, Monika Hassel2 and Maura Grealy3
(1)
Centre of Organismal Studies, University of Heidelberg, Heidelberg, Germany
(2)
Spezielle Zoologie, Universität Marburg FB Biologie, Marburg, Germany
(3)
Pharmacology and Therapeutics, National University of Ireland Galway, Galway, Ireland
When thousands and billions of cells have to fulfil the task to collectively construct an organism with its complex tissues and organs a manifold of mutual agreements are necessary. Controlling cells, for example organizing centres in early embryos, must emit coordinating signals. In order to respond to the signals cells must have the correct equipment including receptors and downstream intracellular signalling systems, which are cell-type specific.
In addition, control instances are required to monitor:
where and when certain types of cells should differentiate,
how many copies of each cell type should be generated and at which time point proliferation of the progenitors should be restricted or stopped,
when and where programmed cell death has to clear spaces, and
which routes migrating cells, outgrowing nerve fibres and sprouting blood capillaries have to take to reach their targets.
A general view of various means of cell communications is given in Figs. 11.1, 11.2, 11.3 and 11.4. The most important signalling molecules and released signalling cascades are summarized in Figs. 11.5, 11.6, 11.7, 11.8, 11.9, 11.10, and 11.11 under various aspects.
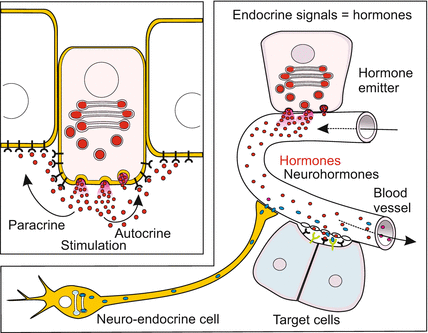
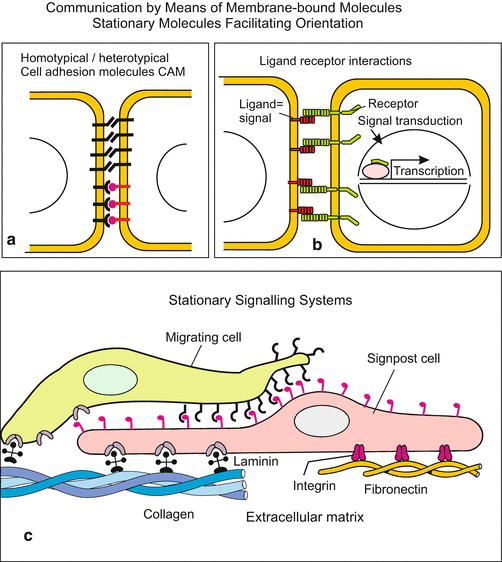
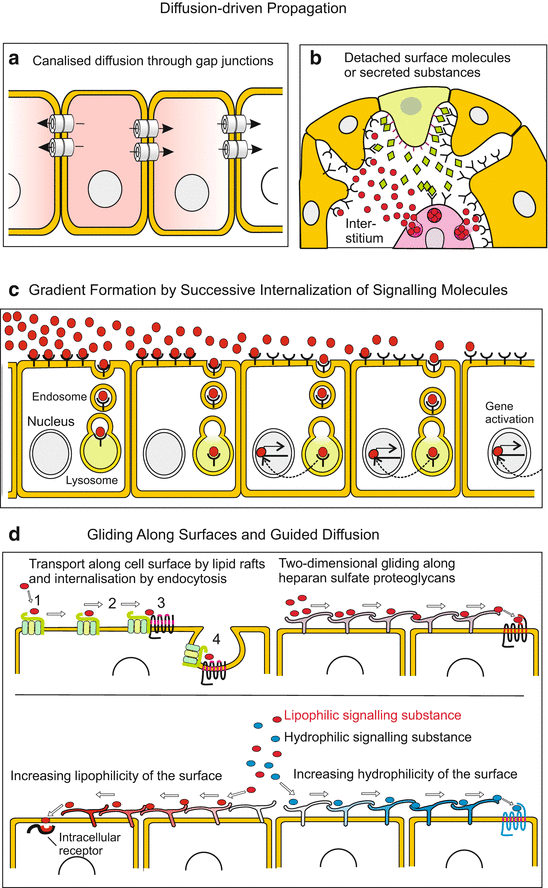
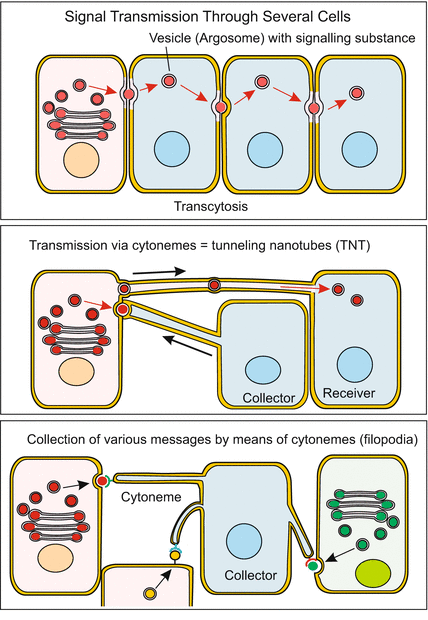
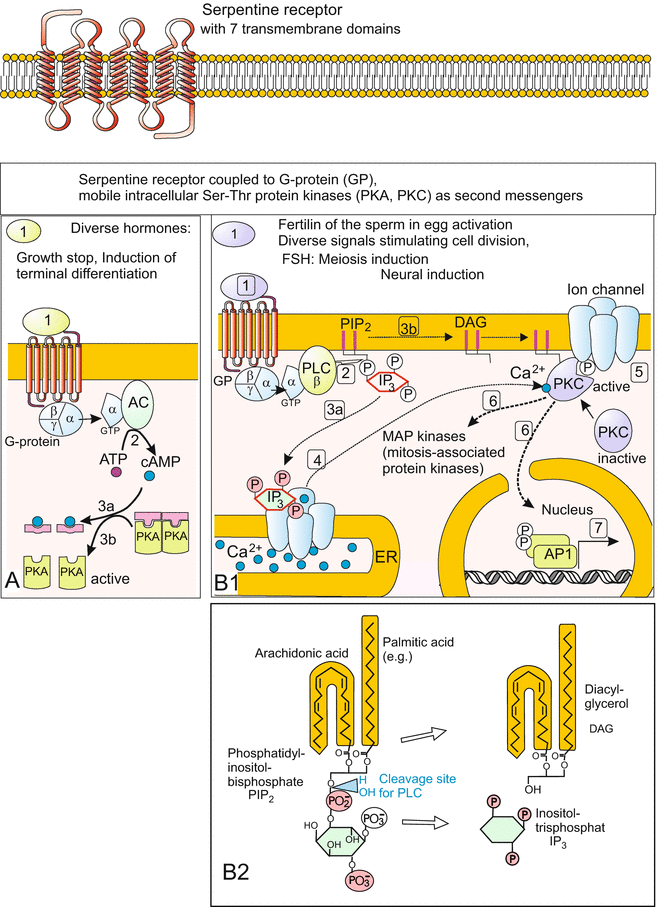
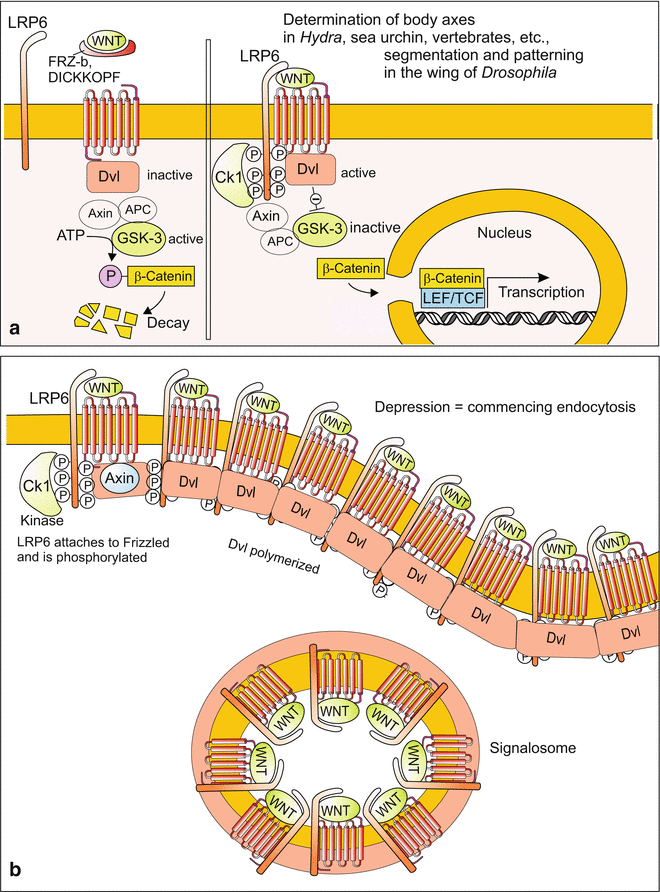
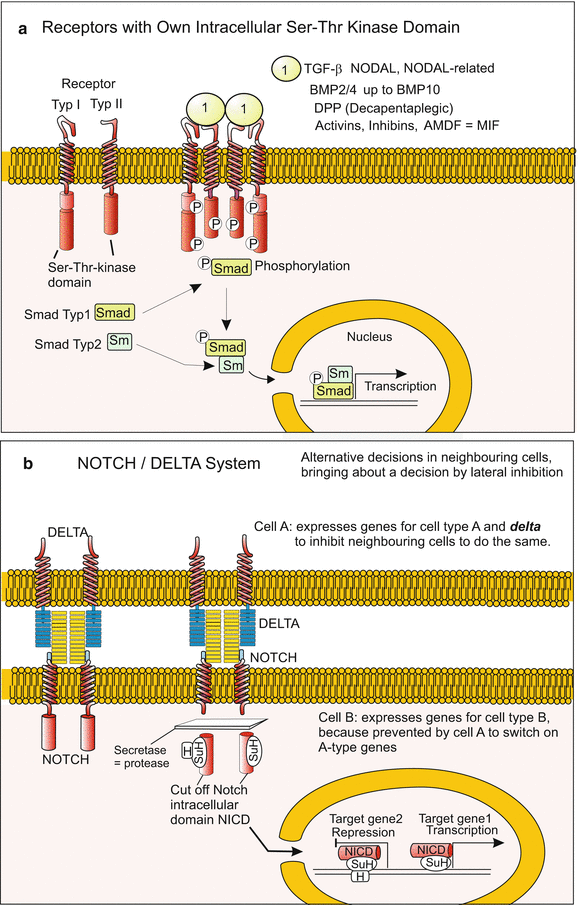
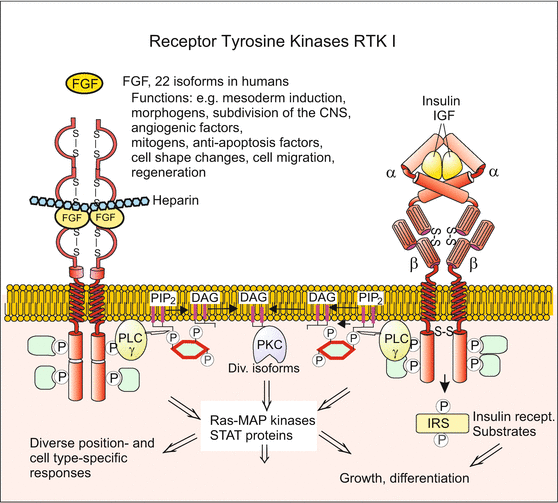
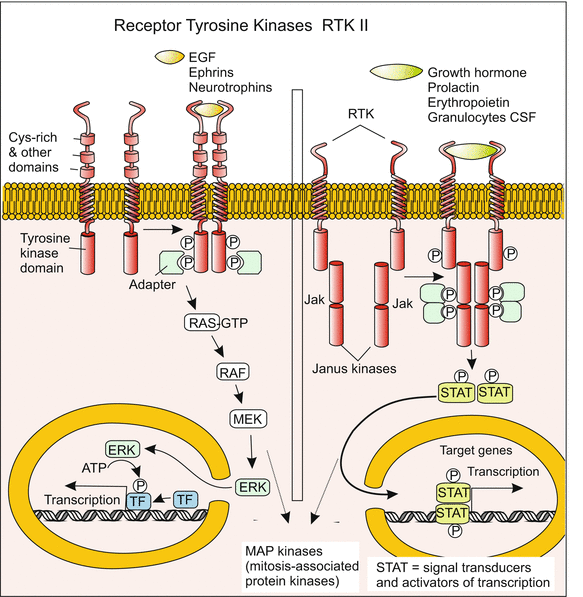
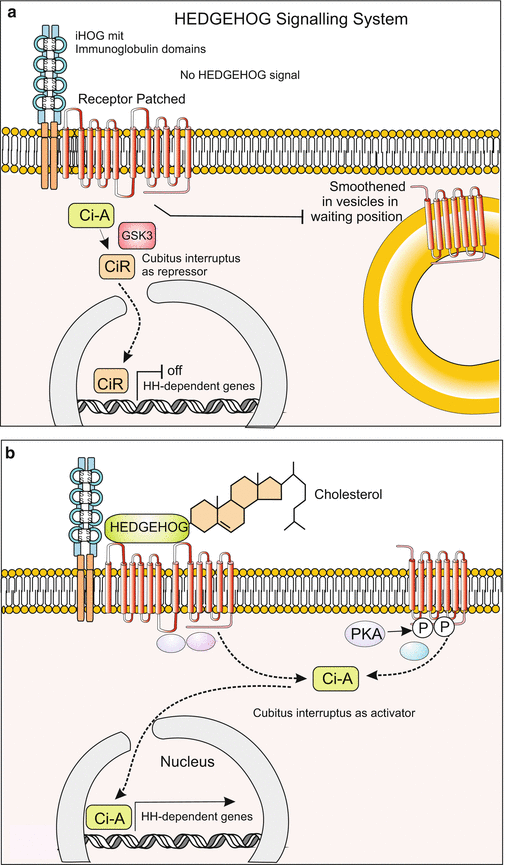
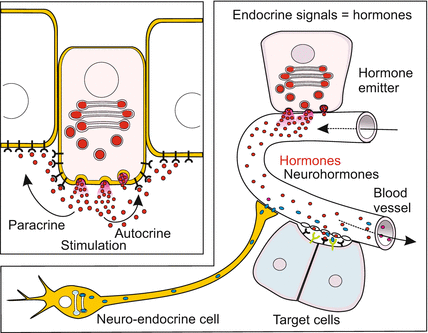
Fig. 11.1
Illustration and terminology regarding propagation of signalling molecules. In autocrine stimulation a cell releases signalling molecules, receives and responds to them itself with enhanced release of the signalling molecule (positive feedback) or by stopping release (negative feedback). The term paracrine stimulation designates dispersal of a factor by an emitting cell and its action in the neighbourhood. The term endocrine secretion is used for the secretion of (neuro-) hormones which are released into the blood or haemolymph and transported to distant targets
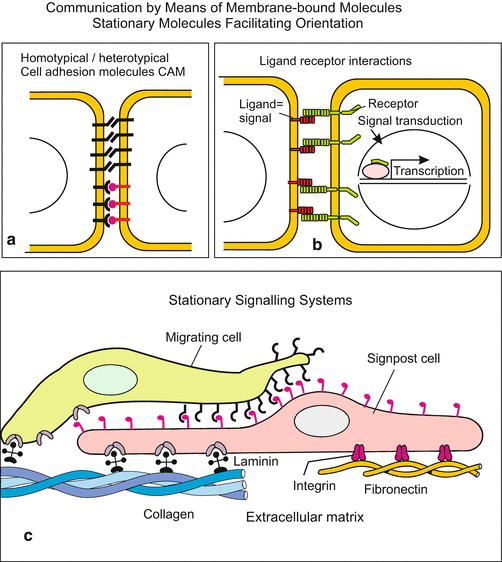
Fig. 11.2
(a–c) Communication between cells mediated by direct contact. (a) Communication by means of homotypic (of the same kind) or heterotypic (different) cell adhesion molecules CAM, or (b) by means of receptor-ligand pairing such as Notch with Delta. (c) Stationary signalling systems enable migrating cells to orient themselves by scanning components of the extracellular matrix or characters on signpost cells. Soluble molecules may enhance attraction
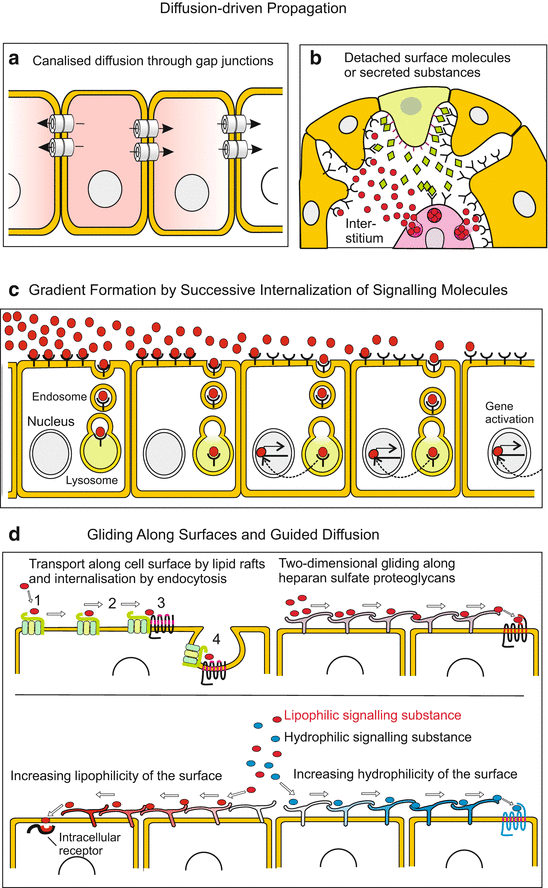
Fig. 11.3
(a–d) Mechanisms of signal transmission. ( a ) Diffusion-driven intracellular spreading through gap junctions or ( b ) extracellular spreading in the interstitial space. (c) Gradients of molecules arise, for example, through binding by antagonists, through enzymatic degradation, or through endocytosis of signal-receptor complexes along a diffusion route, and alternatively by binding of the signalling molecules to the extracellular matrix ECM (for example FGF binds to heparan sulfate proteoglycans). (d) Guided diffusion of signalling molecules is achieved, for example, by receptor binding and transport along cell surfaces, or through attraction or repulsion by hydrophilic or lipophilic cell surfaces
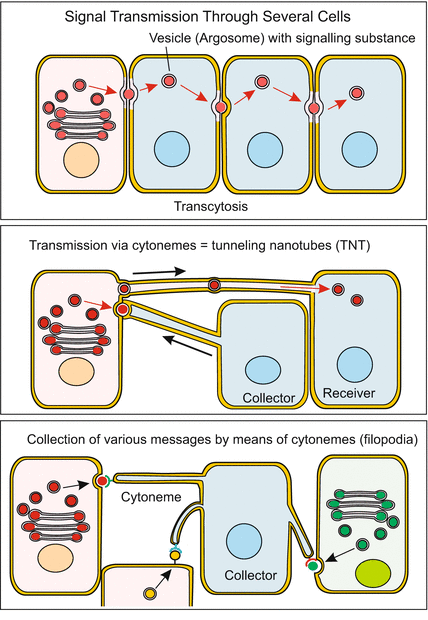
Fig. 11.4
Targeted and directed transfer of signalling molecules. Transcellular transfer is enabled by, e.g., transport vesicles (called by some authors argosomes). These vesicles transport signalling molecules directionally along the cytoskeleton to the neighbouring cell. Transport to distant cells can also happen through tenuous bridges called cytonemes or TNT (Tunneling Nano Tubes) which extend in the manner of filipodia, make contact to adjacent cells and fuse with the membrane of the targeted cell
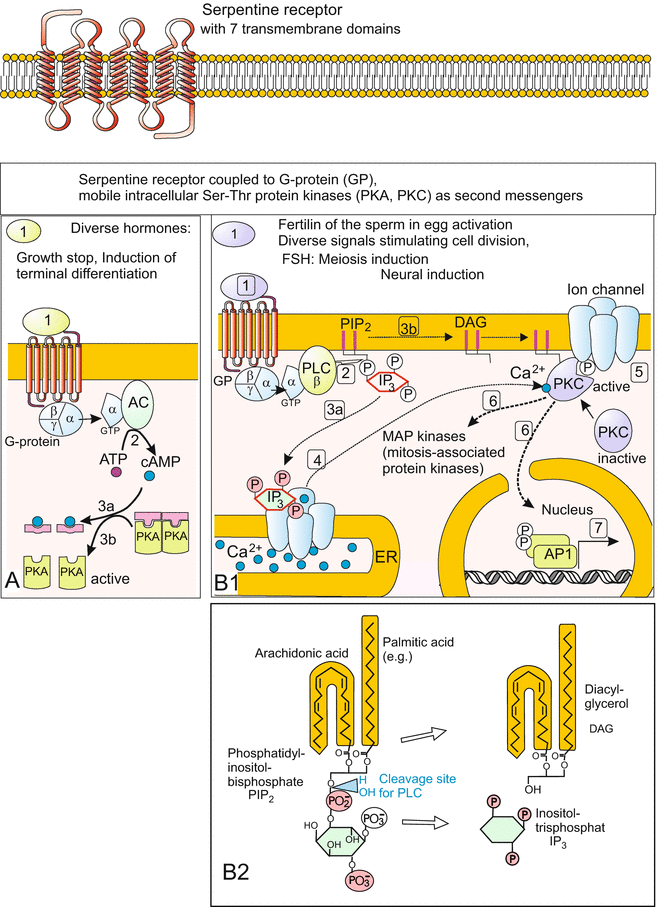
Fig. 11.5
(a, b) Signal transduction 1: Systems with serpentine receptors (seven pass transmembrane receptors) are often involved when peptides act as signalling molecules. The name serpentine receptors points to seven transmembrane helices. Upon reception of a signal a heterotrimeric G-protein binds to the receptor, dissociates in Gα(−GDP) and Gβ/γ subunit; these associate with further proteins, in particular enzymes. (In the illustration GDP and GDP-GTP interconversions are omitted for reasons of simplification.) (a) The Gα(-GTP) subunit activates adenylyl cyclase which catalyzes the conversion of ATP to the second messenger cAMP. cAMP on its part activates protein kinase A by binding and removing the inhibitory component of PKA complexes. PKA (Protein Kinase A) phosphorylates various substrates (actual substrates depending on cell type), in particular enzymes of the carbohydrate metabolism. (b) Activation of the PI-PKC system. Upon binding of G-protein to the ligand-activated receptor the Gα(-GTP) subunit of the G-protein activates phospholipase C (PLC). This enzyme hydrolyses the membrane associated phospholipid phosphatidylinositol-bisphosphate (PIP2) resulting in two second messenger molecules. (1) In the membrane residual diacylglycerol (DAG) recruits a protein kinase C (PKC) to the membrane, where PKC activates diverse proteins (e.g. opens ion channels) through phosphorylation of hydroxyl groups of serine and threonine amino acid residues. In addition, eventually PKC can enter the nucleus to phosphorylate nuclear proteins. (2) Inositoltrisphosphate, IP3, the other second messenger, remains in the cytosol, binds to an IP3 receptor in the endoplasmic reticulum and thereby induces sudden release of calcium ions. These serve as tertiary messengers and co-activate certain subtypes of PKC. The Ca2+-ions induce in different cell types different responses and are pumped back into the ER with lightning speed (compare egg activation in Fig. 3.5). B2 Structure of PIP2 and IP3 in simplified depiction and indication of cleavage (hydrolysis) site of IP3 and DAG from PIP2
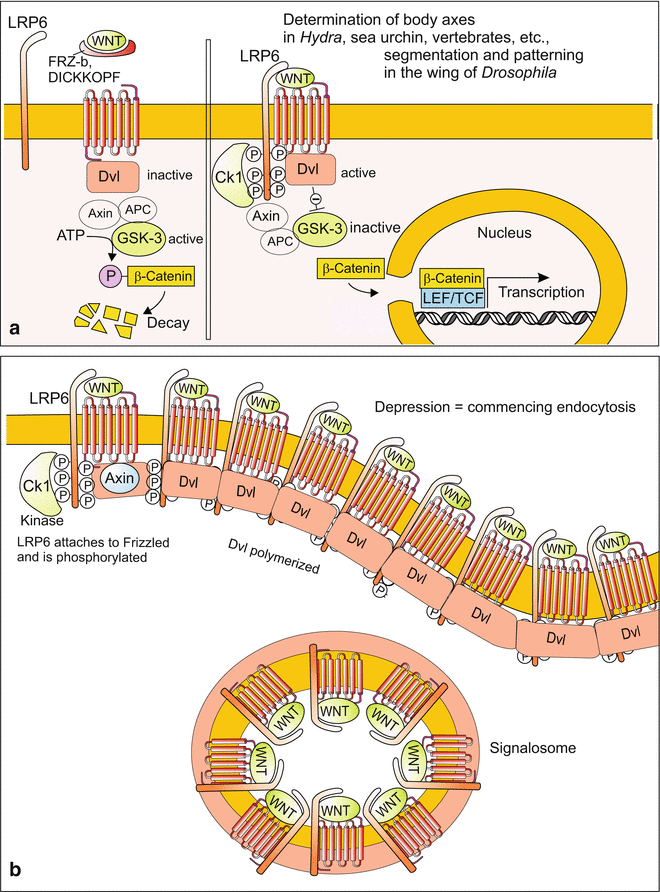
Fig. 11.6
(a, b) Signal transduction 2: The canonical WNT system. (a) Simplified depiction as it is introduced in many publications. If no WNT binds to the receptor FRIZZLED, the kinase GSK3 can unrestrictedly phosphorylate cytosolic β-catenin which thus is subject to degradation by the proteasome. If, however, a WNT signal arrives at the receptor (or GSK3 is inhibited by pharmaceutics), a chain of reactions inactivates GSK3 (chain shortened here). As a consequence β-catenin is no longer degraded, accumulates, enters the nucleus and activates, in cooperation with the transcription factor TCF/LEF, a set of WNT-dependent downstream genes. (b) Model how WNT might be internalized derived from investigations in Xenopus embryos. The transmembrane protein LRP6 attaches on the FRIZZLED receptor the intracellular domain of which is phosphorylated by a kinase. The thus activated FRIZZLED + LRP6 complex recruits DISHEVELLED (Dvl) at its intracellular domain. Adjacent Dvl-molecules polymerize and hold together the complex in form of a vesicle. The vesicle is internalized by endocytosis and called signalosome
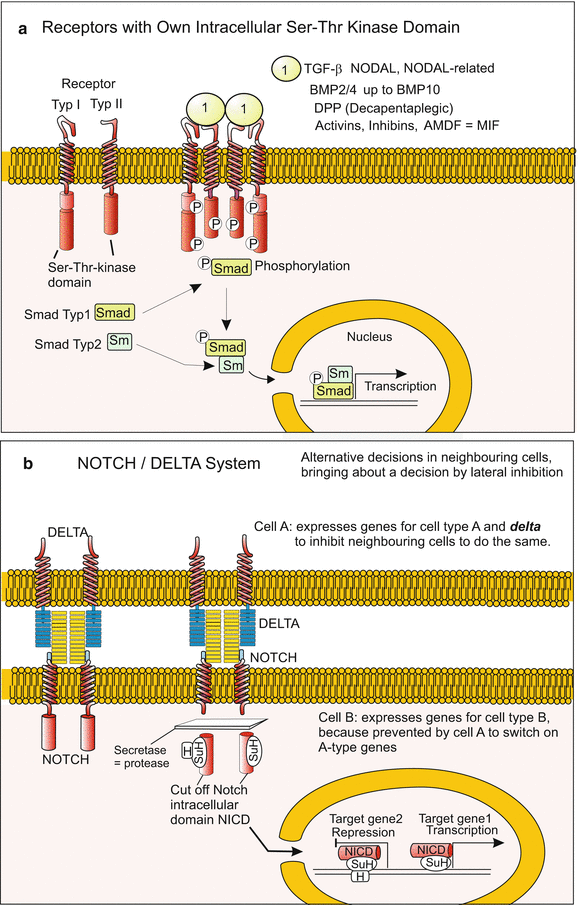
Fig. 11.7
(a, b) Signal transduction 3: (a) Receptors with intracellular Ser-Thr-kinase domain. Such receptors bind, for example, TGF-β, BMP or NODAL arriving from the exterior. They mediate, following multimerization of receptors and SMAD phosphorylation, transduction of the message into the nucleus where transcription of target genes is switched on. (b) The Notch/Delta system, well-known by its function in lateral inhibition and in alternative decisions, is based on the membrane-bound ligand DELTA und its membrane-bound receptor Notch. Upon Delta-Notch binding, the intracellular domain of NOTCH (NICD) is proteolytically cleaved off. NICD enters the nucleus acting there as suppressing or activating transcription co-factor. As a rule, NOTCH and DELTA are exposed on opposite sides of adjacent cells so that the signal is transmitted directionally
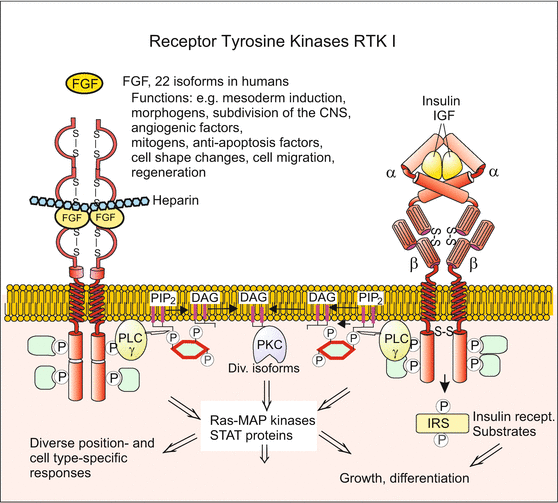
Fig. 11.8
Signal transduction 4: Receptor-tyrosine kinases RTK I dimerize upon ligand binding and activate either a PI/PKC system, the RAS/MAPK pathway, or the PI3-kinase pathway. Whereas the latter induces apoptosis or launches physiological responses, the RAS/MAPK pathway frequently causes changes in the cytoskeleton and thereby in the cell shape, e.g during migration
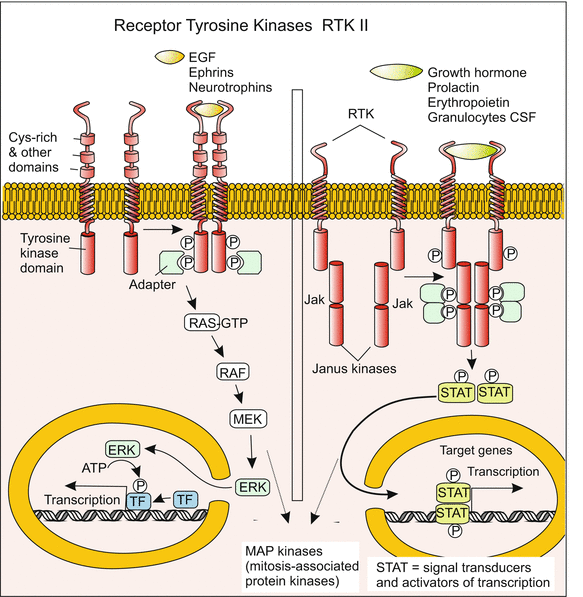
Fig. 11.9
Signal transduction 5: Receptor-tyrosine kinases RTK II activate genes via the RAS-MAPK-ERK-pathway. Alternatively they activate other sets of genes via Janus-kinases and STAT-transcription factors. Result may be, for example, an alteration in the structure of the cytoskeleton or transition of a stem cell into a differentiation path
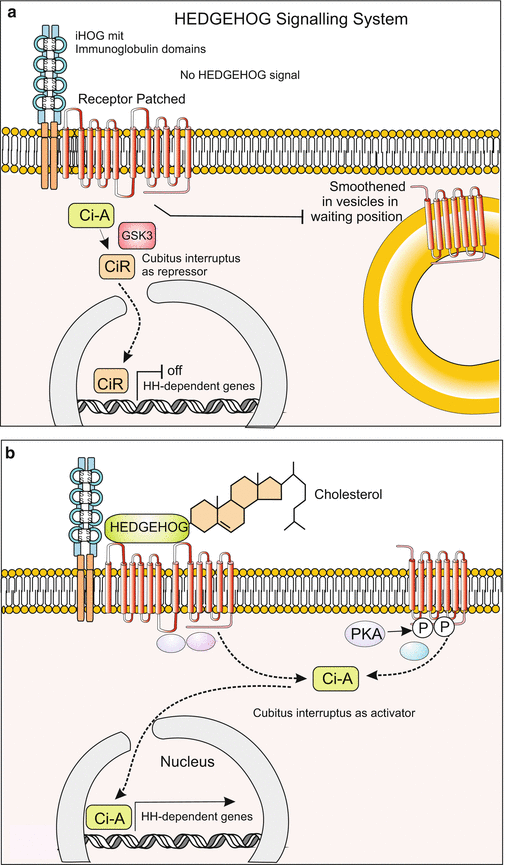
Fig. 11.10
(a, b) Signal transduction 6: The HEDGEHOG system. Ligand is hedgehog, in vertebrates also its homologue SONIC HEDGEHOG. The receptor PATCHED has 12 transmembrane helices and is connected to a further membrane-resident complex, interference Hedgehog (iHOG) the extracellular domains of which exhibit immunoglobulin type repeats. (a) In the absence of a HEDGEHOG signal the transcription factor Ci (Cubitus interruptus) acts as suppressor of hedgehog-dependent genes and the 7-transmembrane protein SMOOTHENED is held in standby condition enclosed in vesicles. (b) Upon binding of the hedgehog ligand, which is covalently linked with cholesterol, to the PATCHED receptor, SMOOTHENED is integrated into the cell membrane. By processes unknown in detail or under debate, the transcription factor Ci is transferred in the active form and taken up by the nucleus. Here it activates genes including the gene patched for the PATCHED receptor (positive feedback)
11.1 Signalling Systems and Mechanisms of Signal Propagation: An Overview
11.1.1 Membrane-Bound Signalling Molecules Convey Messages to Adjacent Neighbours, While Soluble Signal Molecules Spreading in Space Mediate Long-Distance Effects. Signal Transduction into the Cell’s Interior Is Always Needed
Signalling molecules are released, transmitted and received by many different means. Figures 11.1, 11.2, 11.3 and 11.4 illustrate basic mechanisms. In each instance it is necessary that the target cell forwards the message into the cell’s interior.
Independent of the respective biological field of application, intercellular signalling systems follow, as a rule, a chain of events:
1.
The signal is passed on to the neighbours by means of an extracellular molecule or molecule moiety; this molecule becomes the ligand of a receptor at the target cell: in the case of hydrophilic signal molecules the receptor is provided on its surface, in the case of lipophilic molecules it is present either within an interior compartment or also as an integral membrane protein.
2.
In the case of membrane-bound receptors a system is needed that forwards the message away from the membrane into the cytosol of the receiving cell (Figs. 11.5, 11.6, 11.7, 11.8, 11.9, 11.10 and 11.11). In addition, as a rule, these systems amplify the signal strength through downstream enzyme-catalysed processes.
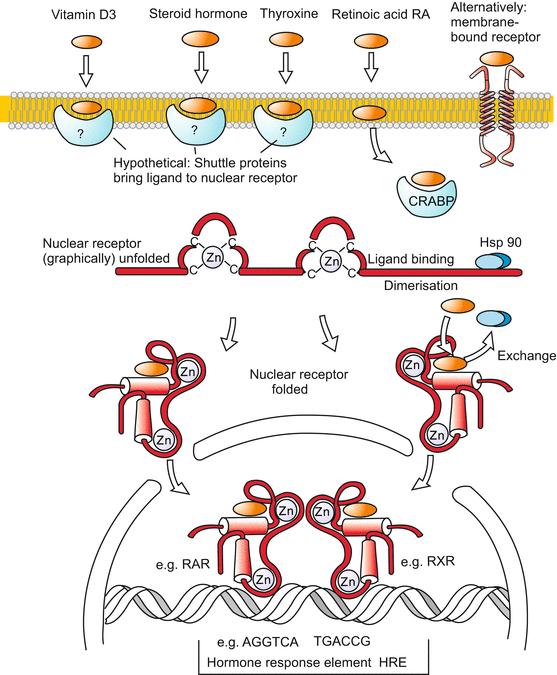
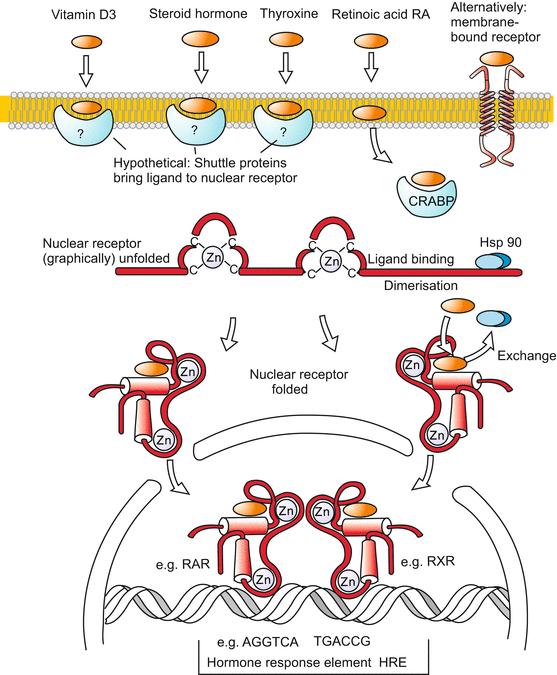
Fig. 11.11
Signal transduction 7: Lipophilic signalling molecules and nuclear receptors. A series of biological signalling molecules, in particular those with (predominantly) lipophilic properties such as steroids, vitamin-A derivatives, thyroxin or corticoids penetrate the cell membrane. In unknown manner (perhaps by means of shuttle proteins with a lipophilic binding domain) they arrive in the cytosol where they bind to intracellular receptors. The signalling molecules displace an inhibitory ligand (Hsp90) thus enabling their receptor to be taken up by the nucleus and binding to control sequences of genes. The receptors for the depicted signalling molecules all belong to the same protein family and possess very similar structures. At the controlling sequences that are marked by HRE sequences, the receptors form dimers, frequently heterodimers whereby the two receptors are loaded with different hormones. In this way a synergistic control of gene activities by two different hormones is possible
3.
Eventually in many cases gene activities are turned on, others turned off, by means of transcription factors. In parallel, other functionally important proteins, for instance ion channels or metabolic enzymes, may be modified: ion channels opened or closed, enzymes activated or inactivated. In the implementation (realization) of such changes often phosphorylation of the proteins by kinases plays an important role.
Before we take a closer look at actual signalling molecules and signalling cascades we will summarize the general nature of signalling molecules and become acquainted with the mechanisms that lead the signals from the emitter to the receptors of the receivers.
11.1.2 A Large Array of Morphogens, Inducers and Other “Factors” Control Development and Growth
A look back to the processes of induction in the amphibian embryo and to pattern formation in the avian wing bud, and a look forward on the development of the nervous and blood system and the regulation of the supplies of blood cells reveals that a multitude of molecules can adopt the function of a messenger.
As biological signalling molecules are effective in minute quantities their identification is difficult, even though their number is growing incessantly. Initially, they reveal their presence only by the effect exerted by a piece of tissue, an extract or the supernatant of a cell culture. Since at the beginning of the search the chemical nature of the effective agent is unknown, the noncommittal designation “factor” gained currency, and when once a substance is labelled “factor” this label remains attached even when the molecule is chemically identified. As a rule, one can assume that a “factor” is a polypeptide (peptide, protein). Therefore it is unable to directly enter the cell but is bound to a receptor on the cell’s surface.
Rich sources of soluble signalling molecules are cells that are propagated in culture and release growth factors into the medium (conditioned media). By means of such factors the cells stimulate themselves mutually to proliferate and therefore the factors were designated growth factors. Other factors induce differentiation or chemotactic movements. The majority of factors identified so far are polypeptides with 500 to 1,000 amino acids. When their sequences were compared it often turned out that one and the same factor was detected with different methods by different research groups and named differently. All factors described so far are multifunctional and used in the organism for very diverse biological purposes.
Examples
The Bone Morphogenetic Proteins BMP stimulate growth of chondroblasts (cartilage precursors) and osteoblasts (bone precursors) and they have functions in bone formation. In addition, members of the BMP family are already found in the early embryo (blastula) well before skeletal elements are being formed. In the early embryo BMPs function as morphogens aiding in realizing the basic body plan of a bilateral symmetric organism (see Figs. 5.18, 5.19, Fig. 10.3, Chap. 10).
Insulin, a well-known hormone regulating blood sugar levels, is found in the embryo long before the Langerhans’ islets are formed in the pancreas, quoted as the place of insulin production in textbooks of physiology.
Multi-functional factors, therefore, often cannot be assigned to a single category but have to be classified among different categories dependent on developmental stage and local function.
11.1.3 Determination Factors, Morphogens, Inducers, Differentiation Factors and Chemokines Are Distinguished According to the Biological Functional Range
One possibility of classification is to categorize development-controlling molecules according to their function in the early embryo.
Determination factors specify the future fate of a cell or group of cells. Morphogens and inducing factors are subsumed within this category.
Morphogens act within a morphogenetic field from which complex structures such as extremities or eyes arise, and they contribute to pattern formation; their locally different effects being a function of their locally different concentration.
Inducers are produced by local emitters and act on neighbouring cells.
Growth factors and cytokines are involved in the control of proliferation (control of cell division).
Differentiation factors initiate terminal differentiation of pre-determined (comitted) cells.
Chemokines are signalling molecules emitted by cells of the target areas, or cells along the way to the target, to guide migrating cells. We refer to the chemokines which guide primordial germ cells (see Fig. 15.2), neural crest cells (Chap. 15, Fig. 15.3), neuroblasts (Chap. 16) or cells of the blood and the immune system (Chap. 17) to their target places.
In practise this subdivision is often not applicable without overlap. Depending on dose and operating place as well as competence of the target cell, but also dependent on tradition and school of thought that had imprinted a researcher, only one of the multiple effects is placed in the foreground or one of many designations is preferred. The “inducers” in the Xenopus embryo are factors belonging to multiple categories all at once.
11.1.4 Autocrine, Paracrine and Endocrine Factors (Hormones) Are Distinguished According to Operating Range and Targets
Another classification accounts for the range and target of the signalling molecules (Fig. 11.1):
1.
Autocrine factors retroact on the producers themselves. The emitter cell possesses receptors for its own signals. These may retroact on the disposition to divide, or cause the emitter to continue or stop signalling. For example, via autocrine stimulation members of the TGF (Transforming Growth Factor) family promote tumour formation.
2.
Paracrine factors diffuse in the interstitial spaces towards neighbouring cells. Here again, the morphogens and inducers in the early embryo could be quoted. In postnatal mammals a protein called PDGF (Platelet-Derived Growth Factor) which plays a role in the regeneration of injured blood vessels and injured tissue is among the paracrine factors.
3.
Endocrine factors = hormones. To get signals to distant targets use is made of circulating fluids in blood vessels (convection, perfusion) Fig. 11.1). Examples are erythropoietin which regulates the amount of red blood cells (Sect. 18.2.7) and the growth-promoting IGFs (Insulin-like Growth Factors, also called somatomedins) in the fetus. When the liver has taken over their production these factors are designated hormones.
Regular classic hormones that are distributed with the blood or other body fluids (lymph) intervene, as a rule, only late in development because the distribution systems must be created first.
Circulation hormones act to initiate and synchronize large-scale processes of transformation, for example control of pregnancy (Chap. 6), sexual development and maturation (Chap. 7), and metamorphosis (Chap. 20); one speaks of endocrine control. Hormones can reach many and distant receivers but cannot provide positional information. A general introduction to the topic of hormones is given in Chap. 20. With regard to its effects on gene activities the paracrine factor retinoic acid, RA, takes a mid-position between “factors” and “hormones”.
11.2 How Cells Communicate: Signal Transmission and Propagation
Well-ordered cell differentiation, directed cell locomotion and the coordinated formation of shape, are true morphogenetic processes in which many cells participate. These processes require communication and interaction between the cells. The transfer of information from cell to cell is called signal transmission. If the message is distributed over large distances one speaks preferentially of signal propagation. The passing on of the message from the cell surface into the interior is designated signal transduction.
In every organism a multitude of signalling molecules is used for a multitude of purposes. Accordingly, there are many means of signal transfer from the emitter to the receiver too, dependent on the physical properties of the signalling molecules and the physical and chemical properties of the tissue which they have to pass and to affect. For a first overview we choose a classification based on the mechanism and the range of signal transmission.
11.2.1 Contact Molecules Mediate Direct Communication With Neighbours
Cells interact with adjacent neighbours by means of signalling molecules anchored in their cell membranes. All cell adhesion molecules CAMs (Fig. 11.2; and Chap. 14) have signalling characteristics. Closely related to CAMs are signal-receptor systems like DELTA-NOTCH (Figs. 11.2b, and 11.7b). Both the receptor NOTCH, and its ligands DELTA and Jagged are anchored in the cell membrane. The system serves to enhance slight differences between neighbours, to drive both cells in varying differentiation pathways, and to demarcate the boundaries between groups of cells so that they can physically separate from each other. In pathfinding of migrating cells further membrane-associated molecules play roles, for example cadherins, ephrins and ephrin receptors, but also components of the extracellular matrix, for example fibronectin which interacts with cell associated proteins such as integrins (Fig. 11.2c). (In addition, soluble molecules present in form of gradients can facilitate orientation).
11.2.2 Stationary Information Carrier and Signposts in the Extracellular Matrix Fulfil Leading Roles
In the clefts and spaces between cells macromolecules such as proteins, glycoproteins and proteoglycans are released which not only constitute an “extra-cellular matrix” ECM and serve as stuffing and stabilising material but also serve as trail signs for migrating cells, outgrowing nerve fibres, and sprouting blood capillaries (Fig. 11.2; Chaps. 16 and 17). Collagen IV, fibronectin, laminin and heparan sulphate proteoglycans are well-known molecules of the ECM which assume a signalling function on demand. Frequently specific signalling molecules released by emitters are bound to the ECM, e.g. netrin and other molecules that label places for growing nerve fibres (Sect. 16.5). They confer specific information, comparable to words on signposts. Some specific factors such as FGFs are bound to the ECM and stored until they are enzymatically released.
11.2.3 Communication Via Liberated, Diffusing Signalling Molecules
The above mentioned DELTA also belongs to those molecules that are partly released from the surface of the cell by means of proteases and thus enabled to reach distant targets via diffusion (Fig. 11.3b). FGF, EGF and SONIC HEDGEHOG are further examples for diffusible extracellular factors. However, free diffusion is often limited and requires certain preconditions:
Small lipophilic molecules can directly pass cell membranes and move from cell to cell if driven by a diffusion gradient. A signalling function is allotted, for example, to derivatives of retinol (vitamin A) as well as to many low molecular weight lipophilic molecules such as arachidonic acid and its many derivatives known as leukotrienes, prostaglandins and hydroxy fatty acids, together with arachidonic acid subsumed under the collective term eicosanoids. Arachidonic acid derivatives influence, among other physiological events, cell motility. The significance of low molecular weight substances for embryonic development is currently underexplored because it is difficult to identify them, to quantify them in situ, and to trace their spreading.
Larger, +/− apolar (=lipophilic) molecules like thyroxin and steroid hormones are traditionally said to penetrate cell membranes and to be bound to cytosolic receptors (see Fig. 11.11). However, in many cases, shuttle proteins for lipophilic molecules are required: for many functions, especially for gene activation, it is not sufficient that such molecules enter the lipid bilayer of the cell membrane, the signal must be forwarded into the watery cytosol. How this might be accomplished is discussed below in Sect. 11.4.1. On the other hand, for many well-known lipophilic molecules receptors in the cell membranes also have been identified which mediate (fast) responses by starting signal transduction pathways.
Large hydrophilic molecules, in particular polypeptides are able to reach surprisingly distant target cells. The majority of the known signalling molecules are peptides/proteins, for example those listed below, the WNTs, FGFs and the members of the TGF-β family including the BMPs. In the traditional concept that underlies most computer simulations of biological pattern formation (Chap. 19, Box 10.2) propagation happens by diffusion.
Precondition for diffusion-driven propagation of such large molecules is the existence of a fluid filled interstitium that offers the necessary free space. If this precondition is met, the range of spread can be between a few nanometres and millimetres. Some protein factors can also serve as chemotactic attractants (chemokines), for instance the Nerve Growth Factor (NGF) which guides the sprouting of the fibres of sympathetic neurons.
However, some proteins have unexpected properties making it unlikely that their propagation is based on simple diffusion. Thus, WNT molecules are post-translationally linked with palmitic acid, therefore being lipophilic and in watery environment not readily soluble. Possibilities to propagate such molecules are pointed out below in Sect. 11.2.4. Recent work shows that also the extracellular matrix plays an important role by binding or repelling signalling molecules thus restricting or increasing the range of diffusion. For example the ECM binds FGFs and thus restricts their range of diffusion in the developing brain (from 40 cell width to only 5). By degrading ECM components proteases release the factors in due time and at due places.
Channeled Restricted and Facilitated Diffusion.
Free spaces for unrestricted diffusion are rare or lacking in tissues where cells are positioned side by side. Cells in epithelia adhere to each other by means of dense adhesion belts. Therefore, free diffusion is only possible in the baso-lateral clefts but not across the epithelia.
Channeled Diffusion Via Gap Junctions.
Injection of fluorescing, low molecular weight dyes or of isotopes in single cells revealed possibilities for diffusion along certain epithelia. Electrolyte-ions (Na+, K+, Ca2+, Cl−) and small polar (=hydrophilic) molecules, such as inositoltrisphosphate (IP3), cAMP, cGMP and ATP, can diffuse from cell to cell when the cell membrane of adjacent cells are equipped with conducting gap junctions (tube-shaped transmembrane channels, Fig. 11.3a).
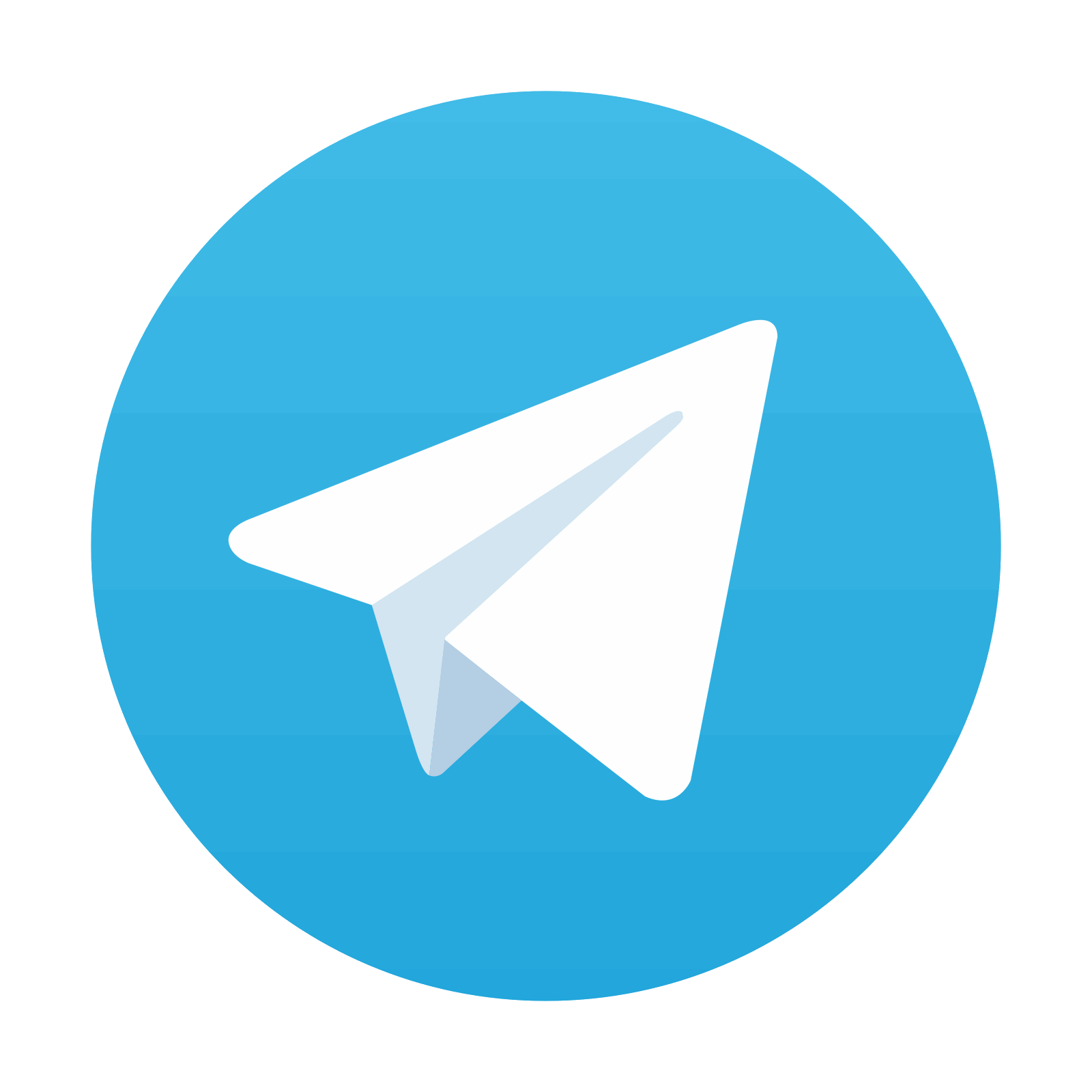
Stay updated, free articles. Join our Telegram channel

Full access? Get Clinical Tree
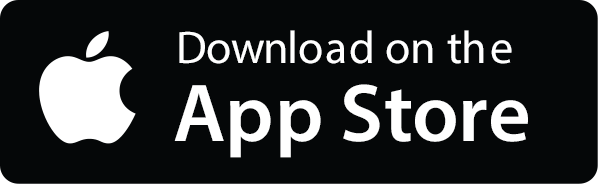
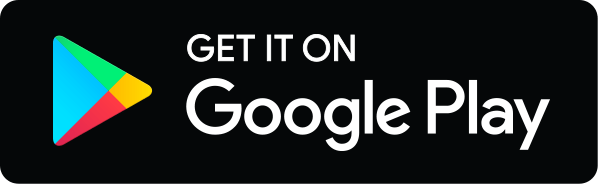
