Martin Furr
Equine Protozoal Myelitis
Equine protozoal myeloencephalitis (EPM) is a commonly diagnosed condition of the horse, which was originally described in 1964 by J. Rooney, and termed “segmental myelitis.” An organism was eventually cultured from an affected horse in 1991 and named Sarcocystis neurona because of its association with neurons. Since that time, the organism has been cultured from several other ataxic horses, and multiple strains of the parasite have been described. In addition to S neurona, the protozoan parasite Neospora hughesi has been recovered from a small number of horses with EPM-like disease, and it must also be considered a cause of EPM, although a less common one.
Epidemiology
Equine protozoal myeloencephalitis is a disease of the American continent, and most horses outside of that geographic region with a putative diagnosis of EPM caused by S neurona have a history of previous residence in the endemic region. Horses in the United States have a variable but generally high serologic positive incidence to S neurona, ranging from 10% to 60%. The seroprevalence for N hughesi is generally low, although the reported seroprevalence is 37% in California.
All horses are susceptible to development of EPM, but epidemiologic surveys have suggested that younger horses (3 to 4 years) have a higher risk for disease, with an age range of reported cases from 2 months to 24 years. Horses of all breeds appear to be affected, and there is no sex bias. Most cases appear to be individual cases, and “outbreaks” of EPM do not appear to occur.
Despite the often high rate of exposure to the organism, however, only a small percentage (perhaps <1%) of horses develop clinical illness. This suggests that immune clearance of the parasite is very effective, but that unknown factors must exist in certain cases to allow clinical disease to be expressed. Parasite dose and differences in strain-specific virulence likely play a role. Other factors that have been considered to have a role in the induction of EPM include physiologic stress associated with shipping, training, showing, and pregnancy, which may make animals more susceptible to EPM. It is assumed that these stressors lead to some degree of immune suppression, which is a commonly implicated factor in protozoan parasite infections. Additional evidence for the role of physiologic stress is found in the observation that stressed horses develop more severe clinical signs than naturally infected (nonstressed) horses. It thus appears likely that stress has a role in the development of EPM, but the interaction is complex and not fully understood at present.
Sarcocystis neurona is a typical coccidian parasite in that it has a two-host life cycle. During part of the parasite’s life it resides in one host, referred to as the definitive host, whereas during another phase of its life cycle, it resides in the tissues of a second animal, known as the intermediate host. Sexual reproduction takes place in the definitive host, whereas asexual reproduction (i.e., growth and replication) takes place in the intermediate host. It has been known for many years that the definitive host for S neurona is the opossum. This has been supported by numerous feeding studies, examination of feral opossums, and molecular studies in this species. Further, the clinical disease of EPM is found to coincide with the environmental range of the opossum. The identification of the intermediate hosts has remained much more challenging, and studies from several laboratories have identified multiple intermediate hosts, including the raccoon, skunk, armadillo, and possibly the domestic cat. Horses become infected by consuming infective sporocysts that have been shed by the opossum into the environment. The horse has been considered to be a dead-end host and unable to transmit the disease to other horses, or in fact to support completion of the life cycle of the organism. This position has been challenged, however, because mature schizonts and sarcocysts have been documented in a 4-month–old foal with clinical signs of EPM. Further work is required to corroborate this finding.
Pathophysiology
During the normal life cycle of S neurona, sporocysts are passed in the feces of the opossum, contaminating feed and water, and are then ingested by the intermediate host. Once within the gastrointestinal tract, the sporocysts excyst and release eight sporozoites, which penetrate the gut and enter arterial endothelial cells in various organs. Meronts develop within the host cells, which then rupture and release merozoites into the bloodstream. In the appropriate intermediate host, sarcocysts form in various muscle tissues. After the intermediate host dies, the flesh is consumed by the definitive host, completing the life cycle.
The development of S neurona has been carefully examined in immunodeficient mouse strains, in which it appears that there is a hematogenous distribution of parasite occurring rapidly (within days) and with widespread seeding of tissues. The development of the parasite in immunocompetent horses has not been similarly examined; however, parasitemia in immunodeficient horses has been demonstrated, and a transient parasitemia has been reported in one immunocompetent horse after oral challenge with S neurona.
The mechanism by which the parasite enters the central nervous system (CNS) is unknown, but the organism may enter the CNS directly through the cytoplasm of infected endothelial cells, or alternatively and perhaps more likely, the organism is carried into the CNS by infected lymphocytes during tissue surveillance, a sequence referred to as the “Trojan horse” hypothesis.
Resistance to S neurona is presumed to be a result of the combined effects of humoral and cellular immunity. After infection, there is relatively rapid production of antibodies (e.g., 13 to 32 days), which appears to depend on the inoculating dose and concomitant stress. Antibodies against protozoan parasites have been demonstrated to block infection with the organisms, and research with S neurona has demonstrated that antibodies against the SN14 and SN16 surface proteins (analogous to snSAG-2) block target cell penetration; blocking the SN30 surface protein (analogous to snSAG-1) had no effect. This finding is in contrast to another report, in which it was suggested that the snSAG-1 protein was important in mediating infection.
Although the effects of circulating antibody are likely to be very important, cell-mediated immunity is necessary for the elimination of intracellular forms of most organisms. In immunocompetent mice, there is a significant increase in both total CD8+ splenocytes, as well as an increase in the percentage of CD8+ peripheral blood lymphocytes, following infection with S neurona. Similar studies in the horse have resulted in conflicting results regarding the changes in CD4+ and CD8+ T-cell responses, whereas another study showed no difference in lymphocyte phenotype subsets in the peripheral blood or cerebrospinal fluid (CSF) of horses with EPM. Meningoencephalitis developed in CD8 knockout mice following challenge with S neurona, highlighting the importance of this cell subset in protection against S neurona. The CD8+ T cells (i.e., cytotoxic T cells) are one important source of interferon-γ (IFN-γ), which is widely recognized as being important in protection against S neurona. Clearly, a substantial amount of work remains to be done to elucidate the pathophysiologic mechanisms involved with S neurona infection in horses.
Epidemiologic studies have suggested that stress has at least a permissive effect on the development of EPM, but there is also evidence that the presence of the organism may itself be immunosuppressive. Cell-mediated immune responses to mitogens have been shown to be reduced in horses with EPM; however, it is unclear whether this was a cause or an effect of infection. Furthermore, IFN-γ messenger RNA production was diminished in lymphocytes from EPM-positive horses. These were in vitro studies, which may not directly represent the in vivo situation, but even local immunosuppression in the microenvironment of the parasite may be significant in an infected animal.
Clinical Signs
After the organism has infected the nervous system, it leads to localized inflammation with clinical neurologic signs that are dependent on the anatomic site of infection within the CNS. In general, S neurona–induced neurologic disease (as well as disease caused by N hughesi) results in clinical signs of muscle atrophy and ataxia, with asymmetry. This reflects the random distribution of the organism within the CNS, affecting upper motor neurons, lower motor neurons, or both. Symmetric illness can be seen, however, and this should not lead one to totally exclude a diagnosis of EPM. Spinal cord signs predominate, leading to ataxia, stumbling, or weakness. The gluteal muscles appear to be the most commonly affected (or at least the most easily noticed), but any muscle can be involved, including the tongue. Progression of clinical signs is typically chronic, but acute signs can also be seen. Cranial nerve signs have been reported in up to 12% of reported cases. These signs include muscle wasting of the temporalis and masseter muscles, head tilt, or dysphagia. Cerebral signs can also be seen, although rarely, and these include blindness, seizure activity, and altered mentation.
Diagnosis
The clinical diagnosis of EPM remains a challenging task for veterinarians. Variable and sometimes subtle presentation of clinical signs and the inherent complexities of the diagnostic tests make a clear-cut and confirmed diagnosis of EPM in the clinical patient difficult. At present, the diagnosis of EPM must be considered presumptive in the live horse. Given these constraints, however, careful interpretation of clinical and appropriate ancillary diagnostic laboratory testing can result in a diagnosis with a high degree of confidence. Diagnosis is dependent on the following:
Clinical Examination
A thorough physical and neurologic examination remains the keystone for establishing a diagnosis of EPM. For a diagnosis of EPM to be supported, conclusive evidence of CNS disease must be present. Musculoskeletal disease should be ruled out, if possible, by appropriate means. This may involve flexion tests and local nerve or joint blocks. Concurrent disease is possible and must be considered. Signs of systemic illness, such as fever, anorexia, respiratory distress, weight loss, and dehydration, are generally not seen. After a physical examination is completed, ancillary testing is important to gather further information. The nature and extent of ancillary testing will be determined by the results of the neurologic examination, but most commonly this will include radiographs of the cervical portion of the spine and CSF collection and evaluation.
Cerebrospinal fluid collection and evaluation are recommended for full examination of any horse with CNS disease. Cerebrospinal fluid should be evaluated for red blood and nucleated cell count, total protein, and glucose concentrations, as well as be examined cytologically. A full evaluation of the CSF is important in identifying horses with viral or bacterial meningoencephalitis, tumors, or trauma, which are important differential diagnosis considerations for horses with CNS disease. The CSF is usually normal in horses with EPM; however, this finding has diagnostic value in ruling out other conditions of the equine CNS.
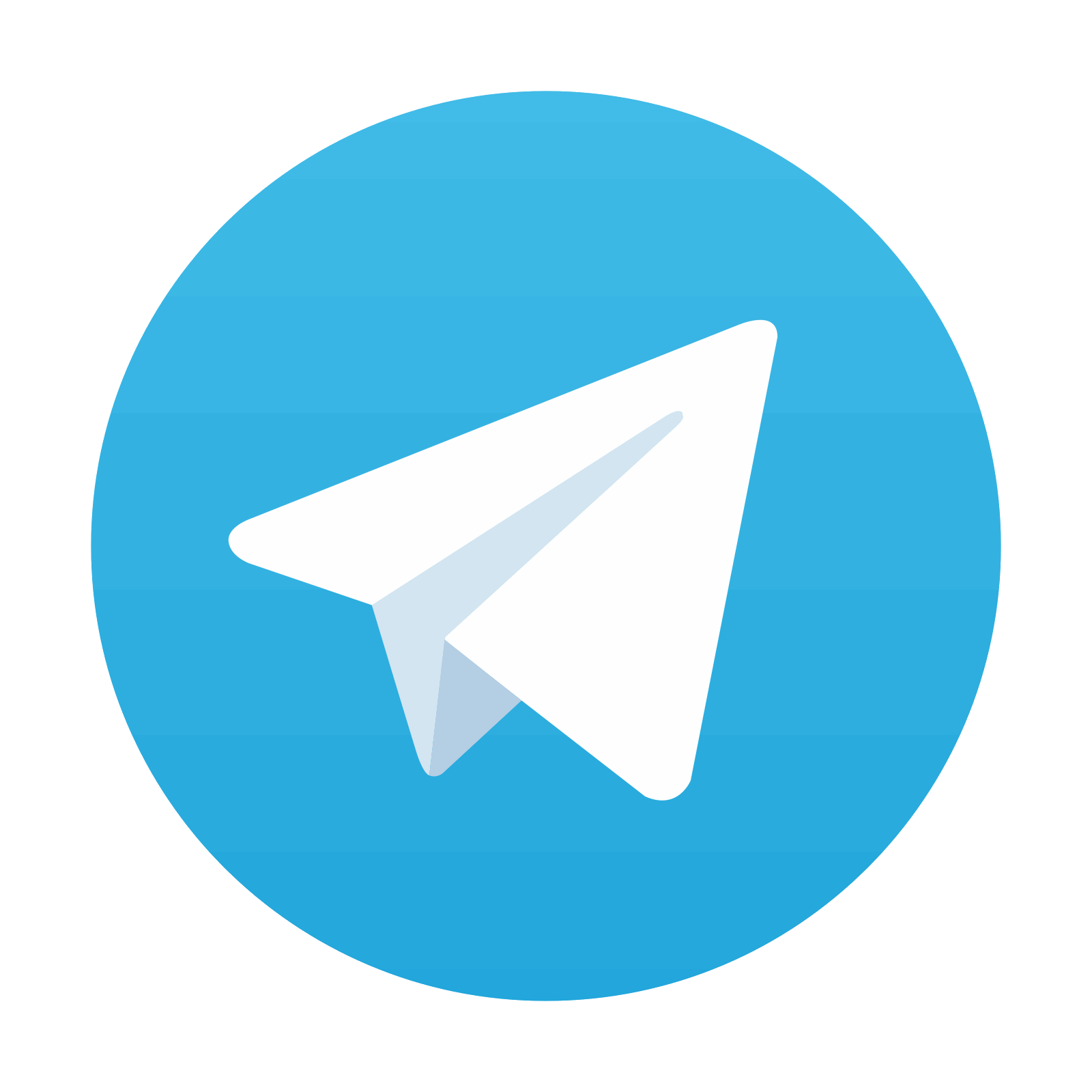
Stay updated, free articles. Join our Telegram channel

Full access? Get Clinical Tree
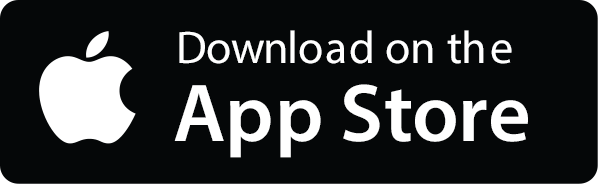
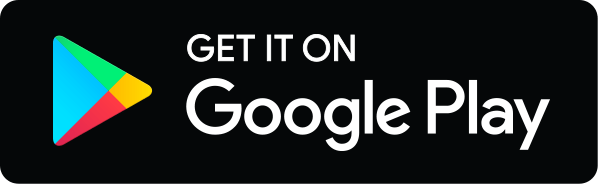