CHAPTER 11 Sean G. Sanders The vestibular system is a component of the nervous system responsible for the maintenance of posture and balance relative to the head and body. This system functions in close coordination with the cerebellum, with portions of the cerebellum providing functions similar to the vestibular nuclei. The components of the vestibular system can be anatomically and functionally divided into those found peripherally (outside of the brain stem) and those found centrally (within the brain stem and cerebellum, Fig. 11.1). Separation of diseases affecting these two main areas is important both for differential diagnosis and prognosis in animals with vestibular system abnormalities. Figure 11.1 Schematic illustration of the anatomy of the ear and the location of the vestibulocochlear receptors in relation to the brain stem and cerebellum. (The Ohio State University. Reproduced with permission.) The vestibulocochlear nerve is the only cranial nerve that does not exit the skull. The sensory neurons of both the vestibular and the auditory portion of cranial nerve (CN) VIII are bipolar neurons with cell bodies located in either the spiral ganglion (auditory) or the vestibular ganglion deep within the bony labyrinth of the petrous temporal bone. The dendritic zones of these neurons are in synaptic contact with specialized hair cells in various receptor organs of the inner ear. The “hair” bundles of these specialized cells are actually stereocilia that function in transforming mechanical deformation (from fluid movement within the membranous labyrinth) into neural signals. Three components make up the bony labyrinth within the petrous temporal bone (Fig. 11.2A and B). These include the three semicircular canals, a vestibule, and the cochlea (which originates in the vestibule). The latter is important for auditory functions. The components communicate with each other and are filled with a fluid resembling cerebrospinal fluid, known as perilymph. These organs comprise membranous structures filled with another type of fluid, known as endolymph. Collectively, the organs are called the membranous labyrinth. Figure 11.2 (A) Schematic illustration of the vestibulocochlear receptors and nerve in the petrosal portion of the temporal bone and their relationship with the facial nerve. (B) Schematic illustration depicting the structures of the vestibular/auditory apparatus. The image at the center depicts the relationship between the vestibular and cochlear receptors. The top image shows the crista ampullaris, the receptor from the semicircular canals, and the bottom image depicts the macula with the statoconia, which is the receptor from the utricle and sacculae. (The Ohio State University. Reproduced with permission.) The semicircular ducts are located in the semicircular canal and are involved with vestibular function. The utricle and saccule are also involved with vestibular function and are located within the large vestibule. The vestibular labyrinths detect either static or kinetic positional signals. The three semicircular canals are oriented at right angles to each other in order to detect angular movements of the head. The receptor of the semicircular ducts is the crista ampullaris (Fig. 11.2B). Like the macula, the crista ampullaris is covered by hair cells that project their cilia into a gelatinous structure known as the cupula. As the endolymph in the semicircular canals moves in relation to outside forces, the cupula moves and deforms the hair cells, which then stimulate the dendritic endings of sensory neurons of the vestibular portion of CN VIII. The crista ampullaris is a “kinetic” labyrinth because it senses the position of the head in any plane and rotational angle. The static labyrinths are the utricle (utriculus) and saccule (sacculus). The receptor of the utricle and saccule is the macula. The macula is covered by hair cells, which are the actual receptor cells. Along the luminal surface of the hair cells are cilia that project into a gelatinous substance known as the otolithic membrane. The otolithic membrane contains otoliths known as statoconia. As the otolithic membrane moves relative to gravitational forces, the hair cells are deformed. This generates an action potential that is propagated through the vestibular portion of the vestibulocochlear nerve (CN VIII). The utricle and saccule are responsible for localizing the static position of the head in space and in linear acceleration or deceleration. Axons from bipolar neurons within the petrous temporal bone (i.e. spiral and vestibular ganglion neurons) enter the cranial vault through the internal acoustic meatus at the cerebellomedullary angle (rostral medulla oblongata). The combination of vestibular and auditory axons at this level compose CN VIII. These axons enter the brain stem at the level of the trapezoid body and caudal cerebellar peduncle. Vestibular axons enter the brain stem and travel in several different pathways. The majority of the axons will synapse on the vestibular nuclei. A small number of axons will bypass the vestibular nuclei and ascend into the cerebellum via the caudal cerebellar peduncle. Some of these axons will synapse on the fastigial nucleus and some of the axons will ascend into the cerebellar cortex into the ipsilateral flocculonodular lobe. There are four vestibular nuclei on either side of the brain stem located in the ventrolateral wall of the fourth ventricle (Fig. 11.3). Axons from these nuclei project to the spinal cord (vestibulospinal tracts) and rostrally within the brain stem (the medial longitudinal fasciculus, or MLF). Fibers that descend the spinal cord are found primarily in the vestibulospinal tracts (primarily lateral vestibulospinal tract) and mainly influence limb extensor tone. The lateral vestibulospinal tract projects from the lateral vestibular nucleus to all levels of the spinal cord in the ipsilateral ventral funiculus (see Fig. 11.4). These axons will then synapse on interneurons in the ventral gray matter of the spinal cord to mediate facilitation of extensor muscles and inhibition of flexor muscles on the ipsilateral side. Figure 11.3 Dorsal aspect of the brain stem. The cerebellum has been removed. M, medial vestibular nucleus; C, caudal vestibular nucleus; L, lateral vestibular nucleus; R, rostral vestibular nucleus. (The Ohio State University. Reproduced with permission.) Figure 11.4 Schematic illustration depicting the course of the lateral vestibulospinal tract. (The Ohio State University. Reproduced with permission.) The MLF travels both rostrally to influence eye position and caudally into the spinal cord. The caudal or spinal limb of the MLF is sometimes referred to as the medial vestibulospinal tract. The group of axons that course rostrally will terminate within the nuclei of CN III, IV, and VI. This circuit influences the position of the eyes relative to the head in space and is responsible for the oculocephalic reflex. The oculocephalic reflex is the physiological nystagmus that is generated when the head is moved from side to side. Within the spinal cord, the medial vestibulospinal tract is located in the ipsilateral ventral medial portion of the ventral funiculus. These fibers, in conjunction with the lateral vestibulospinal tract, are responsible for maintaining the position of the body and limbs relative to the head. Finally, vestibular information is projected to other areas in the brain stem and cerebrum. The vomiting center, located within the reticular formation of the medulla, will receive afferent input from the vestibular portion of the vestibulocochlear nerve. These afferents likely play a role in motion sickness. A final pathway of the vestibular portion of CN VIII will ascend to the cerebrum (involving synapse in thalamic relay nuclei) along with portions of the cochlear nerve to provide a conscious awareness of the body’s position in space. The sensory neurons of the auditory portion of CN VIII are bipolar neurons with their cell bodies located in the spiral ganglion within the bony labyrinth of the petrous temporal bone. The axons project to their respective receptor organs found in the bony labyrinth where their dendritic zones form synapses with mechanoreceptors (hair cells). The auditory and vestibular receptors develop together embryologically and make up the inner ear. Sensory receptors for hearing are located in the cochlea in the organ of Corti. The cochlea is divided into three compartments. The two outside compartments contain perilymph. They are known as the scala vestibuli and the scala tympani. The middle compartment, known as the scala media or cochlear duct, contains endolymph secreted by the stria vascularis (the vascular endothelium lining one wall of the middle compartment of the cochlear duct). Between the cochlear duct and the scala vestibuli is the flexible vestibular membrane (Reissner’s membrane). The cochlear duct contains the basilar membrane, along which the organ of Corti lies. The organ of Corti houses the receptors (hair cells) necessary for audition. The cochlear duct does not communicate with the outside compartments (scala vestibuli and scala tympani); however, the outside compartments communicate with one another through an opening at the apex of the cochlea known as the helicotrema. At the base of the scala vestibuli there is an opening to the middle ear. This opening is called the oval window or vestibular window (although it has nothing to do with vestibular function). The three ossicles (Latin for “little bones”) are located in the middle ear. The first of these bones, the malleus, is attached to the tympanic membrane. As the tympanic membrane vibrates from sound waves in the outer ear, the vibrations are transferred to the malleus. The malleus is attached to the second ossicle, the incus. The incus is attached to the third ossicle, the stapes, which is attached to the oval window. Because ultimately the goal will be to move the fluid in the cochlear duct, and because fluid resists movement much more than air does, the ossicles act as an amplifier of the sound waves traveling through air to overcome the increased pressure necessary to move the fluid. The muscles of the middle ear, the tensor tympani (innervated by CN V), and the stapedius (innervated by CN VII) will reflexively contract in response to loud noises to dampen the activity of the ossicles and prevent damage to the inner ear. It is also thought that these muscles are active during vocalization in order to dampen the sound emanating from the pharynx. As the stapes pushes on the membrane of the oval window, perilymph in the scala vestibuli moves toward the helicotrema and deforms the vestibular membrane. Deformation of the vestibular membrane leads to a deformation of the basilar membrane secondary to compression of the endolymph within the cochlear duct. The distance that the compressive fluid wave travels along the basilar membrane will depend on the frequency of the sound. The basilar membrane is stiff and narrow at the base of the cochlear duct and becomes floppy and wide as it extends to the apex. A high-frequency sound will dissipate quickly at the stiff narrow end of the basilar membrane, while a low-frequency sound will travel far along toward the apex. Therefore, the longer the basilar membrane, the higher the frequency of sound perceived by the animal. Dogs have much longer basilar membranes than humans and are therefore able to “hear” higher-frequency sounds (such as a dog whistle). The area of the basilar membrane that is maximally deformed by the fluid wave will establish a place code for that frequency and produce maximal activation of the hair cells sitting atop the basilar membrane. The deformation of the hair cells will activate the dendrites of the auditory portion of the vestibulocochlear nerve. Fibers from the cochlear portion of the vestibulocochlear nerve leave the spiral ganglion and enter the brain stem at the level of the junction of the medulla oblongata and pons, synapsing in the cochlear nucleus. From the cochlear nucleus, axons will either ascend the brain stem through the acoustic stria or cross midline in the trapezoid body (Fig. 11.5). In the medulla they may synapse in the dorsal or ventral trapezoid, and some will continue on in the lateral lemniscus pathway (pons) to its termination in the caudal colliculus (midbrain). Efferents from the caudal colliculus will cross midline and descend to brain stem lower motor neurons as tectobulbar projections to help mediate brain stem reflexes. Some fibers will join the tectospinal tract, which originates in the rostral and caudal colliculus and descends to the cervical spinal cord in the ventral funiculus. These fibers contain reflex information from both visual and auditory inputs in order to provide reflex movements of the head and neck in response to auditory and visual stimuli. The caudal colliculus is involved in reflex auditory functions. Auditory efferent axons will project from the caudal colliculus to the medial geniculate nucleus in the thalamus to mediate conscious auditory perception. The thalamocortical projections travel from the medial geniculate nucleus in the thalamus through the internal capsule and into the auditory cortex of the temporal lobe (cerebrum). The projections are predominantly contralateral; however, multiple areas of crossing of the pathways occur, so the representation of sound on each side of the cerebral cortex from the auditory system is rather diffuse. Figure 11.5 Schematic representation of the auditory pathway. (The Ohio State University. Reproduced with permission.) The vestibular system functions normally to maintain the animal’s position in space. This coordinated activity of sensory input and reflex output is performed at a subconscious level. The maintenance of balance, posture, and tone contribute to normal equilibrium. The vestibular system is also intimately connected with the extraocular muscles responsible for reflex ocular movements and tracking objects in space. Signs of vestibular disease are usually manifested as unilateral or asymmetric ataxia. With pure vestibular disease, the strength of muscle contraction is normal. Careful observation of the patient in a relaxed environment is very helpful when evaluating an animal for vestibular dysfunction. Allowing the patient to walk around the examination room and become accustomed to its surroundings may provide the investigator with important clues. Excessive barking or meowing may be appreciated in deaf dogs and cats. Of primary importance is the differentiation of peripheral from central vestibular disease. Behavioral changes (fear, aggression) or mental status changes (depression, dementia) are signs that indicate a central lesion. A thorough history along with a complete physical and neurologic examination are some of the most important diagnostic tools a clinician can have; however, without an adequate understanding of the anatomy and physiology of the vestibular system, even the most expertly performed neurologic examination is useless if not interpreted correctly. Additionally, false interpretations of a neurologic examination without additional diagnostic tests may lead to the unnecessary euthanasia of animals. A clear understanding of the concept of disease progression over time is also helpful when establishing a list of differentials. The vestibular apparatus can be thought of as contributing equal, tonic input to each side of the head. When the vestibular nuclei are excited in the brain stem, there is ipsilateral facilitation of extensors and contralateral facilitation of flexors in the muscles of the limbs and trunk mediated via the vestibulospinal tracts. When the system is functioning correctly, the two opposing vestibular systems (left and right) balance each other out and the body’s position in space is maintained at equilibrium. If a lesion prevents the activation of one side of the vestibular apparatus, the ipsilateral vestibular nuclei will not be excited as much as the functioning, contralateral side. The imbalance in the system will cause the relative facilitation of extensors on the normal side and the lack of facilitation of extensors on the side of the lesion. Therefore, the body will be “pushed” by the normal extensors in the direction of the abnormality. This is manifested clinically as a head tilt, circling, leaning, falling, or rolling to the side of the lesion (Fig. 11.6). Figure 11.6 Two dogs displaying head tilt of different severities. (A) Mild left-sided head tilt. To facilitate visualization, draw an imaginary horizontal line at the center of the eyes. (B) Severe left-sided head tilt. The severity of the head tilt has no relationship with lesion localization, i.e. central or peripheral vestibular disease. Because the vestibular system also contributes to the generation and maintenance of the oculovestibular reflexes, abnormalities of movements of the eyes can be very helpful clinically when trying to localize a lesion. When the head is rotated, the brain will try to keep the eyes focused on the visual field in order to keep the visual stimulus centered on the retina for optimal visual acuity. To perform this reflex activity, there is vestibular input to the brain stem nuclei of CN III, IV, and VI. As the head is rotated, the extraocular muscles opposite the direction of rotation will contract to “pull” the eyes back toward the center of the desired visual field. Once the muscles reach their limit, the eyes will quickly release and snap back in the direction of rotation and then again slowly move back toward the center of the visual field. This reflex activity is known as the oculocephalic reflex and represents a form of physiologic nystagmus. Nystagmus is a rhythmic, involuntary oscillation of the eyes. In the description above, the slow phase of the nystagmus was opposite the rotation. Nystagmus can have equal movements (no fast or slow phase), which is then referred to as pendular nystagmus, or it can have a fast phase and a slow phase, in which case it is referred to as jerk nystagmus. Pendular nystagmus is not a sign of vestibular disease. It is often observed in normal Siamese, Himalayan, and crosses of these breeds as a congenital defect in the visual pathways. When the vestibular apparatus is damaged on one side, there is an imbalance of neural activity in the vestibular nuclei because the vestibular apparatus on the normal side continues to supply the vestibular nuclei with a constant signal. This imbalance is “interpreted” by the brain stem as rotation or movement of the body. A nystagmus will be generated, even though the body and head are stationary, with the slow phase directed toward the side of the lesion. By convention, the nystagmus is described by reference to the fast phase. A nystagmus can be characterized by the position the animal is in when the movement is generated and by the actual movement of the eyeballs themselves. For a postural description, the nystagmus may be spontaneous or positional. With a spontaneous nystagmus, the movement is present when the animal is in a normal postural position. With positional nystagmus, the involuntary eye movements are elicited when the animal is placed in an abnormal position, such as turned on its back or held upside down. The nystagmus may also be described based on the plane in which the eyeballs are moving. The movements can either be horizontal, vertical, or rotary. The nature of the movement (horizontal, vertical, or rotary) is dependent on which semicircular canal is affected. The vertical semicircular canals cancel each other out; therefore, horizontal or rotary nystagmus is seen with peripheral vestibular disease. Because injury to the peripheral vestibular apparatus rarely involves one semicircular canal, usually there are components of both rotary and horizontal nystagmus, and the character may change between examinations. Finally, nystagmus can be described as either conjugate, in which case both eyes are moving in the same direction, or dysconjugate, in which case each eye is moving in a different direction. Strabismus is an abnormal position of the eye. Strabismus is often present in dogs and cats with vestibular disease. This is usually seen as a deviation of the eye ventrally and laterally on the ipsilateral side (Fig. 11.7). This strabismus may be positional (induced when the head is rotated dorsally) or spontaneous (always present). In some cases, the strabismus may be induced upon rotation of the head dorsally, and then the eye slowly returns to the normal position of looking straight up at the examiner. This may be seen with careful observation of both eyes while looking for subtle differences in the position of the globe. This type of strabismus is not due to paralysis of any of the cranial nerves that innervate the extraocular muscles of the eye but due to a disorder of vestibular input. Figure 11.7 Dog with a positional ventrolateral strabismus. This patient was tentatively diagnosed with granulomatous meningoencephalomyelitis. Motion sickness secondary to vestibular function is rarely reported in dogs and cats, but probably occurs, especially in cases of acute vestibular dysfunction. Vomiting and salivation secondary to vestibular disease have been treated with various medications. Some are thought to have weak anticholinergic activity and therefore decrease the firing rate of the neurons at the vestibular nuclei. The vomiting center is located within the reticular substance of the medulla, and there are direct connections from the vestibular nuclei to the vomiting center. It is thought that relief from motion sickness may result by suppressing the activity of the vestibular nuclei. Drugs that have commonly been used are the phenothiazine derivative chlorpromazine (at a dose of 0.2–0.4 mg/kg SQ q 8 hrs in dogs and cats), and the antihistamines diphenhydramine, dimenhydrinate, and meclizine. The phenothiazines are thought to exert their effect through action at D2-dopaminergic, M1-cholinergic and α2-adrenergic receptors. They are not particularly effective in preventing vomiting associated with motion sickness in dogs and cats and it suggested that the effectiveness that is observed may be due to the sedative effects of the drugs. The antihistamines also exert some anticholinergic effect and are far more effective in preventing vomiting associated with motion sickness in people then compared to dogs and cats. A suggested dose for diphenhydramine is 2–4 mg/kg PO or IM q 8 hrs in the dog or cat. Dimenhydrinate can be given at 4–8 mg/kg PO, q 8 hrs in the dog or cat. There are several suggested dosing regimens for oral meclizine: the authors recommend 25 mg q 24 hrs for medium- to large-breed dogs and 12.5 mg q 24 hrs for smaller dogs and cats. None of the above-mentioned drugs is labeled for use in small animals. Maropitant is a veterinary-labeled drug specifically manufactured for the treatment of motion sickness in dogs and cats. It has been shown to be effective in the prevention of vomiting induced by motion sickness in dogs (by 79% of those treated when compared to placebo). Its mechanism of action is through antagonism of the NK1 receptors which bind substance P in the emetic center and in particular at afferent inputs from the vestibular apparatus and cerebral cortex to the nucleus tractus solitarius. Antagonism of substance P receptors is a highly effective means of preventing vomiting induced by emetogenics such as cisplatin, copper sulfate, and apomorphine in dogs and cats. A dose of 8 mg/kg PO q 24 hrs given 1 hr before an anticipated event, which would induce motion sickness, is recommended. Treatment for more than two consecutive days is not recommended. As always, contraindications and adverse side effects of each drug should be reviewed before administration. There are numerous clinical signs that can help the practitioner in localizing a vestibular lesion. The primary goal is to decide whether the problem is within the peripheral vestibular system or the central (brain) vestibular system. An accurate history and physical and neurologic examination are essential to success. The first priority is to determine whether vestibular disease is a likely candidate for the animal’s presenting signs. A key component in the diagnosis of central vestibular disease is the presence of neurologic deficits that cannot be attributed to the peripheral nervous system (PNS) alone. Seizures, neck pain, tremors, and myasthenia gravis are often mistaken for vestibular disease. Animals with vestibular dysfunction will usually present with a primary complaint of head tilt, nystagmus, or ataxia (or combinations of all three). The key to differentiation between peripheral and central vestibular disease is to try to isolate the clinical signs to either involve only components of the peripheral vestibular system or include deficits that could only be explained by a central (brain) lesion. The deficits that may point the examiner in the direction of central vestibular disease include cranial nerve deficits (other than CN VII and VIII), vertical nystagmus, positional nystagmus, behavioral changes, mentation changes, seizures, and the presence of proprioceptive deficits (Table 11.1). Dogs with central vestibular disease are significantly more likely to have nonambulatory tetraparesis compared with those with peripheral vestibular disease. Clinical signs of resting nystagmus and veering or leaning to one side are significantly more common in dogs with peripheral vestibular disease. Table 11.1 Differentiation of peripheral vs. central vestibular disease. A head tilt may be present with either peripheral or central vestibular disease. In the case of peripheral vestibular disease, the head tilt is always toward the side of the lesion. In the case of central vestibular disease, the head tilt may be toward or away from the side of the lesion (see discussion below of paradoxical vestibular disease). Nystagmus that is either horizontal or rotary in nature can indicate either central or peripheral vestibular disease; however, the presence of a vertical nystagmus is highly suggestive of a central disease process. With peripheral vestibular disease, the fast phase of the nystagmus is away from the lesion. With central vestibular disease, the fast phase may be in either direction (toward or away from the lesion). The nystagmus is usually not positional with peripheral vestibular disease. Because animals are able to compensate rather well for peripheral vestibular disease, the nystagmus may only be present for a few days. With central lesions, the nystagmus is often positional, and it may change character (e.g. vertical to rotary) when the head position is altered. Another factor that may help with the differentiation between central and peripheral vestibular disease is the number of beats per minute (BPM) of resting nystagmus. It has been found that dogs with a resting nystagmus rate of ≥ 66 BPM are significantly more likely to have peripheral vestibular disease (median rate = 90 BPM), compared with those with central vestibular disease (median rate = 0 BPM). Additionally, the longer the clinical signs are present, the slower the rate of resting nystagmus (inverse correlation). With bilateral peripheral vestibular disease, a nystagmus (or head tilt) will often not be present. These animals will appear as if they are getting ready to collapse. They will walk very tentatively, with a low, crouched-down stance. Such patients may also display wide, side-to-side head excursions when ambulating. The presence of strabismus is more of a “soft” finding when trying to differentiate peripheral from central vestibular disease. With peripheral lesions, the strabismus tends to be ventral or ventrolateral on the same side as the lesion (ipsilateral). Strabismus is often positional, regardless of whether the causative lesion is peripheral or central. Extending the patient’s neck (moving the head dorsally) often elicits a ventral or ventrolateral strabismus in patients with vestibular dysfunction. With a lesion in the central vestibular system, the strabismus may be deviating in various directions. Dysconjugate strabismus describes a deviation of both eyes but in different directions. This is an uncommon finding and is usually associated with central vestibular disease. The facial nerve (CN VII) enters the internal acoustic meatus of the petrosal bone and courses through the facial canal to the point where it exits the skull (stylomastoid foramen) dorsal to the tympanic bulla. Because of its intimate contact with the peripheral vestibular system, disease processes such as otitis may affect facial nerve function. This may be manifested as complete or partial facial nerve paralysis or spasm. The sympathetic innervation to the eye also passes in close contact with the structures of the middle and inner ear. Therefore, the sympathetic system may be affected by peripheral vestibular disease and clinical signs of ipsilateral Horner’s syndrome (ptosis, enophthalmos, miosis, protrusion of the third eyelid) may be present. Deficits in facial nerve function or sympathetic innervation to the eye suggest a peripheral lesion, especially in the face of otitis or trauma to the same side and absence of conscious proprioceptive deficits. The presence of cranial nerve deficits other than the facial or vestibulocochlear nerve is suggestive of a central problem. Alterations in mental status or level of consciousness may support a diagnosis of central vestibular disease based on the notion that vestibular projections to the reticular substance in the brain stem may be affected along with the neighboring ascending reticular activating system. The single strongest sign of central vestibular disease is the presence of conscious proprioceptive deficits. It is common to mistake ataxia for proprioceptive deficits. Ataxia may be present with both central and peripheral vestibular disease. Because the proprioceptive pathways do not come into contact with the peripheral vestibular system, alterations of conscious proprioception are consistent with a central lesion. Portions of the cerebellum add to the maintenance of posture; therefore, cerebellar signs, along with vestibular signs, suggest either brain stem and/or cerebellar dysfunction. Clinical signs attributed to the forebrain (e.g. seizures, vision loss, behavioral or mentation changes), along with vestibular signs, suggest a multifocal or diffuse disease process. Paradoxical vestibular disease refers to lesions of the central vestibular system in which the head tilt is away from the lesion. The cerebellum normally sends inhibitory efferent projections through the caudal cerebellar peduncle to the ipsilateral vestibular nuclei. If there is a lack of inhibition to the vestibular nuclei on the same side as the cerebellar lesion, the vestibular nuclei will be disinhibited, and through a mechanism similar to that discussed previously, there will be greater facilitation of the extensors on the ipsilateral side and facilitation of the contralateral flexors. Therefore, the body will lean away from the side of the lesion, and the head tilt will be in the opposite direction. In these cases, the fast phase of any resulting nystagmus will be toward the lesion. In many cases there will also be asymmetric conscious proprioceptive deficits. The lesion can be localized to the same side as the worst conscious proprioceptive deficits. Paradoxical vestibular disease can be seen with lesions of the flocculonodular lobes of the cerebellum, the caudal cerebellar peduncles, and the rostral and medial vestibular nuclei in the medulla. Occasionally, abnormalities in the dorsal nerve roots of C1–C3 will produce a paradoxical head tilt, and, if present, conscious proprioceptive deficits will localize the lesion to the most severely affected side. The ear canals of dogs and cats open within the second week of life; however, the complete maturation of the auditory system does not occur until 6–8 wks of age. Some estimates have put the maturation date at 4–8 wks. When assessing hearing in dogs and cats, it is better to err on the conservative side and wait for complete maturation of the auditory apparatus. The entire auditory pathway, including the cerebral portions, is probably not completely matured until 12 wks of age. The clinical assessment of a suspected deaf animal can be difficult. Bearing this in mind, it is important to remember that all animals may react to vibratory clues (slamming a book on a table or shutting a door) or visual cues (clapping hands). Therefore, one should endeavor to stay out of the animal’s visual field when trying to elicit an auditory perceived response. Complete hearing loss can be a challenge to assess clinically, and partial or unilateral hearing loss is even more difficult to ascertain. Central deafness, or deafness due to an abnormality in the brain stem without concomitant signs of diffuse neurologic disease, is rare. Because there are multiple points along the auditory pathways from the cochlear nuclei to the thalamus that cross, the auditory pathways are well represented bilaterally within the brain stem. It would therefore take a severe injury or disease process in order to completely disrupt auditory function. A process of that degree would certainly manifest itself with other signs of severe brain-stem disease. Therefore, complete bilateral deafness is usually caused by an abnormality with the auditory receptor (the organ of Corti) and more specifically with the hair cells themselves. The history and signalment of an animal suspected of having auditory dysfunction is very important. Especially pertinent are the breed of the dog and the age of onset when the owner first suspected a problem. This may help differentiate congenital deafness from acquired deafness, which may affect breeding programs. Other signs of neurologic disease, especially vestibular disease, are important to document, as well as any history of trauma to the head or ears, use of potentially ototoxic drugs or compounds, and any previous or current clinical signs of irritation (such as head shaking, odor, or exudates from the external ear canal). There are two types of deafness in dogs and cats: conduction deafness and sensorineural deafness. When the middle or external ear is responsible for hearing loss, such loss is due to a problem with the actual conduction or transmission of the sound waves from the external environment to the cochlea. Blockage of sound wave transmission may be due to occlusion of the external ear canal by ceruminous debris, tissue, or foreign bodies. These blockages may be congenital, as in the case of birth defects, or acquired. Conduction blockage in the middle ear may be due to rupture of the tympanic membrane, fluid or exudate, excessive tissue growth, foreign body, malformation of or damage to the ossicles, or stiffening of the ossicles with age. If the inner ear auditory structures or auditory pathways are compromised, sensorineural deafness may result. Abnormalities of the cochlea, cochlear portion of the vestibulocochlear nerve, auditory pathways in the brain, thalamus, and cerebrum all have the potential to lead to a blockage of the auditory signal transmission and subsequent deafness. In all practicality, almost all cases of pure sensorineural deafness are due to a functional problem with the hair cells of the organ of Corti. There are no degenerative, congenital, or acquired conditions that specifically target the auditory pathways and projections in the brain. Peripheral vestibular disease may be evident in young animals and attributed to a congenital malformation or degeneration of the inner ear structures. If the abnormality is bilateral, these animals may not have a head tilt or nystagmus; however, they will frequently have a symmetrical ataxia, a wide-based stance, and a side-to-side movement of the head in the horizontal plane. The vestibular disease may be present in association with deafness. The clinical signs will usually present when the animals begin to ambulate. They may consist of head tilt, nystagmus, strabismus, ataxia, circling, falling, rolling, and abnormal head movements. Clinical signs associated with the vestibular disease may resolve; however, a head tilt or head tilt that shifts from side to side may persist. Many of the reported animals with this congenital peripheral vestibular disease will compensate for the abnormalities in balance. It is thought that any compensation is centrally mediated, likely through visual mechanisms. If the animal is deaf, the hearing abnormality is permanent. The abnormality has been known to affect German Shepherds, English Cocker Spaniels, Doberman Pinschers, as well as Siamese and Burmese cats. In a report of Doberman Pinscher puppies affected from multiple litters from the same dam, a lymphocytic labyrinthitis was discovered on histological examination. An in utero viral infection was hypothesized to cause the degeneration. No other abnormalities have been documented in affected animals. There is no treatment for the condition. Hypothyroid-associated neurologic dysfunction is well recognized. Specific involvement of the peripheral vestibular system may be the primary presenting clinical complaint. It is usually seen in older animals as an acute onset, nonprogressive presentation. Nearly all animals will have a head tilt and positional strabismus. Many animals also have decreased menace responses and decreased palpebral reflexes. More obvious signs of facial nerve paralysis may also be present. Other clinical signs may be an abnormal gait and circling. Brain-stem auditory evoked response (BAER) testing may be abnormal. There may be other clinical and clinicopathological signs of hypothyroidism (hypercholesterolemia, abnormal thyroid-stimulating hormone response). Hyperlipidemia may be a contributing factor. Labrador Retrievers with severe hyperlipidemia have been described with central and peripheral vestibular signs, tetraparesis, paraparesis, and facial nerve paralysis. Infarction may be an inciting or contributing factor. Other potential causes of vestibular disease must be ruled out, paying special attention to possible structural disease. Because the clinical signs associated with this disease may suggest central vestibular disease, the clinician should be certain a poor prognosis is not given to the owners of the animal without first evaluating and ruling out the possibility of hypothyroidism. The diagnosis may be challenging in that many animals are mistakenly thought to have euthyroid sick syndrome. With an adequate supplementation of levothyroxine, the majority of these animals will return to normal. Neoplastic processes affecting the PNS may result in peripheral vestibular disease by compression or invasion of the vestibulocochlear nerve. Peripheral nerve tumors (i.e. schwannomas) may initially present as peripheral vestibular dysfunction; however, with time they can invade the brain stem. Tumors of the ear (e.g. ceruminous gland adenocarcinoma) may invade the middle and inner ear and cause vestibular disturbances. Other tumors—such as chondrosarcoma, osteosarcoma, fibrosarcoma, and squamous cell carcinoma—have the potential to invade adjacent tissue, resulting in vestibular disease. Treatment is directed at the primary lesion. Supportive treatment for vestibular dysfunction may also be necessary. A condition of idiopathic vestibular disease is well recognized in dogs and cats. Cats of any age can be affected. Dogs tend to be older, and therefore the disease is often referred to as idiopathic geriatric vestibular disease. These vestibular episodes are often confused with vascular accidents (“strokes”) or seizures. The key to this diagnosis is the absence of any detectable structural, metabolic, or inflammatory disease, as well as lack of evidence of central vestibular disease. The onset is acute or peracute, and animals may present with signs of dysfunction ranging from a mild head tilt to severe imbalance and rolling. The clinical signs are usually unilateral, with a horizontal or rotary nystagmus (fast phase away from the side of the head tilt) and an asymmetrical ataxia. The ataxia may be confused with proprioceptive deficits. Animals with idiopathic vestibular disease maintain their strength and mental status. The vestibular signs can be so severe that the affected animals are often incapacitated, making the initial neurologic exam difficult, especially in cats. Most animals will improve rapidly, although complete recovery may take 2–3 wks in a typical case. Improvement is often appreciated within the first 72 hrs. In some animals, clinical signs may persist for up to 5 wks. Occasionally, a mild head tilt will persist after other clinical signs have resolved. The condition can be relapsing. It is thought that this syndrome may result from abnormalities with the endolymphatic fluid of the inner ear structures, a mild intoxication of the vestibular system, or autoimmune disease. There is no specific treatment for the disease. The use of corticosteroids has not resulted in more rapid clinical improvement. Care should be directed at supportive treatment, which may include sedatives, and therapy directed at relieving motion sickness. There is debate as to whether central signs (such as proprioceptive deficits) may be present with this clinical syndrome. Occasionally, in geriatric dogs with this disorder, pelvic limb conscious proprioceptive deficits will be demonstrated on neurologic examination. In many of these cases, the placing deficits are suspected to be due to chronic, subclinical disc protrusions unrelated to the vestibular disorder. However, until there is substantial improvement in vestibular function, or central nervous system (CNS) disease is ruled out, pelvic limb conscious proprioceptive deficits should be considered potential evidence of a central vestibular disorder. The most common cause of peripheral vestibular disease in dogs and cats is otitis media/interna. It has been associated with approximately 50% of the cases of peripheral vestibular disease in older animals. The incidence is much lower in cats. The etiology of this condition is multifactorial. Otitis media/interna may develop from extension of otitis externa across the tympanic membrane, from the nasopharynx via the Eustachian tube, or hematogenously. In most cases, otitis externa is thought to develop as a secondary complication of various disease processes; such disorders include hypersensitivities (e.g. atopy, contact allergy, food allergy), parasites (e.g. Otodectes cynotis, Demodex canis), foreign bodies, and tumors. The most common infectious agents are Staphylococcus spp., Streptococcus spp., Pseudomonas spp., Proteus spp., and Malassezia pachydermatis. Herpes virus infection has been associated with or suspected to be the underlying etiology in several human conditions causing vestibular dysfunction (Ménière’s disease, Ramsay Hunt syndrome). Sensory ganglia are a site of viral latency with possible sources of infection being hematogenous, sensory nerve endings on the body surface, and through the meninges via the endolymphatic duct and cochlear aqueduct. A naturally occurring canine herpesvirus infection of the vestibular labyrinth and ganglion of dogs has been identified. Its role in the possible etiology of vestibular disease in dogs is unknown. However, its presence raises the possibility of it as a potential cause of the symptoms, similar to what is suspected for cases such as idiopathic facial nerve paresis/paralysis and trigeminal neuritis. In addition to infectious otitis media/interna, an apparently noninfectious form of otitis media has been described in Cavalier King Charles Spaniels. This disorder has been termed primary secretory otitis media (PSOM) and denotes the accumulation of a viscous mucus plug within one or both middle ear cavities. These dogs do not have evidence of otitis externa and display clinical signs of discomfort around the head and neck regions, as well as other indications of otitis media (e.g. head tilt, facial nerve dysfunction). Although the pathophysiology of PSOM is unknown, it has been theorized that an increased production of mucus within the middle ear cavity, a decreased drainage of this mucus via an abnormal Eustachian tube, or a combination of these two factors may be involved. A complete, deep otoscopic exam is imperative. Additionally, a myringotomy with associated histopathology, antimicrobial culture, and sensitivity will direct the clinician to a proper treatment regimen. Radiographs or advanced imaging, such as computed tomography (CT) or magnetic resonance (MR) imaging, may confirm involvement of middle and inner ear structures. Occasionally, cases of otitis media/interna may extend centrally. In these cases, advanced imaging techniques such as MR imaging may help the clinician to prognosticate, depending on the character of the lesion, and evaluate potential success of treatment (Fig. 11.8). A combination of surgery (ventral bulla osteotomy) and antibiotic treatment appears to be associated with a favorable prognosis in cases of bacterial otitis media/interna that extend into the brain in dogs and cats. If myringotomy samples are not successful in the diagnosis of an etiologic agent and the animal does not respond to broad-spectrum antimicrobials, a bulla osteotomy may be indicated both as a treatment modality and to arrive at a definitive diagnosis of the etiologic agent. Most cats with polyp-associated otitis media/interna will require a bulla osteotomy for resolution of clinical signs. General treatment of otitis media/interna consists of the removal of any offending foreign bodies, adequate control of parasites, and usually long-term antibiotics or antifungals specifically directed at the offending organism(s) for 3–6 wks or until resolution of clinical signs. Occasionally, animals may need to be treated with medications directed at improving signs associated with motion sickness (e.g. diphenhydramine, dimenhydrinate, maropitant). Any predisposing anatomic conditions may have to be addressed if the condition is chronic or relapsing (surgical resection or ablation of the ear canal). The prognosis for recovery is good if the damage to the receptor organs is not severe. Many animals will be able to centrally compensate for any residual vestibular deficits. Figure 11.8 Transverse T1-weighted MRI (with contrast) of a cat with otitis media/interna that extended into the brain stem. (Sturges and Rosychuk 1994. Reproduced with permission from Elsevier.)120 The same toxic substances that may result in hearing deficits will affect the labyrinthine receptors of the vestibular system (see the “Diseases affecting the auditory system” section below). Animals will usually present with signs of unilateral vestibular disease, although bilateral involvement is possible. The most common compounds are antimicrobials that belong to the family of aminoglycosides, loop diuretics (e.g. furosemide, ethacrynic acid), and ear-cleansing compounds. If toxicity is recognized early, and the offending compound is discontinued, vestibular signs may resolve. Although uncommon, trauma to the petrous temporal bone may cause unilateral vestibular signs. Disruption of the bony and membranous labyrinth will predominate; however, if the injury is severe, other adjacent structures may be affected. It is much more common to have associated brain-stem, cerebellar, or supratentorial signs with trauma to this region of the skull. Ipsilateral facial nerve or trigeminal nerve dysfunction may also result. If the injury is mild or confined to the petrous temporal bone, or the animal heals from associated areas of trauma (brain stem or cerebellum), the prognosis for recovery from peripheral vestibular disease is good, providing central compensating mechanisms are adequate. Any disease process involving the brain has the potential to manifest itself with vestibular dysfunction. Alterations of normal vestibular pathways as a direct result of ischemia, compression, or infiltration will be secondary to the primary offending lesion. There are no recognized diseases of the CNS that specifically target the vestibular pathways; however, some of the disease processes that affect the vestibular structures do so preferentially. Any neoplastic process, whether primary or secondary, may affect portions of the nervous system involved with vestibular functions. Ischemia, compression, and infiltration may all result from neoplasia. The clinical signs associated with the tumor may have an acute onset or be slowly progressive. They may result from a tumor in the immediate vicinity of the brain stem or supratentorial masses that compress the diencephalon and cause secondary compression of the brain stem (either directly from brain parenchyma or via herniation). Tumors of the fourth ventricle—such as choroid plexus papillomas, ependymal tumors, as well as epidermoid and dermoid cysts—may cause compression of adjacent brain-stem structures. Medulloblastomas originating from the cerebellum may affect the brain stem or the vestibulocerebellum. Oligodendrogliomas and astrocytomas may involve parenchyma directly associated with vestibular pathways. The most common brain tumors of dogs that affect vestibular function and associated brain-stem areas are meningiomas and choroid plexus tumors (Fig. 11.9). Meningiomas and lymphomas are common neoplastic lesions in cats. The prognosis depends on many factors, including the location, tumor type, size, and systemic status of the animal. Treatment options include glucocorticoids to alleviate any peritumoral edema, chemotherapy, surgery, radiation therapy, or a combination of all of these (see Chapter 7 for more details concerning brain tumors). Figure 11.9 Transverse, T1-weighted MRI (with contrast) of a dog with a plaque-like meningioma involving the ventrolateral medulla. Canine distemper virus (CDV) may cause a distemper encephalomyelitis. Older dogs that develop distemper encephalomyelitis may present with an altered gait and vestibular disease. These dogs usually have an adequate vaccination history, and a diagnosis of canine distemper encephalomyelitis can be difficult. Cerebrospinal fluid (CSF) may be characterized by a moderate mononuclear pleocytosis that is positive for the antibody directed at the CDV. It is important to realize that a breakdown in the blood–brain barrier (BBB) may result in a false positive test result due to a leakage of systemic antibody into the CSF. More specific information regarding diagnostic testing for CDV infection can be found in Chapter 7. Young dogs affected with distemper encephalomyelitis may recover from their systemic disease. In these cases, the resolution of vestibular signs may follow. Cats infected with the feline infectious peritonitis (FIP) virus will often develop vestibular signs. Cats diagnosed with FIP usually have the dry form of the disease. A diagnosis is based on history, potential exposure, clinical signs, and the demonstration of a high CSF antibody titer directed at the feline coronavirus (see Chapter 7). There is no treatment for the disease, and the prognosis is poor if the clinical signs are severe. Dogs infected with the rickettsial agents causing Rocky Mountain spotted fever (RMSF) or ehrlichiosis may, among other clinical signs, develop vestibular dysfunction. The diagnosis of rickettsial infection will be based on potential exposure to ticks, hematological and biochemical abnormalities, clinical signs consistent with rickettsial disease, a single high titer, and/or a rising titer. Most cases will present between April and October, with the highest incidence in the month of June. RMSF is typically an acute onset infectious disease. Characteristic abnormalities may include anorexia, lethargy, fever, cutaneous lesions, ocular lesions, leukocytosis, anemia, thrombocytopenia, and other signs referable to a vasculitis. Vestibular signs may include a horizontal nystagmus and ataxia. Early recognition and treatment with doxycycline will often result in the complete resolution of the disease and vestibular signs. Meningoencephalomyelitis resulting from primary bacterial infections is an uncommon occurrence in dogs and cats. When suspected, a CSF tap followed by antimicrobial culture and sensitivity, is indicated. A combination of antimicrobials should provide broad-spectrum antimicrobial activity against most organisms if a specific etiology cannot be determined. Animals with an inflammatory CSF sample may benefit from low doses of prednisone (0.5 mg/kg bid). The anti-inflammatory activity of the prednisone may alleviate some of the inflammation responsible for the clinical signs. The dose is low enough that it should not severely inhibit the immune response and may be discontinued early in the course of treatment (see Chapter 7). Other infectious organisms such as Toxoplasma gondii and Neospora caninum can infect the nervous system and result in clinical signs that may include vestibular disease. Other clinical signs such as myalgia (due to myositis), paresis, and multifocal systemic involvement may predominate. Chapter 7 contains more information on the diagnosis and treatment of toxoplasmosis and neosporosis. Fungal infections involving the nervous system may be isolated to neural tissue; however, most animals will show other systemic signs of fungal disease, including cutaneous, ocular, respiratory, and gastrointestinal disease. The most common fungal organism to involve the nervous system of dogs and cats is Cryptococcus neoformans. Coccidioidomycosis may be suspected in dogs with recent respiratory signs. Animals will often recover from a mild respiratory tract infection. In order to make this diagnosis, the animal’s history should indicate that it has been in the Southwest United States. Another fungal infection that usually affects multiple organs is blastomycosis. Multifocal disease, along with any indication for potential fungal infection (travel history, unresponsiveness to antimicrobials, etc.), should give the clinician cause to investigate a possible fungal infection with attempts at direct visualization of the organism from infected tissues, CSF analysis, and serology. The prognosis depends on the duration of the infection and the severity of clinical signs. Fluconazole is the antifungal of choice for cases of cryptococcosis and coccidioidomycosis. Very few animals show improvement with antifungal treatment for nervous system infection of blastomycosis. Disseminated protothecosis has been reported to cause vestibular signs in a dog. Prototheca wickerhamii and Prototheca zopfii have been identified as pathogenic species of algae. Although an uncommon infection, other clinical signs of multi-organ involvement are usually apparent. The prognosis for animals infected with protothecosis is poor. It is thought that granulomatous meningoencephalomyelitis (GME) is a primary autoimmune disease. This disease may represent an immune response to an as yet unidentified organism. There are disseminated (multifocal), focal, and ocular forms of the disease. The distinction between autoimmune disease and neoplasia has been debated. This disease typically affects middle-aged, small-breed dogs. Brain-stem signs including vestibular dysfunction are the primary signs associated with the disease; however, clinical signs would be referable to that portion of the nervous system that is most severely affected. In the vast majority of cases, a definitive diagnosis of GME can only be made on a postmortem examination of affected tissue. The histologic lesions consist of perivascular granulomas comprising primarily dense accumulations of inflammatory cells. Accumulations of histiocytes, lymphocytes, and plasma cells in the nervous system result in compression and invasion of surrounding tissue. A presumptive diagnosis may be made based on CSF analysis, by ruling out infectious etiologies, and by a demonstration of focal or diffuse contrast-enhancing lesions on CT or MR examination. Lesions may not be evident on CT/MR images in GME cases. On CSF examination, there is usually a mixed, predominantly mononuclear, pleocytosis and moderately elevated protein. Most animals will initially show a response to systemic corticosteroids; however, the remission of disease is usually short-lived. Radiation therapy may provide an effective treatment option for dogs with focal GME. Improved survival times for GME have been obtained in recent years with the use of several new treatment options, including procarbazine, cyclosporine, and cytosine arabinoside (see Chapter 7). Metronidazole has been associated with vestibular disease. The onset is acute and usually occurs when animals receive high doses of the medication for a long duration. However, signs of metronidazole toxicity have been seen with treatment for as little as 1 wk and as long as 4–6 wks. There is much debate as to the toxic dose of metronidazole in the dog. Both dogs and cats are susceptible to metronidazole toxicity, and the toxicity is well recognized in humans. Clinical signs of metronidazole toxicity usually show up 7–12 days after continuous high doses (greater than 60 mg/kg/day), but they have also been reported to occur at lower doses. The exact mechanism of the toxicity is unknown. It has been hypothesized that it may interfere with RNA synthesis or possibly inhibit the actions of the primary inhibitory neurotransmitter in the cerebellum and vestibular systems; gamma-aminobutyric acid (GABA). The onset of clinical signs is likely dependent on the dose the animal is receiving. However, clinical signs can also develop after long-term, low-dose therapy. They may consist of generalized ataxia, nystagmus, anorexia, and vomiting. In severe cases, altered mental status, seizures, and opisthotonus may be present. Cats are typically affected by altered mental status and supratentorial dysfunction (seizures, blindness, and ataxia). Clinical signs will usually resolve within 1–2 wks after discontinuation of the drug. Clinical recovery has been shown to be more rapid in dogs that were treated with injectable and oral diazepam compared to those that were not treated. In a treated group, dogs receiving an average dose of 0.43 mg/kg of injectable or oral diazepam every 8 hrs for 3 days had an average recovery time of 38.7 hrs compared to an average recovery time of 11.6 days in those that were not treated. Some changes to the CNS may result in permanent neurologic deficits. Although a very effective antimicrobial, metronidazole is not innocuous. It is recommended that the dosage schedule be individualized for every animal, dependent upon the intended target of the drug. For most applications of metronidazole, a total daily dose of 30 mg/kg body weight (e.g. 10 mg/kg body weight, q 8 hrs) is sufficient. Degenerative processes rarely specifically affect the central vestibular system. However, hereditary polioencephalomalacia results in degeneration of central vestibular pathways (thalamocortical pathway), such as the caudal colliculus, vestibular nuclei, and cerebellar nuclei, amongst other areas of the brain and cervical spine. Cases have been reported in Australian Cattle Dogs and a Shih Tzu. A heritable, rapidly progressive lethal necrotizing encephalopathy similar to human Leigh syndrome (a mitochondrial encephalopathy) has also been reported in related American Staffordshire Terrier dogs. These dogs presented at 6–8 wks of age with clinical signs of neurologic dysfunction referable to the central vestibular system (ataxia, head tilt, intention tremor, strabismus, and nystagmus). This disorder is distinct from another mitochondrial encephalopathy that affects this breed (L-2-hydroxyglutaric aciduria). Vestibular dysfunction may result secondary to ischemic changes or direct trauma to the vestibular pathways. Hydrocephalic animals may have vestibular deficits that are part of the constellation of clinical signs that are commonly present (see Chapter 7). Metabolic disturbances—such as hepatic encephalopathy, altered electrolyte balance, or renal disease—may also cause secondary vestibular signs; however, these abnormalities would not likely cause isolated alterations in vestibular function. Rather, they would result in multifocal signs, of which vestibular signs may be a part. Thiamine deficiency is a nutritional disorder that can affect the vestibular system. The deficiency may result from a diet that is deficient in thiamine, an all-cooked diet, or a diet that contains large amounts of thiaminase (fish viscera). Thiamine is necessary for the completion of the citric acid cycle and therefore is particularly important in tissues that rely on glucose for energy (brain). Thiamine deficiency primarily results in bilaterally symmetrical areas of necrosis, spongiosis, and hemorrhage in the brain stem. In cats, the vestibular nuclei are often affected, resulting in vestibular signs. Cerebellar signs consisting of ataxia, tremors, and absent menace responses may also be seen. Because the heart is also very dependent upon glucose as a fuel source, it too may be affected. Bradycardia, tachycardia, and arrhythmias may be appreciated with thiamine deficiency. If diagnosed early, many animals will respond to parenteral administration of thiamine hydrochloride (vitamin B1). Many animals will have a complete resolution of clinical signs if treated early. Hypothyroidism has been associated with central vestibular dysfunction in a limited number (14) of dogs. Whether the pathophysiology of hypothyroid-related central vestibular dysfunction involves infarction, abnormal neurotransmitter release, or some combination of these and other factors is unknown. All dogs responded favorably to thyroid supplementation. Intracranial epidermoid cysts, while technically tumors, are not neoplastic. They are commonly found at the cerebellomedullary junction but may also arise within the fourth ventricle. It is hypothesized that they arise from fragments of epidermoid tissue at an abnormal location due to a defect in neural tube closure. While the incident occurs around 3–5 wks of gestation, the clinical signs may not be apparent until adulthood. It may be challenging to differentiate intracranial epidermoid cysts from other intracranial cysts with advanced imaging; however, characteristics particular to cystic lesions in the brain with poor or no contrast enhancement in the area of the cerebellar medullary junction and fourth ventricle should be suspected. These lesions have also been described in other areas of the brain. There is one report of a fourth ventricular cholesterol granuloma in a dog. Vascular disease is associated with central vestibular signs. Transient ischemic attacks (TIA) and cerebrovascular accidents (CVA) or strokes are widely recognized in the human population. MR imaging has aided greatly in the detection of CVA events in dogs, particularly in the region of the brain supplied by the paired rostral cerebellar artery and the paired caudal cerebellar artery, both of which arise from the basilar artery. The rostral cerebellar artery supplies the rostral portion of the cerebellar hemispheres, the vermis, and the dorsolateral brain stem. The caudal cerebellar artery provides blood supply to the caudoventral portion of the cerebellum and the lateral medulla oblongata. Ischemic events involving both these arteries have been documented in veterinary medicine. TIAs are a diagnostic challenge considering that even with advanced imaging techniques, such as diffusion-weighted imaging using MRI, the diagnosis is often presumptive based primarily on the time course of the clinical signs (usually less than 24 hrs in humans) and lack of MRI findings. Central vestibular signs may be paroxysmal and are more commonly observed in patients with hypertension, heart disease, hypercoagulable conditions, diabetes mellitus, and hypothyroidism. However, a significant number of documented CVAs have no underlying cause and are therefore defined as “cryptic” or “cryptogenic.” Prognosis for complete recovery with TIAs is good to excellent, whereas that of CVAs is relatively good to excellent but significantly more dependent on the degree of damage to the affected portion of the brain. Time to recover and supportive care of the patient should be encouraged. Due to the close anatomical relationship between the auditory and vestibular receptors, abnormalities of hearing may be observed in conjunction with clinical signs of peripheral vestibular disease. As previously mentioned, a presumptive diagnosis of deafness is easier to make if bilateral disease is present. Congenital aplasia/hypoplasia of auditory receptors Loss of hearing receptors either in utero or shortly after birth is a very common cause of deafness in dogs and cats. This is a pure sensorineural deafness due to degeneration of the hair cells in the organ of Corti. It is thought that an abnormality of the stria vascularis leads to a secondary degeneration of the hair cells. Once lost, hair cells are gone forever. Dogs and cats can be either unilaterally or bilaterally affected with partial or complete hearing loss, respectively. This form of deafness often affects white-colored dogs and cats and merle or piebald-colored dogs. Nonwhite breeds of dogs, such as the Doberman Pinscher, can also be affected. High-incidence dog breeds include Dalmatian, English Cocker Spaniel, English Setter, Australian Shepherd, Australian Cattle Dog, and Bull Terrier. Deafness may also be associated with blue eye coloration. White cats that have two blue eyes have a 50% chance of being born either unilaterally or bilaterally deaf. The incidence of deafness in Dalmatians is reported to be nearly 30%. Congenital deafness has been reported in many breeds (Box 11.1), although not all have been shown to be hereditary. Because the degeneration of the organ of Corti may not take place until 3 wks after birth, it is important to delay testing young animals until their auditory apparatus has matured (6–8 wks). There is a syndrome of congenital deafness and vestibular dysfunction that has been reported in Beagles. Congenital deafness may also be the result of exposure to ototoxic drugs in utero.
Disorders of Hearing and Balance: The Vestibulocochlear Nerve (CN VIII) and Associated Structures
Functional neuroanatomy of the vestibular system22, 27, 34, 45, 67, 86, 122
Functional neuroanatomy of the auditory system15, 27, 34, 45, 90
Clinical evaluation of the vestibular system4, 10, 15, 31, 34, 45, 57, 67, 74, 86, 90, 126
Clinical signs of vestibular dysfunction
Clinical localization of peripheral versus central vestibular disease (Videos 8, 19 and 20)
Neurologic sign
Peripheral
Central
Proprioceptive deficits
No
Usually
Altered mentation
No
Possible
Head tilt
Yes
Yes
Deficits other than CN VII or VIII
No
Possible
Nystagmus
Yes
Yes
Horizontal
Yes
Yes
Rotary
Yes
Yes
Vertical
No
Yes
Positional
No
Yes
Spontaneous
Yes
Yes
Conjugate
Yes
Yes
Dysconjugate
No
Yes
Strabismus
Yes
Yes
Clinical evaluation of the auditory system34, 90, 116
Conduction deafness
Sensorineural deafness
Diseases affecting the peripheral vestibular system4, 7, 9, 15, 19, 34, 36, 41, 48, 55, 61, 64, 65, 66, 68, 71, 80, 82, 83, 86, 92, 104, 105, 113, 119, 122, 128, 129, 137
Degenerative/anomalous
Metabolic
Neoplastic
Idiopathic (Video 19)
Inflammatory/infectious (Video 20)
Toxic
Traumatic
Diseases affecting the central vestibular system4–6, 11–13, 15–18, 20, 24, 26, 28, 29, 32, 34–37, 42–44, 46–49, 54–57, 59–61, 64, 69, 70, 72, 73, 77, 79, 80, 83, 85–89, 91, 94, 97, 100, 101, 103, 104, 106, 112, 119, 121, 123, 124, 128, 129, 134, 136, 138, 139
Neoplastic
Inflammatory/infectious (Video 8)
Toxins
Miscellaneous
Diseases affecting the auditory system1–3, 8, 14, 15, 19, 21, 23, 25, 29, 30, 33, 34, 36, 38–40, 48, 50–53, 58, 62, 63, 66, 69, 71, 75, 76, 78, 81–83, 85, 90, 93, 95, 96, 98, 99, 101, 102, 107–111, 114–118, 122, 125, 127, 131, 132
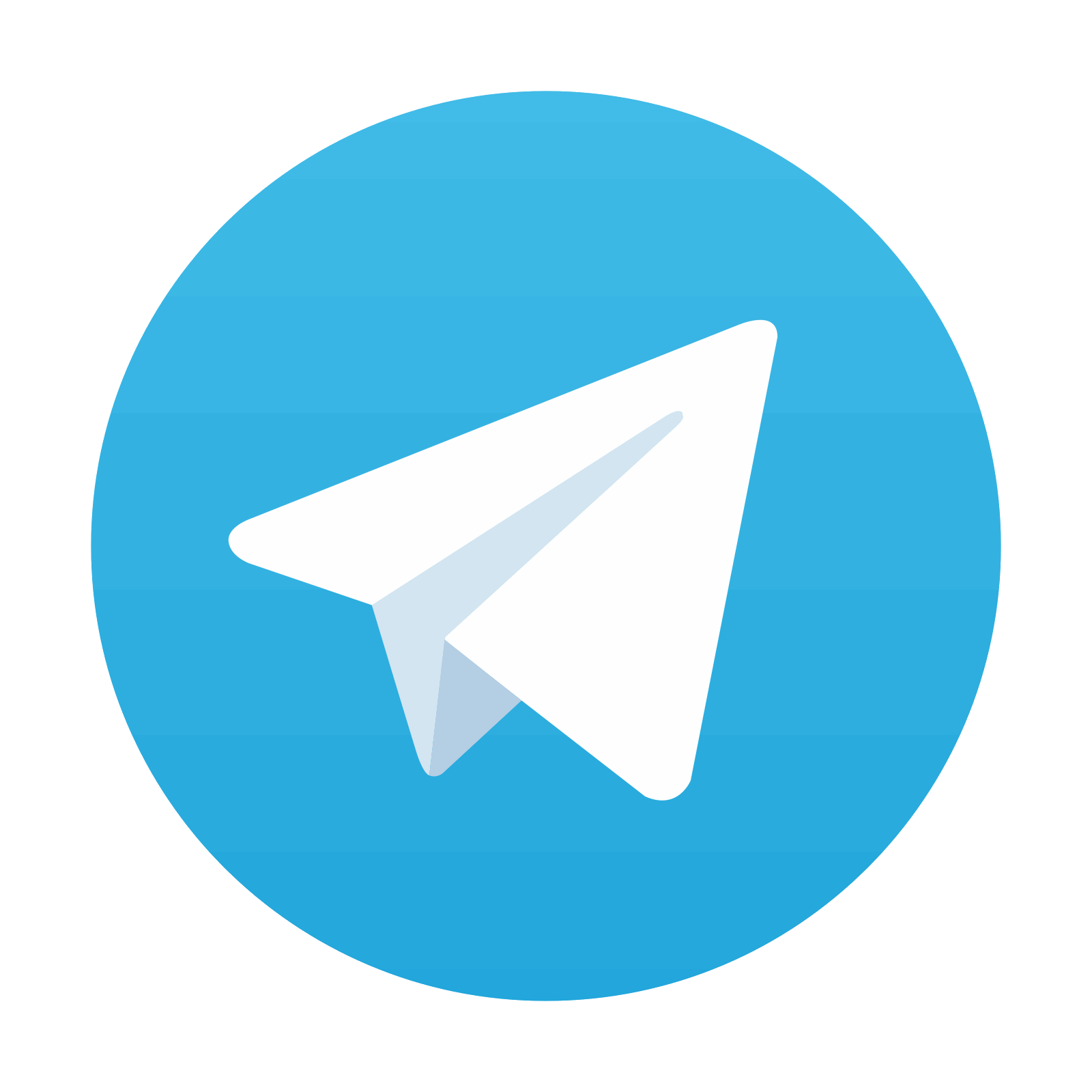
Stay updated, free articles. Join our Telegram channel

Full access? Get Clinical Tree
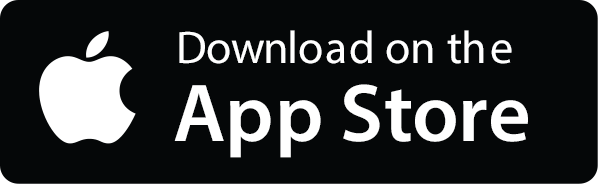
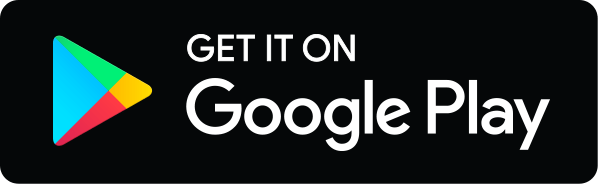