cortical] or metaphyseal [cancellous] bone, comminuted or simple fracture, healthy or diseased bone). The strength of six commonly used linear external skeletal fixator frame configurations has been evaluated under static unidirectional axial compression, shear, and torsional loads.33 In general, the increase in external skeletal fixator frame complexity was proportional to an increase in the mechanical strength and stiffness properties of the frame; type Ia, Ib, IIa, IIb, and III linear external skeletal fixator frames (see Figure 44-2) are successfully stronger in resisting axial and torsional loads. The order of external skeletal fixator frame number designation followed the same pattern for increasing resistance to shear as with axial and torsional loads, with the exception that type Ib frames have greater resistance to shear than type II frames. As such, type Ia and Ib frame configurations are considered to provide ideal stabilization for simple fractures, while type II and III frame configurations are mechanically suited for the treatment of complex fractures in dogs and cats. Although type I frames generally are considered weaker in terms of strength and stiffness than other frame configurations, placing a second connecting bar on a type Ia frame will increase the frame’s resistance to axial stiffness by a factor of 2.5.16,33,109 The use of a solid external plate augmentation to a single stainless steel connecting bar (Unilateral Augmentation System, Securos) will produce almost a 4.5-fold increase in axial stiffness and medial-lateral bending, and twofold increased stiffness to cranial-caudal bending compared with standard stainless steel single connecting bar type Ia constructs80; however, a double stainless steel connecting bar type Ia frame will be 80% stiffer in axial compression and 170% stiffer in medial-lateral bending compared with the external augmented plate system.109 It is interesting to note that the mechanical gain in stiffness under axial compression achieved by adding a second connecting bar to a type Ia frame is approximately equal to the mechanical stiffness reported for type IIb frames and approximately 50% of the mechanical stiffness of a type IIa frame, especially when larger-diameter connecting bars composed of newer materials such as carbon fiber are used.109 The effect of using larger-diameter connecting bars to increase frame stiffness is eventually negated when frames are constructed with two or more full pins, as are used in type II and type III frame designs.17 In these advanced frame designs, the developed mechanical stiffness becomes more a function of the transfixation pin size, the number of pins incorporated into the frame, and the orientation of pins along the frame.17 Developments in terms of stronger frame components,17 hybrid frame designs,37,38 frame tie-in methods with intramedullary pins,6 and threaded pins1,2 have substantially improved the mechanical properties of the type I frame, so it is now possible for this frame configuration to provide adequate stabilization for all forms of long bone fractures, especially in fractures proximal to the elbow and stifle—regions that do not allow the application of type II or III frame configurations. External skeletal fixator frame stiffness can be increased and pin-bone interface stress decreased by increasing the number of transfixation pins incorporated into the frame by using up to six or eight pins per construct (three to four pins per fragment).13,16,87 A minimum insertion of two pins per fragment is always required to create at least two-point fixation of all fragments within the construct. Two-point fixation prevents rotational instability of the fragment that would otherwise occur around the central axis of a single pin insertion. Placing more than four pins per fragment does not significantly increase frame stiffness.15,16 Reducing pin-bone interface stress will decrease the incidence of bone resorption around the pin and subsequent loosening of the external skeletal fixator frame from the construct. Placing smooth pins at a 70 degree angle to the long axis of the bone will enhance the pin-bone interface purchase and increase the stiffness of the external skeletal fixation construct.16 Transfixation pins ideally should be evenly spaced along the length of each fragment to decrease the stress forces developed at each interface point. The pins should be placed no closer than three times their diameter, or half the diameter of the bone, from joints and fracture edges. Depending on fracture location and/or the seated angle required for smooth transfixation pins and the potential for insertion interference from other angled adjacent pins, it may not be possible to place three or four transfixation pins per fragment. In those cases, fragments transfixed with a lesser number of pins will be expected to show clinical evidence of loosening (drainage at the pin-skin interface, radiographic evidence of bone resorption around the pin) sooner compared with fragments transfixed with a greater number of pins. Unlike smooth transfixation pins, positive-profile pins are placed perpendicular to the long axis of the bone; this allows less interference from adjacent pins during insertion and the opportunity to place more pins per fragment based on fracture position. The use of larger-diameter transfixation pins or threaded transfixation pins can also significantly increase external skeletal fixation construct stiffness, no matter the frame configuration used for fracture fixation. The bending stiffness of a pin is determined by the area moment of inertia of the pin and is based on the fourth power of the diameter of the pin.78,87 Therefore, a small increase in pin diameter results in a large increase in bending moment stiffness for the pin and the external skeletal fixation construct. This concept also applies when the connecting bar size to be used for the frame is selected. The optimal pin size diameter to use with an external skeletal fixation system will be influenced by the diameter of the bone fragments present. The bone hole created to accommodate a larger pin acts as a stress riser and weakens the bone in torsion and bending. To prevent a stress-riser effect of the bone hole created by pin insertion, the diameter of transfixation pins should not exceed 20% to 30% of the diameter of the bone. As previously mentioned, the incorporation of larger-diameter connecting bars into an external skeletal fixator frame will result in greater stiffness of the external skeletal fixator.16 External skeletal fixator stiffness can also be enhanced by positioning the connecting bar in close proximity to the central bone axis by placing the pin-connecting bar clamp to the inside of the connecting bar. The effect is a reduction in distance between the skin and the connecting bar, which functionally minimizes the working length of the transfixation pin. Shorter pins are stiffer than longer pins because pin stiffness is proportional to pin length to the third power.13 A shorter working length of the pin increases the pin’s resistance to a bending moment; this contributes to a stiffer external skeletal fixator.87 The pin-bone interface is the most highly mechanically stressed position on the external skeletal fixation construct.* Preserving the integrity of this interface is essential in maintaining the long-term durability of an external skeletal fixator after surgical application of the frame and in minimizing the complication of premature frame loosening during the treatment period.22 Positive-profile pins are strongly recommended for use as transfixation pins for external skeletal fixators over negative-profile pins because they are stronger (equal shank diameter throughout the pin length) and have the added property of increasing bone-holding capacity compared with smooth pins in all but the simplest fractures.3 Because of manufacturing differences between positive- and negative-profile pins, the thread-nonthread junction of a negative-profile pin can become a stress riser for concentrated cyclic bending forces, and this can lead to material failure at this point on the pin.1,11,22,88 The method of transfixation pin placement used is critical for the pin-bone interface. Direct trocar-pointed smooth pin insertion with a hand-held chuck, if not done carefully, can result in marked wobbling during insertion and the creation of a functionally larger bone hole, leading to poor pin-bone contact and premature loosening of the fixator. Although the use of power drill insertion for pins reduces the wobble effect of hand-drilled insertion, it is critical to keep the speed of insertion low (<150 rpm) to reduce the development of pin heat during insertion and the potential for bone necrosis and resulting resorption of bone at the pin-bone interface.31 To prevent direct mechanical damage to bone and weakening of the pin-bone interface during insertion of positive-profile pins, it is recommended to create a pilot hole with a drill bit that is sized slightly smaller than the shank pin diameter.23 Offset (or out of plane) positioning of transfixation pins may also enhance pin-bone interface functionality. A 60-degree offset transfixation pin angle can produce a four-fold to five-fold increase in type Ia frame stiffness when the construct is subjected to axial compression and cranial-caudal static load forces.83 Although offset pin angle insertion is not feasible for use with currently marketed linear systems, the use of a hand-made acrylic connecting bar column allows the inclusion of offset pins in the acrylic mass and the ability to use the offset angle mechanical benefit for an acrylic-based external skeletal fixator frame. Circular external skeletal fixators offer several distinct advantages over other fixation modalities (e.g., intramedullary pins and cerclage wire, bone plates and screws, interlocking nails, some linear external skeletal fixation systems). These advantages include (1) excellent dynamic mechanical properties (increased stiffness when subjected to shear and bending forces, and reduced stiffness when subjected to axial compressive loads), (2) the ability to stabilize short bone fracture segments (10- to 15-mm long), and (3) frames that are fully adjustable after bone fixation, allowing for acute or progressive translation, rotation, or angulation of bone segments.67 As with linear external skeletal fixation systems, circular external skeletal fixators have been used to successfully treat acute or chronic fractures, open fractures with or without adjacent joint instability, infected or sterile nonunions, and malunions. Circular external skeletal fixators provide an excellent stabilization option for treating long bone fractures. As with type II and III frames, circular external skeletal fixators are better applied to fractures distal to the stifle, especially (1) when bone fragments are physically or functionally (e.g., fissures present) too short to transfix a minimum number of transfixation pins (two), (2) when bone defects are present, or (3) when postoperative adjustability is desired following frame application to the limb.70 Ideally, four wires are used in each bone segment, and as with transfixation pins or wires of linear external skeletal fixation systems, they should span the length of the bone as evenly as possible and should avoid all fracture or fissure lines. Recommended wire diameters for cats and dogs are listed in Table 44-2.67 Smooth wires with stoppers (olive wires) are also available with large-diameter stoppers at the midpoint that decrease translation of the bone in relation to the wire. Several options are available to achieve adequate transfixation when a bone segment is too short for the placement of two rings; these include (1) a single ring with olive wires transfixed into the bone, (2) a third wire placed on posts or on threaded rods, and (3) one or preferably two or three divergent half pins added with the use of posts or fixation cubes.68,70 Similar to linear hybrid frames, hybrid linear-circular external skeletal fixators provide the mechanical properties of both systems and, because of the geometry of the final frame design, can be used on long bone fractures above and below the elbow and stifle.37,38 Wire tension force recommendations are listed in Table 44-2.67 Wires connected to posts, connecting rods, or partial rings should not be tensioned more than 30 kg to avoid frame deformation.67 Table • 44-2 Recommendations for Wire Size and Wire Tension Force for Circular External Skeletal Fixation Systems67
External Skeletal Fixation
Introduction and History
Components and Nomenclature of External Skeletal Fixators
Linear External Skeletal Fixation Systems
Pins/Kirschner Wires Used in Linear External Skeletal Fixation Systems
Connecting Bars and Pin-Connecting Bar Clamps Used in Linear External Skeletal Fixation Systems
Circular External Skeletal Fixation Systems
Transfixation Kirschner Wires Used in Circular External Skeletal Fixation Systems
Rings, Threaded Rods, and Nuts/Bolts Used in Circular External Skeletal Fixation Systems
External Skeletal Fixator Frame Configurations and Biomechanics
Stay updated, free articles. Join our Telegram channel

Full access? Get Clinical Tree
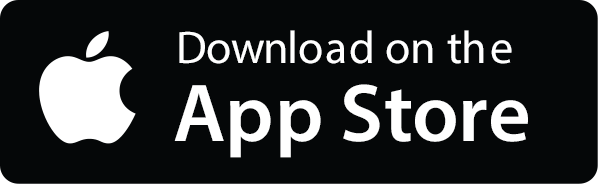
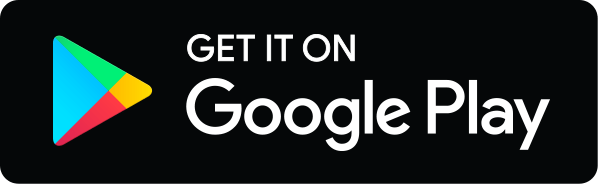