48 Mark G. Papich The afferent arc of the cough reflex receives input from sensory nerves in the bronchial and tracheal airways. Airway irritation and inflammation stimulate the afferent nerves, which in turn activate the cough center located in the medulla oblongata. The antitussive drugs directly depress the cough center in the medulla. The site of action may be either μ- or κ-opiate receptors (discussed in Chapter 13). Opiate drugs that act on these receptors are effective, but it is not simply because they produce sedation (Morjaria et al., 2012). Either of the opiate receptors may be responsible for the action because both butorphanol (κ-receptor agonist) and codeine or morphine (µ-receptor agonists) can suppress cough (Takahama and Shirasaki, 2007; Gingerich et al., 1983; Christie et al., 1980; Morjaria et al., 2012), but naloxone is capable of antagonizing this effect. There may be a nonopiate mechanism for some antitussives as well (Morjaria et al., 2012). For example, dextromethorphan is an opiate derivative, but does not have activity at opiate receptors and may bind to sites in the brain that are distinct from opiate receptors. It is continues to be marketed as an over-the-counter (OTC) antitussive for people – apparently based on early clinical trials. Its mechanism is unknown. The neurokinin receptor (NK) may play a role in antitussive activity of such nonopiates. This mechanism has not been well characterized (Takahama and Shirasaki, 2007), but is discussed further with the NK-1 receptor antagonist drugs below. Beta-2 receptor agonists (β2) will suppress cough in some animals. The effect of these drugs is mediated via bronchodilation. These drugs will be discussed in Section Bronchodilator Drugs (β-Adrenergic Receptor Agonists). Opioids are administered as premedications prior to surgery. This provides a sedative effect, analgesia, and also may facilitate intubation. Inhibition of the cough reflex may decrease laryngeal spasms and coughing associated with intubation during anesthesia induction. Morphine and derivatives have opiate-receptor–mediated effects, but also are effective as antitussives. Morphine is a natural derivative of one of the alkaloids of opium (Chapter 13). Morphine is the prototype of an opiate (narcotic) analgesic with good binding affinity for the µ- and κ-opiate receptors. Morphine can be administered at low doses that produce antitussive effects without causing analgesia and sedation. The antitussive dose has been reported to be approximately 0.1 mg/kg, q 6–8 h. Morphine is not regularly used as an antitussive because of the side effects and potential for abuse and addiction in humans. Oral formulations of morphine do not have good systemic absorption in dogs (see Chapter 13). Codeine phosphate and codeine sulfate are found in many preparations including tablets, liquids, and syrups. There are over 50 different combinations and preparations of codeine. However, codeine oral absorption in dogs is low and inconsistent. Codeine has analgesic effects that are approximately 1/10 that of morphine. Studies in people have shown little benefit from codeine for cough suppression, but the small amount of codeine metabolized to morphine may produce an antitussive effect. In dogs codeine administered orally attains systemic levels of only 4% (KuKanich, 2010), but it is possible that other metabolites may be responsible for the antitussive effect. Despite the occasional use in dogs, the efficacy for treating cough has not been studied. It is available as 15, 30, and 60-mg tablets and oral syrups (2 mg/ml). In some states (regulated by local regulations), and some countries, codeine in antitussive formulations may be obtained without prescription. In people, the side effects of codeine at antitussive doses are significantly less than those experienced with morphine. The potential for addiction and abuse is considerably lower. Important side effects include sedation and constipation. Hydrocodone is similar to codeine in mechanism of action, but it is more potent. Hydrocodone is combined with an anticholinergic drug (homatropine) in the preparation Hycodan®, which has been prescribed often for small animals. Pharmacokinetic studies have shown that is absorbed orally in dogs but there is conflicting evidence in dogs on the metabolism to hydromorphone (Benitez et al., 2015). It is possible that the antitussive effect is produced by a different metabolite. Nevertheless, some veterinarians consider it their first drug of choice for symptomatic treatment of cough in dogs. The anticholinergic component (homatropine) is added to discourage abuse rather than for a respiratory indication. Oral doses administered to dogs are approximately 0.22–0.25 mg/kg, q 6–8 h (approximately 1 tablet per 20 kg). Hydodan® is a combination product with hydrocodone bitartrate formulated as 5 mg of hydrocodone + 1.5 mg homatropine. In some countries it is available without an anticholinergic (5 mg). Hydrocodone (5 mg) + acetaminophen (500 mg) is combined in a tablet and used for analgesia (Vicodin). Because of the acetaminophen in these products, they should never be used in cats. In dogs, high acetaminophen exposure may result from the high doses necessary. In the United States recently, hydromorphone was rescheduled by the DEA from Schedule III, to Schedule II, which makes prescribing more restrictive. Dextromethorphan is not a true opiate because it does not bind µ- or κ-opiate receptors, but studies in people have supported its antitussive effects. More recent investigations have questioned the antitussive properties of OTC formulations. Dextromethorphan is the D-isomer of levorphan (the L-isomer, levorphan is an opiate with addictive properties, but the D-isomer is not). Dextromethorphan produces mild analgesia and modulates pain via its ability to act as an NMDA (N-methyl D-aspartate) antagonist, but this is unrelated to the antitussive action (Pozzi et al., 2006). Dextromethorphan has been administered to dogs and cats, but pharmacokinetic studies in dogs indicated that dextromethorphan does not attain effective concentrations after oral administration (KuKanich and Papich, 2004a). After IV injection it produced adverse effects in dogs (vomiting after oral doses, and central nervous system reactions after IV administration). Even after IV administration, concentrations of the parent drug and active metabolite persisted for only a short time after dosing. Therefore, routine use in dogs is not recommended until more data are available to establish safe and effective doses. There are many preparations available without a prescription (OTC) in liquid and tablet form. For example, Vicks Formula 44® and Robitussin® contain dextromethorphan as their active antitussive ingredient. OTC formulations may vary in concentration, but most contain 2 mg/ml, or 15 or 20 mg per tablet. Pet owners should be cautioned that many OTC preparations contain other drugs that may produce significant side effects. For example, some combinations also contain acetaminophen, which can be toxic to cats. Some preparations also contain a decongestant, such as pseudoephedrine, which can cause excitement and other side effects. Butorphanol is an opioid agonist–antagonist that has been used both as an analgesic and antitussive. It has been a potent antitussive with clinical studies that support its use in dogs (Gingerich et al., 1983; Christie et al., 1980). High doses may induce side effects such as sedation. Butorphanol is poorly bioavailable because of oral first-pass metabolism. Therefore, in dogs the oral dose is higher (0.55 to 1.1 mg/kg) than the IV or SC dose (0.05 to 0.1 mg/kg). It is administered as frequently as needed to control cough – usually every 6 to 12 hours. In clinical studies the peak effect was rapid after the injectable formulation. After oral administration, the maximum effects were observed for 4 hours but persisted for up to 10 hours in dogs (Gingerich et al., 1983). By contrast, codeine’s effect in dogs is much shorter. It is available as 1, 5, and 10-mg tablets and an injectable solution. Tramadol is an inexpensive oral drug used for analgesia. It possesses opiate, serotonin, and α2 activity. (The pharmacology of tramadol is discussed in more detail in Chapter 13). The active metabolite has opiate activity and is believed to be responsible for most of the analgesia. This drug also has antitussive properties and appears to be well tolerated in dogs, and it is not a controlled substance. However, efficacy for treating cough in dogs has not been evaluated. The recommended oral dose is 5 mg/kg, every 6 hours, but this has not been tested for antitussive efficacy (KuKanich and Papich, 2004b). The β-adrenergic receptor agonists have a beneficial effect in the treatment of some airway diseases. They are used commonly in people for the treatment of asthma. In animals, they are used for airway disease, allergic bronchitis, and similar diseases such as feline “asthma,” recurrent airway obstruction (RAO) (“heaves”), and inflammatory airway disease (IAD) in horses. The autonomic properties are discussed in more detail in Chapter 7 of this textbook, and the application to respiratory diseases will be included here. Bronchial smooth muscle is innervated by β2-adrenergic receptors. Stimulation of β2 receptors leads to increased activity of the enzyme adenylate cyclase, increased intracellular cyclic adenosine monophosphate (cyclic-AMP), and relaxation of bronchial smooth muscle (see Chapter 7). Stimulation of β-receptor activity on mast cells decreases release of inflammatory mediators. The β receptor on mast cells produces a stabilizing effect (inhibition of mediator release) (Chong et al., 2002). There is little effect on other inflammatory cells. Therefore, when airway inflammation is mediated by neutrophils and/or eosinophils, this property of the β agonists may be less important. Cytological examination of fluid from a tracheal wash or bronchoalveolar lavage may help in making this distinction. There is some evidence that β-adrenergic receptor agonists increase mucociliary clearance in the respiratory tract. The clinical significance of this effect has not been established (Norton et al., 2013). The β agonists discussed below should be used for short-term relief of bronchospasm. They should be avoided for long-term use as repeated administration may diminish the response because of a change in receptors after prolonged exposure. The receptor changes during chronic administration are a result of down-regulation and desensitization. Concurrent use of corticosteroids may attenuate the desensitization. For management of airway diseases, other drugs (e.g., corticosteroids) can be used for long-term maintenance, and β agonists used short term during acute exacerbations of bronchoconstriction. Epinephrine stimulates α- and β-adrenergic receptors (Table 48.1). It produces pronounced vasopressive and cardiac effects. Epinephrine is considered the drug of choice for the emergency treatment of life-threatening bronchoconstriction, such as during an anaphylactic reaction. The nonspecific stimulation of other receptors and its short duration make it unsuitable for long-term use. The dose used in animals is 10 µg/kg IM or IV administered as a one-time treatment, or repeated in 15 minutes. Formulations are available in either 1 : 10,000 concentration (0.01%, 0.1 mg/ml) or 1 : 1,000 concentration (0.1%, 1 mg/ml). Duration of action is short (less than 1 hour). Norepinephrine (Levarterenol) has similar β1 effects as epinephrine but fewer α1 and β2 effects, and therefore is not suitable for respiratory therapy. Table 48.1 Relative effects of catecholamines and adrenergic agonists on adrenergic receptors DA, dopamine. Isoproterenol is a potent β receptor agonist. It is selective for β receptors with little α-receptor–mediated effects, but the cardiac (β1) effects make it unsuitable for long-term use. It has been administered by inhalation or injection. It has a short duration of action (less than 1 hour). To avoid the adverse cardiac effects of β-adrenergic agonists, drugs have been developed that are more specific for the β2-receptor (Table 48.1). These drugs are preferred for repeated use or long-term use. These drugs are also incorporated in some of the metered-dose inhalers used to treat bronchoconstriction. Terbutaline is similar to isoproterenol for its β2 activity, but it is longer acting (6 to 8 hours). It may be injected subcutaneously to relieve an acute episode of bronchoconstriction. (The dose in people is approximately 3.5 µg/kg, repeated once per hour.) The oral dose is 2.5 mg/dog q 8 h for dogs, and 0.625 mg (one-quarter of a 2.5-mg tablet) q 12 h for cats (e.g., cats with feline “asthma”) (Hawkins and Papich, 2014). It is available as 2.5 and 5-mg tablets and 0.8 mg/ml (820 µ/ml) injection. The injection can be helpful for treatment of acute bronchoconstriction. The dose for injection in cats is 0.01 mg/kg IV or IM. Terbutaline has been used in horses to treat recurrent airway obstruction (RAO), which is a bronchoconstrictive disease in horses analogous to asthma in people. It is not absorbed after oral administration in horses; therefore, injectable preparations must be used. Metaproterenol is similar to terbutaline, but the duration of action is shorter (4 hours). It is available as 10 and 20-mg tablets and 2 mg/ml oral syrup. The dose in small animals is 0.325 to 0.65 mg/kg. Albuterol is similar to terbutaline and metaproterenol in its action. Doses used in small animals are 20–50 µg/kg up to four times per day. (Dose in people is 100–200 µg/kg four times daily.) It is available as 2, 4, and 5-mg tablets and 2 mg/5 ml syrup. Levalbuterol is the R-isomer of albuterol, and is used in some products. The proposed benefit of administration of levalbuterol compared to the R-, S-racemic mixture of albuterol is that the S-form may have bronchoconstrictive and proinflammatory properties, and a pure R-isomer avoids these problems (Reinero et al., 2009). In cats, the regular use of the racemic form (R and S) of albuterol increased airway inflammation. Therefore, these authors and others have questioned the regular use of racemic albuterol in cats for treating feline asthma (Reinero, 2011; Trzil and Reinero, 2014). On the other hand, in a study in horses, administration of levalbuterol to horses with RAO did not result in greater bronchodilation compared to albuterol (Arroyo et al., 2016). Levalbuterol was an effective bronchodilator in these horses, but the short duration of action is impractical for long-term treatment of RAO. Clenbuterol was approved by the FDA in 1998 for use in horses. Its use in food animals is illegal, which is discussed in the section on prohibited drugs in food producing animals. Its use has not been reported in small animals. Clenbuterol has been used primarily for the treatment of recurrent airway obstruction (RAO) (Box 48.1) in horses and some studies have demonstrated efficacy (Shapland et al., 1981). However, its bronchodilating ability has been questioned. Compared to other β agonists, such as terbutaline, clenbuterol has lower clinical efficacy because it is only a partial agonist and has lower intrinsic activity (Törneke et al., 1998; Derksen et al., 1987). Clenbuterol is available as a syrup (Ventipulmin® syrup, 100 ml and 33 ml bottles, 72.5 µg/ml) for horses for oral administration. The dose is 0.8 µg/kg twice daily, but can be increased to 2×, 3×, and then 4× (up to 3.2 µg/kg) if the initial dose is not effective. The duration of effect is 6–8 hours. Prolonged administration is discouraged (Mazan, 2015; Ivester and Couëtil, 2014). Prolonged administration can diminish the effect through receptor tolerance or tachyphylaxis (desensitization of receptors) (Read et al., 2012). Adverse effects in horses include sweating, muscle tremor, restlessness, and tachycardia. There is conflicting evidence on the effect of clenbuterol on exercise and muscle physiology in horses. One study indicated no improvement in aerobic metabolism of horses (Ferraz et al., 2007). On the other hand, it may have a negative ergonomic effect due to decrease in aerobic capacity, time to fatigue, cardiac function, and oxygen consumption. It has an anabolic effect on skeletal muscle but the anabolic effects do not produce greater athletic performance. One study showed that less than 2 weeks of treatment at approved doses did not reveal any adverse effects on equine cardiac or skeletal muscle (Thompson et al., 2012), but a study in rats (Huang et al., 2000) showed that down-regulation of β2 receptors occurs after 10 days of treatment and results in a decrease in receptor expression in skeletal muscle (35% decrease) and lung (45%). It appears that the effects on equine performance may be controversial, but there is agreement among equine specialists that clenbuterol should not be used solely to enhance athletic performance (Carlos and Davis, 2007). Clenbuterol is banned from use in food-producing animals, but has been used in livestock illegally as a repartitioning agent. These agents repartition nutrients away from adipose tissue in favor of muscle (Peters, 1989). The repartitioning and anabolic effects are caused by leptin- and adiponectin-mediated effects that produce an increase in muscle mass (Kearns et al., 2006). In food animals, the result is increased carcass weight and an increased ratio of muscle to fat, while reducing the feed required per kg of weight gain. A review of the use of β-adrenergic agonists as growth-promoting agents is discussed in the article by Mersmann (1998). Clenbuterol residues in food products pose a threat to people (e.g., pregnant women and people with heart conditions). Deaths in humans have been reported as a result of eating beef liver contaminated with clenbuterol residue, making the illegal use of clenbuterol in food animals a regulatory concern to the FDA and other international agencies. The US FDA has established a “zero tolerance” for residues (Chapter 52). In the USA, clenbuterol residues have been highest in competition “show animals”. Another unapproved use of clenbuterol is the abuse by people. It is used (abused) for “muscle building” in human athletes and for weight loss. Also called “Clen”, it can be found widely on the internet, promoted for use in people for weight loss and muscle development. Zilpaterol (Zilmax®) is another β agonist that has been used in cattle. This is an FDA-approved feed supplement for cattle that has been used by cattle farmers globally for nearly two decades to improve cattle’s natural ability to convert feed into lean beef. Zilpaterol is fed at 6.8 grams per ton (90% dry matter basis) for the last 20 days on the sole ration of feed to provide 60 to 90 mg zilpaterol hydrochloride per head per day. There is a 3-day slaughter withdrawal time. In August 2013, the manufacturer withdrew the medication because of concerns about adverse effects in cattle that included difficulty walking, abnormal gait, and hip pain. Animal welfare concerns were publicized and the company withdrew the medication with plans to reintroduce at a later time. These drugs are long-acting β agonists that have been used in human medicine, but their use in veterinary medicine has not been reported. Inhaled aerosols of β2 agonists are important drugs for treatment of acute bronchospasm in human patients with asthma or allergic bronchitis. Nebulization is the process of creating small droplets of a drug that can be inhaled into the airways. Nebulized agents are delivered via jet nebulizers, ultrasonic nebulizers, and metered-dose inhalers. (Inhalation products are also discussed with the corticosteroids in Section Inhaled Corticosteroids.) Several metered-dose inhalers are marketed for this use in people, such as albuterol (Ventolin®), bitolterol (Tornalate®), terbutaline (Brethaire®), and pirbuterol (Maxair®). The use of these aerosols has become more common in animals because adapters are available to allow for use by animal owners. In horses, masks are available that allow a metered-dose inhaler to be used (Derksen et al., 1996; Tesarowski et al., 1994). These devices are available for dogs, cats, and horses. For example, the AeroKat® chamber has also been used to deliver these medications to cats. In 2002, albuterol sulfate was approved by the US-FDA for horses, indicated for relief of bronchospasm and bronchoconstriction associated with recurrent airway obstruction (RAO). It is an aerosol formulation in a pressurized canister delivered via a specialized nasal delivery bulb. The nasal bulb is inserted into the horse’s nostril and when the horse inhales, the device is activated. Masks that fit over the horse’s nose (discussed in Section Inhalant Formulations: Nebulized β Agonists) also have been used. In horses, doses of 360 or 720 µg delivered by aerosolization caused significant bronchodilation (Derksen et al., 1999). The same author also administered pirbuterol at 600 µg/horse with good results. For small animals, the metered dose inhaler to deliver albuterol in people has been adapted for use. However, the use of inhaled albuterol in cats with hyperresponsive airway disease is discouraged because it may exacerbate airway inflammation (Reinero, 2011). The most common adverse effects from β agonists involve the cardiovascular system (tachycardia) and skeletal muscles (muscle tremors) (Fox and Papich, 1989). Cardiac effects (rapid heart rate, palpitations, tremors) can be prominent with β1 agonists and can be produced by β2 agonists at high doses. When high doses of β agonists have been nebulized to horses, muscle twitching, sweating, and excitement have occurred. β2-receptor agonists also inhibit uterine motility and should not be used late in pregnancy. However, these drugs have been used therapeutically for this purpose in situations in which it may be desirable to inhibit uterine contractions to delay labor. High doses of β2 agonists also can produce hypokalemia. As noted above in the clenbuterol section, these drugs also have properties that affect skeletal muscle. These drugs have been abused in humans and animals for their muscle-building properties. High doses can cause muscle twitching and hyperthermia. Regular administration of β agonists can produce drug tolerance, which is a loss in the sensitivity of β-adrenergic receptors owing to down-regulation of receptors. This effect occurs when β-adrenergic drugs are administered regularly for several weeks. Therefore, it is best to rely on these drugs intermittently and allow drug-free breaks in treatment. In animals, the best use of these drugs is for short-term treatment for acute exacerbations of bronchoconstriction and corticosteroids can be used to decrease inflammation for long-term management. Cromoglycate is used occasionally to stabilize mast cells in animals with hypersensitive airways. It is usually administered as a 2% solution for nebulization in the treatment of bronchoconstrictive diseases. It has been used in people to treat asthma and occasionally nebulized to horses via a special mask to treat recurrent airway obstruction (RAO). Cromolyn inhibits mast cell release of histamine, leukotrienes, and other substances from sensitized mast cells that cause hypersensitivity reactions, probably by interfering with calcium transport across the mast cell membrane. It has no intrinsic bronchodilator action. Cromolyn has been used as an aerosol for treating recurrent airway obstruction (RAO) in horses (Thomson and McPherson, 1981) and has improved some clinical measurements in horses with respiratory disease (Hare et al., 1994). It has been administered via inhalation once daily for 1–4 days, which will provide a therapeutic effect for several days. In one report, nebulization of 80 mg once daily for 1–4 days prevented signs of heaves in horses for up to 3 weeks. The recommended dose is 80 mg of a 0.02% solution from an ultrasonic nebulizer, or 200 mg from a jet nebulizer (Couëtil et al., 2016). However, other studies have produced conflicting results. The primary drawback is the route of administration and timing. To be effective, it must be administered before the horse is exposed to the allergen (prophylactically). This agent has its effects primarily on mast cells (Hare et al., 1994). Because airway diseases in animals may have neutrophilic or eosinophilic inflammation, this treatment may have limited impact unless mast cells contribute substantially to the disease. Nedocromil sodium (Tilade) is a chemically unrelated drug, but has an antiinflammatory effect via a similar mechanism to chromolyn. It is approved for use in treating asthma in people but use in veterinary patients has not been reported. The methylxanthines include theobromine, caffeine, and theophylline. Pentoxifylline also is a methylxanthine, but is used for conditions other than asthma. The methylathines – particularly theophylline – have been used as bronchodilators, but the use is no longer common. Once a mainstay in the therapy of human asthma, theophylline use has diminished because of a high incidence of side effects in people and because there are better-tolerated and more effective drugs available. However, occasionally in small animals theophylline is used because it is tolerated well and oral dosing is convenient. There is occasional use of theophylline in horses (and pentoxifylline for other diseases), but the use of theophylline in horses is hampered by adverse effects and racing restrictions. There are no clinical studies that have documented efficacy in small animals; the use is purely anecdotal and doses based on pharmacokinetic studies. The methylxanthines are classified as CNS stimulants, but they have a variety of pharmacological effects on various organ systems (Weinberger and Hendeles, 1996). The methylxanthines relax bronchial smooth muscle and produce antiinflammatory effects that are unrelated to the bronchodilating properties. In addition, there are nonrespiratory effects of methylxanthines, which include CNS stimulation, urinary diuresis (mild), as well as cardiac stimulation (mild). The cellular basis of action of the methylxanthines is still not completely understood. The effect reported in the literature is the action to inhibit phosphodiesterase types 3 and 4 (PDE-3, PDE-4). Phosphodiesterase is the enzyme that catalyzes cyclic-AMP to inactive products. Inhibition of the phosphodiesterase enzyme increases intracellular concentrations of the cyclic nucleotide cAMP. Cyclic-AMP inhibits the release of inflammatory mediators from mast cells, has antiinflammatory effects, and produces bronchial smooth muscle relaxation (Barnes, 2003). Another mechanism of action that may explain the benefits for respiratory disease is via antagonism of adenosine receptors. Adenosine causes bronchoconstriction in asthmatic patients, but blocking this receptor also may contribute to the adverse effects. The antiinflammatory effects of theophylline may occur separately – and at lower concentrations – than the bronchodilating effects (Barnes, 2003). This may occur by decreasing the expression of inflammatory genes. Treatment with theophylline has induced a decreased response to histamine and other inflammatory mediators in the airways. Theophylline’s antiinflammatory effects include diminished activity of inflammatory and immune cells – especially eosinophils – in the airways. Eosinophils can play an important role in the bronchial response to inhaled allergens, and they release inflammatory mediators that induce bronchoconstriction and inflammatory changes in the airways. Theophylline has been available in several formulations, including, injectable, aqueous solutions, elixirs, tablets, and capsules. The most convenient for small animal use are extended-release forms that allow for twice daily treatment in dogs and once-daily or once every 48 hour treatment in cats. These are discussed in more detail in Section Clinical Use in Dogs and Cats. However, the availability of these formulations has greatly declined. Because theophylline is uncommonly used in human medicine, and there are no approved veterinary formulations, products for use in animals can be difficult to locate. Examples of other forms of theophylline are presented in Table 48.2. These salts are designed to improve solubility and decrease stomach irritation. The theophylline content of these salts must be considered when calculating a dosage regimen. Table 48.2 Formulations of theophylline After oral administration, theophylline is rapidly and completely absorbed. Systemic availability is 91%, 96–100%, and 100% in dogs, cats, and horses, respectively. Theophylline is metabolized primarily by the liver in all species; only 10% of the dose is eliminated unchanged in the urine. The pharmacokinetics have been studied in several veterinary species and are listed in Table 48.3 with comparisons to people. Table 48.3 Theophylline pharmacokinetics and doses Theophylline drug plasma assays are available in many laboratories. When theophylline is used long term, monitoring is recommended to establish the optimum dose. In dogs and cats, the accepted therapeutic plasma concentration is 10 to 20 µg/ml, but these values were extrapolated from human studies. In animals, we aim for plasma concentration greater than 10 µg/ml (usually 10–20 µg/ml) for clinical effects. Plasma concentrations greater than 15 µg/ml have been reported to cause clinical adverse effects in horses. Antiinflammatory action may occur in patients at concentrations that are below the concentrations usually considered for bronchodilation. For example in people, a concentration of 6.6 µg/ml theophylline caused a reduction in activated eosinophils (Sullivan et al., 1994). In the review by Barnes (2003) the antiinflammatory effects are reported to occur in people at concentrations of 5–10 µg/ml, which is approximately half of the concentration needed to produce bronchodilation. Mild adverse effects include gastrointestinal signs, which includes nausea and vomiting. More important adverse effects of theophylline are attributed to cardiac stimulation. In this regard, theophylline is more potent than either caffeine or theobromine. The cardiac effects may be summarized as increased heart rate, increase in myocardial contractility (mild), improved right and left systolic function, vasodilation (mild), and diuretic (weak). At high doses, cardiac arrhythmias are possible. CNS stimulation is more likely from caffeine than theophylline or theobromine. However, at high doses, theophylline also can affect the CNS. These are reported less frequently from theophylline use in small animals than in humans, but they can be common in horses. The CNS effects can be characterized by increased alertness, agitation, nervousness, increased activity, and convulsions at high doses. At high doses there is decreased cerebral blood flow (26%), which may be related to the incidence of seizures. Caution is advised after rapidly intravenous dosing as adverse effects have been described when theophylline is rapidly administered intravenously in horses and dogs. If the drug is administered orally, high peak plasma concentrations are less likely (Munsiff et al., 1988). Theophylline relies on hepatic metabolism by cytochrome P450 enzymes for clearance. Therefore, interactions are possible when other drugs are administered that affect these enzyme systems. The metabolism of theophylline may be inhibited by erythromycin, fluoroquinolone antibiotics (for example, enrofloxacin), and cimetidine. The metabolism may be induced by rifampin and phenobarbital, which may necessitate increasing the dose if these drugs are used together. Activated charcoal will increase the clearance of theophylline and other methylxanthines and is useful for treatment of a toxic overdose. Theophylline has been used for the treatment of both cardiac and respiratory diseases in dogs. Some veterinarians have included theophylline in regimens for the management of congestive heart failure, although this has become an outdated treatment because of availability of newer cardiac drugs. (Note that pimobendan and milrinone, discussed in Chapter 21, are also phosphodiesterase inhibitors.) Theophylline is also employed for the management of intrathoracic collapsing trachea and various forms of bronchitis. Older sustained-release tablets have been studied in dogs and cats (Koritz et al., 1986; Dye et al., 1989). However, these formulations were recently discontinued and the dose recommendations no longer apply. Decreased availability of older sustained-release formulations led to the use of sustained-release tablets and capsules. These have now become unavailable to many pharmacies, which has led to a decline in use. If available, oral administration of sustained-release formulations in dogs at a dose of 10 mg/kg, q 12 h will produce concentrations in dogs within a range considered to be therapeutic (Bach et al., 2004). Studies in cats (Guenther-Yenke et al., 2007), showed that a dose of 100 mg per cat of the tablet, or 125 mg/cat of the capsule (approximately 15 or 19 mg/kg, respectively) every 24–48 hours maintained plasma concentrations in a range considered to be therapeutic. Administration of theophylline to horses has a narrow therapeutic index and it is considered less effective than other agents (Box 48.1). Adverse effects have prevented more common use and, because of the CNS stimulant effect, it is banned from performance horses. Despite the risk of adverse effects and questionable efficacy, theophylline has been considered for management of RAO in horses. Studies in the 1980s (Button et al., 1985; Errecalde et al., 1984; Ayres et al., 1985; Ingvast-Larsson et al., 1989; Kowalczyk et al., 1984) summarize the pharmacokinetics and effects of theophylline in horses. Oral doses are preferred to avoid the high concentrations from injections. A loading dose of 12 mg/kg has been followed by maintenance doses of 5 mg/kg, every 12 hours. One study demonstrated that affected horses showed decreased wheezing, decreased respiratory effort, and an improvement in PCO2 and blood pH following theophylline administration. However, none of the horses returned to normal. There also is evidence that phosphodiesterase inhibitors are not effective in horses with recurrent airway obstruction (Lavoie et al., 2006). Theophylline produces a positive cardiac chronotropic effect and a weak diuretic effect in horses. Toxicosis can occur from large doses and doses administrated rapidly intravenously. Toxic signs include tremors, excitement, tachycardia, and sweating. Although there is little clinical experience with the use of theophylline in cattle, experimental evidence suggests that it is a poor bronchodilator in this species (McKenna et al., 1989). Anticholinergic (parasympatholytic/antimuscarinic) drugs such as atropine and glycopyrrolate are effective bronchodilators and N-butylscopolammonium bromide (Buscopan injectable solution) has emerged as an effective agent for horses. (The pharmacology of anticholinergic agents was provided in more detail in Chapter 8.) Cholinergic stimulation causes bronchoconstriction. Anticholinergic drugs inhibit vagal-mediated cholinergic smooth muscle tone in the respiratory tract because asthmatic individuals have excessive stimulation of cholinergic receptors. In some people, COPD can be more effectively treated with anticholinergic drugs than by β agonists (Barnes, 2000). Although atropine will provide relief from these effects in many patients, the side effects are unacceptable for long-term use. Adverse effects involve the CNS and gastrointestinal systems. Pearson and Riebold (1989) compared the effects of atropine, isoproterenol, and theophylline in horses with RAO (Box 48.1). Atropine at doses of 0.02 mg/kg, IV was better at relieving some signs of RAO (reduction in intrathoracic pressure for example) than either theophylline or isoproterenol. Isoproterenol was more effective in this study than theophylline. If anticholinergic drugs are administered, the quaternary amines such as glycopyrrolate (Robinul-V®), propantheline, (ProBanthine®), and isopropamide should be used instead of atropine because quaternary amines are less likely to cross the blood–brain barrier and CNS side effects will be minimized. (This property is discussed in Chapter 46.) Atropine is acceptable for short-term use and a 5 mg IV dose has been used as a response test in horses suspected of presenting with RAO. Ipratropium bromide (Atrovent®) is a quaternary amine that is an effective bronchodilator in human asthmatic patients. It is administered as an aerosol and is the first anticholinergic drug to be approved as a bronchodilator. Since ipratropium is not absorbed from the airways, it is described as a “topical form of atropine.” It has been administered to horses for treatment of RAO and produced effects for 6 hours at a dose of 2 µg/kg. This anticholinergic, antimuscarinic agent is a quaternary ammonium compound approved by the FDA for treatment of some forms of colic in horses (discussed in Chapter 46). Adverse effects include transient tachycardia and decreased intestinal motility. Because of its antimuscarinic action, it has been administered to horses with RAO at a dose of 0.3 mg/kg IV as a single dose. It has a rapid onset (within 2 minutes) and has a short duration of action that inhibits intestinal motility for only 2 hours (Couëtil et al., 2012). The cardiac effects and inhibition of gastrointestinal function prohibit long-term use of atropine. The topical drugs (e.g., ipratropium) have less systemic effects. Because these agents are used in horses, the most important concern is the effect on intestinal motility. Atropine should not be used repeatedly in horses because it can cause ileus. N-butylscopolammonium bromide has become preferred for the acute treatment of bronchoconstriction in horses because when administered once (0.3 mg/kg IV), it was shorter acting, and produced fewer adverse effects, including those on the heart and intestine, than atropine (de Lagarde et al., 2014). Glucocorticoids decrease inflammation associated with inflammatory pulmonary diseases. Prednisolone has been effective for treatment of feline tracheobronchitis (“feline asthma”), nonseptic pulmonary diseases associated with pulmonary infiltrates of leukocytes (noninfectious bronchitis), allergic bronchitis, and recurrent airway obstruction (RAO) and inflammatory airway disease (IAD) of horses. For treatment of asthma in people, inhaled corticosteroids are recognized as the most effective therapy available (Barnes, 1995b, 2006). In one article, they were called the “drugs to beat” (Barnes, 2006), and have become the mainstay of asthma management for people. The pharmacology of the glucocorticoids was discussed in more detail in Chapter 29, with some of the immunosuppressive properties discussed in Chapter 45. In patients with inflammatory airway diseases, glucocorticoids have potent antiinflammatory effects on the bronchial mucosa. Glucocorticoids bind to receptors on cells and inhibit the transcription of genes for the production of mediators (cytokines, chemokines, adhesion molecules) involved in airway inflammation (Rhen and Cidlowski, 2005). In horses with RAO, administration of oral dexamethasone in combination with feeding changes decreased the gene expression of proinflammatory cytokines in bronchoalveolar cells (DeLuca et al., 2008). A decrease in the synthesis of inflammatory mediators such as prostaglandins, leukotrienes, and platelet-activating factor caused by glucocorticoids also may be important (Barnes, 1995a, 1989, 2006). Glucocorticoids have a more pronounced effect on neutrophils and eosinophils than mast cells, which is important because neutrophilic and eosinophilic inflammation has been cited as an important component of equine and feline airway disease (Reinero, 2011; Trzil and Reinero, 2014; Mazan, 2015; Couëtil et al., 2016; Pirie, 2014). Glucocorticoids also enhance the action of adrenergic agonists on β2 receptors in the bronchial smooth muscle, either by modifying the receptor or augmenting muscle relaxation after a receptor has been bound. Corticosteroids may prevent down-regulation of β2 receptors. Glucocorticoids and theophylline used together appear to be synergistic (Barnes, 2003). Oral prednisolone or prednisone is usually the drug of choice when a corticosteroid is needed for treating chronic airway disease. A typical antiinflammatory dosage is 0.5–1.0 mg/kg. After an initial course of treatment many patients can be managed by administering this dose on an every-other-day (EOD) basis. Inhaled corticosteroids also can be administered, such as fluticasone delivered with a metered-dose inhaler (for example, 110 µg twice daily). The inhaled agents are discussed in Section Inhaled Corticosteroids. Because cats are somewhat resistant to glucocorticoids, higher doses have been used compared to doses administered to dogs. Oral prednisolone initial dosages of 2–4 mg/kg/day have been used for 10–14 days, followed by chronic doses of 1.0 mg/kg/day for the treatment of feline asthma (Box 48.2). For cats, prednisolone should be used instead of prednisone because of an absorption/conversion problem similar to horses (see Section Horses) (Graham-Mize and Rosser, 2004). For difficult to medicate cats, some veterinarians have administered 20 mg per cat of a long-acting formulation, methylprednisolone acetate (Depo Medrol®) intramuscularly. The effects of one injection may persist for 3 weeks.
Drugs that Affect the Respiratory System
Antitussive Drugs
Cough Reflex
Mechanism of Action of Antitussive Drugs
Use as preanesthetics:
Morphine
Codeine (Methylmorphine)
Hydrocodone
Available Products
Dextromethorphan
Clinical Use
Formulations
Butorphanol (Torbutrol®, Torbugesic®)
Tramadol (Ultram and Generic)
Bronchodilator Drugs (β-Adrenergic Receptor Agonists)
Mechanism of Action
Stabilize Mast Cells
Increased Mucociliary Clearance
Clinical Use
Short-Acting Nonspecific Bronchodilators
Epinephrine (Adrenaline)
Drug
α1
β1
β2
DA
Norepinephrine (noradrenaline)
+++
1
0
0
Epinephrine (adrenaline)
+++
+++
++
0
Ephedrine
+++
+++
+++
0
Dopamine (Intropin®)
+++
+++
+
++++
Dobutamine (Dobutrex®)
+
+++
+
0
Isoproterenol (Isuprel®)
0
+++
++++
0
Isoetharine (Bronkosol®)
0
+
+++
0
Metaproterenol (Alupent®)
0
+
++
0
Terbutaline (Bricanyl®)
0
+
+++
0
Albuterol, Salbutamol (Ventolin®)
0
+
+++
0
Clenbuterol (Ventipulmin®)
0
+
+++
0
Isoproterenol (Isuprel)
Longer-Acting β2-Specific Drugs
Terbutaline (Brethine®, Bricanyl®)
Metaproterenol (Alupent®, Metaprel®)
Albuterol (Salbutamol) (Proventil®, Ventolin®)
Clenbuterol (Ventipulmin®)
Effect on exercise in horses:
Prohibition to use in food-producing animals:
Other β agonists used for weight gain:
Salmeterol and Formoterol
Inhalant Formulations: Nebulized β Agonists
Albuterol (Torpex®)
Adverse Effects from β Agonists
Tolerance with Chronic Use
Cromolyn (Sodium Cromoglycate) (Intal®)
Mechanism of Action
Clinical Use
Nedocromil Sodium
Methylxanthines (Xanthines)
Pharmacological Effects
Cellular Basis of Action
Antiinflammatory Effects
Formulations
Brand name
Percent theophylline
Theophylline base
Many
100
Theophylline elixir
Many
100
Theophylline monohydrate
90
Theophylline ethylenediamine (aminophylline)
Many
80
Choline theophyllinate (oxtriphylline)
Choledyl®
65
Theophylline sodium glycinate
Asbron®
50
Theophylline calcium salicylate
Quadrinal®
48
Pharmacokinetics
Species
Half-life (hours)
Volume of distribution (l/kg)
Percent absorbed orally
Suggested dose
People
6–8
0.5
90
4 mg/kg q6h
Dog
5.7
0.82
91
9 mg/kg q6–8h, or 10 mg/kg q12h of the extended release formulations
Cat
7.8,11.7 (IV)14–18 (oral)
0.46, 0.86
96–100
4 mg/kg q8–12h or extended-release at 15 mg/kg (tab) and 19 mg/kg (cap) q24h (q48h may be possible in some cats)
Cattle
6.4
0.815
93
20 mg/kg q12h
Horse
12–15
0.85–1.02
100
5 mg/kg q12h
Plasma Concentrations and Clinical Monitoring
Side Effects and Adverse Effects
Drug Interactions
Clinical Use in Dogs and Cats
Clinical Use in Horses
Clinical Use in Cattle
Anticholinergic Drugs
Use of Anticholinergic Drugs in Horses
Drug Choices
N-butylscopolammonium bromide (Buscopan® injectable solution):
Adverse Effects
Glucocorticoids
Mechanism of Action
Clinical Use
Dogs
Cats
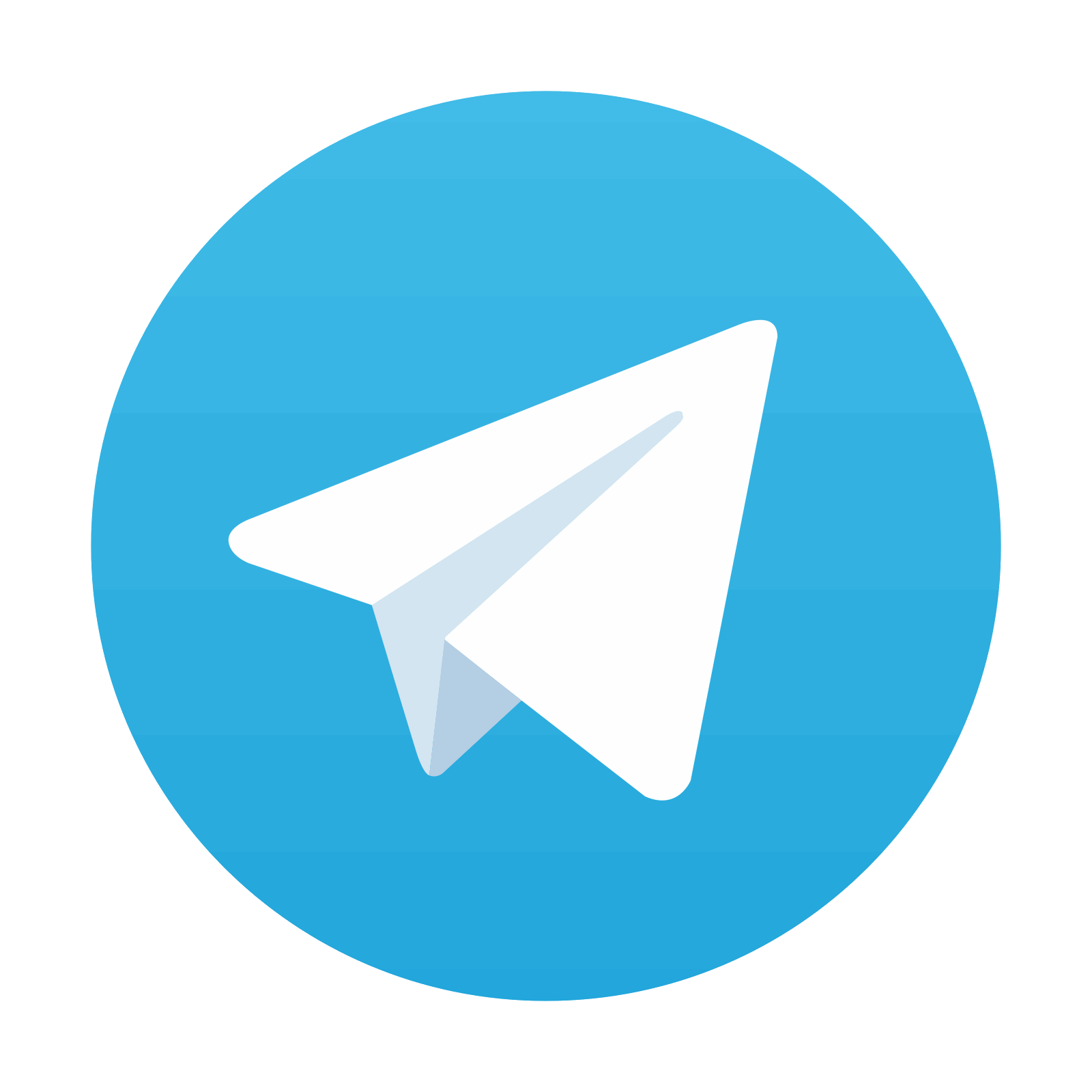
Stay updated, free articles. Join our Telegram channel

Full access? Get Clinical Tree
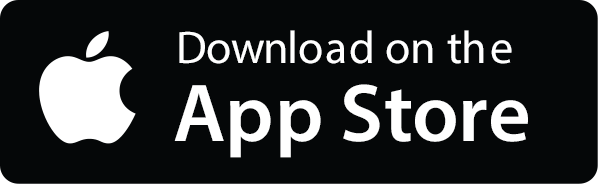
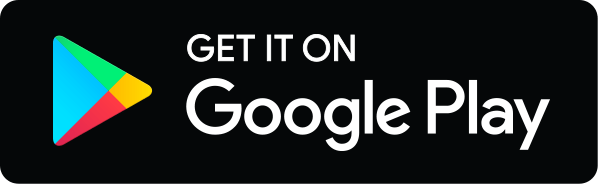