© Springer Science+Business Media New York 2014
William V. Holt, Janine L. Brown and Pierre Comizzoli (eds.)Reproductive Sciences in Animal ConservationAdvances in Experimental Medicine and Biology75310.1007/978-1-4939-0820-2_2020. Conclusions: Environmental Change, Wildlife Conservation and Reproduction
(1)
Academic Department of Reproductive and Developmental Medicine, University of Sheffield, Jessop Wing, Tree Root Walk, Sheffield, S10 2SF, UK
(2)
Center for Species Survival, Smithsonian Conservation Biology Institute, 1500 Remount Road, Front Royal, VA, USA
(3)
Smithsonian Conservation Biology Institute, National Zoological Park, Washington, DC, USA
Abstract
Our intention when planning this book was to explore the diverse ways that reproductive science is inextricably tied to many aspects of biodiversity conservation, using the opportunity to present a vast amount of specialised information in a way that forms a coherent and important body of work. Some of the chapters were therefore concerned with understanding how taxonomic groups and species are being affected by globally important environmental changes, mostly caused through anthropogenic influences. Others were more focused on monitoring and understanding the physiology of wild species, with the aim of better understanding mechanisms underlying responses to captive conditions and environmental change, in both wild and captive animals. We also wanted to review advances in technological measures that are being actively developed to support the breeding and management of wildlife. In a few cases we have presented specific case studies that highlight the amount of effort required for the successful development of assisted reproductive technologies for wild species. Viewed overall, the outcome is spectacular; the last decade has seen enormous progress in many aspects of the sciences and technologies relevant to the topic. It is also clear that the boundaries between different scientific disciplines are becoming ever more blurred, and it is no longer easy or even possible to remain focused on a highly specialized topic in reproduction or conservation, without having at least some understanding of allied subjects. Here we present a few concluding comments about what we have learnt, and how the various topics interact with each other. We also emphasize that, as far as we know, no similarly comprehensive consideration of the contribution of reproductive science to wildlife conservation has been published within the last decade.
Keywords
Assisted colonizationClimate changeCryobiologyEpigeneticsExtinctionGenomics1 Is Reproductive Science Helpful for Conservation?
The global environment is under increasing pressure from expanding human activities and, despite the efforts of climate change deniers and sceptics, the outcomes are widely accepted by the scientific community (Rosenau 2012). Species of all types, especially those that cannot migrate, have to face increasing environmental temperatures, atmospheric and marine pollution, ocean acidification, desertification and other threats. Ecosystems are therefore changing in response to these problems, and despite a few notable achievements in terms of protection of rare species and habitats, traditional conservation approaches have been largely powerless to reverse or stabilize the underlying rhythms of the global economy. Documenting these problems has largely been the preserve of ecologists, whose work often identifies long-term biological trends associated with environmental change, and whose advice is then fed into the formulation of policies for habitat protection (Bino et al. 2013). However, the formulation of those policies tends to be in the hands of politicians who, under pressure from interested lobby groups, are free to ignore the well intentioned advice while species go extinct. The precarious state of the endangered Leadbeater’s possum in Victoria, Australia, is a topical example of a mammal likely to undergo extinction because of timber logging (see http://www.depi.vic.gov.au/environment-and-wildlife/wildlife/leadbeaters-possum).
One might therefore ask whether reproductive scientists can really play a useful part in solving these problems. The causes of imminent extinctions of wild species are not, after all, always caused by simple failure to breed. This was, however, the problem with the Florida panther, an endangered subspecies of puma (Felis concolor coryi), where some years ago the population was known to be shrinking as individual animals showed one or more of a variety of physiological, reproductive, endocrine, and immune system defects (Facemire et al. 1995). Initial hypotheses for the decline of this species blamed the presence of environmental endocrine disrupters, but eventually it was determined that the reproductive abnormalities were caused largely by inbreeding depression. The introduction of new genetics into the population, by the importation of unrelated males of another subspecies (F. c. stanleyana), successfully reversed the situation and resulted in a new generation of healthy animals (Mansfield and Land 2002; Hostetler et al. 2012). Without input from reproductive biologists, the problems associated with abnormal sexual differentiation and defective sperm function would not have been recognised. The recognition that abnormal sexual differentiation (i.e., feminization) in fish within UK rivers was caused by the presence of endocrine disrupting chemicals (EDCs) originating from sewage was also a landmark contribution to conservation made by reproductive biologists (Jobling et al. 2002; van Aerle et al. 2001; Rodgers-Gray et al. 2001), and, as explored in this book, the negative consequences of endocrine disruption exposure by chemicals in the environment are widely recognised in many different taxonomic groups and monitored all over the world.
It is also important to note that humans are not exempt from the deleterious consequences of EDC exposure, and there is a widespread consensus that sperm output in men has been declining globally over the last several decades. This was first noted, and widely dismissed, in a controversial paper by Carlsen et al. (1992), but now has been confirmed by reanalysis and updated with new sperm concentration data from many countries (Swan et al. 2000). Detailed studies on laboratory species have revealed potential mechanisms for the decline in sperm production, the most likely of which is exposure to industrial chemicals at important stages of sexual differentiation. These findings have certainly had high level impacts and have stimulated global regulatory initiatives aimed at safety assessments for newly manufactured chemicals, and the enforcement of safe disposal methods. This particular example also emphasises the important interdisciplinary benefits that emerge when related fields in wildlife science and human clinical medicine collide or collaborate. It is increasingly recognised that mechanisms exist whereby exposure to an EDC such as Bisphenyl A in one generation produces reproductive and other effects that persist down the generations (Sofiane et al. 2013).
Similarly, the reported rapid changes to the breeding seasons of wild species, coupled with loss of their normal synchronization with the seasonal production of their appropriate foodstuffs, parallels the linkages between inadequate nutrition and early embryonic development in mammals. It is now well established that the occurrence of many adult diseases such as diabetes and hypertension, can be traced back to mismatches between the poor availability of nutrition at the embryonic stage and richer diets later in life (Barker et al. 2010; Barker 1999). Such problems have been replicated in laboratory species, and it seems logical to expect that the relatively rapid environmental changes observed today would induce similar effects in wild species.
These relationships have received very little attention in terms of wildlife, but we suspect they will soon be recognised as being highly influential modulators of species survival. An improved understanding of these interactions is, however, not easy to achieve. It requires a great deal of scientific effort and is problematic in unusual species, especially those whose genomes have not yet been sequenced, microarrays for gene expression studies have not yet been developed, and epigenetic chromatin patterns are not known. Nevertheless, over the last decade, biologically-based technologies of all descriptions have leapt forward in ways that were previously impossible to foresee, most notably in the field of genomics. Whole genomes can now be sequenced relatively cheaply, specific DNA and RNA sequences can be easily detected on a massive scale using array technologies and when these are combined with proteomic technologies and computational power, the ability to generate data in basic cell and molecular biology is staggering. Projects such as “Genome 10k” (http://genome10k.soe.ucsc.edu/ ), which aims to evaluate the DNA sequences for 10,000 vertebrate species, will contribute towards these advances and their progress will hopefully accelerate as time passes.
Knowing the DNA sequence is, of course, only a first step in understanding the ways that cells and whole animals work. How species with similar DNA sequences, such as humans, chimpanzees and bonobos that share 98 % of their alignable genomic sequence, develop into phenotypically distinct animals is still poorly understood. A recent study into this question (Marchetto et al. 2013) showed that the expression and “within-genome” mobility of a retrotransposon, known as “long-interspersed element-1”, may have exerted a profound influence in primate evolution. It is of interest in the context of this book that this study, whose aim was mainly to study processes in evolution, was only possible because the authors were able to generate pluripotent iPS cells from an archive of frozen chimpanzee and bonobo fibroblasts. Within this book we have stressed the importance of cryopreserving cells and tissues from wild species, partly for their potential use in assisted reproductive technologies, but also because of their value for scholarly and applied research. This represents just one example of an important insight in basic biology that would not have been possible without access to frozen cells and highlights the unforeseeable value of maintaining cryopreserved cell and tissue collections from wild species. While the immediate benefit of establishing such collections has not always been clear, especially to funding bodies with focused conservation or biomedical goals in mind, the consistent pace of advances in genomics emphasises the benefits of being able to study the entire cell, with its elaborate compartmentation, complex system of regulatory RNA molecules and its interplay with mitochondrial DNA, rather than only having a genomic DNA sequence available for examination.
Over the past 60 years the practice of cell and tissue preservation has relied heavily on freezing technology, with the result that repositories of cells and tissues around the world are reliant on electrical or liquid nitrogen-cooled freezers of various sorts. These are not only expensive to purchase and maintain, but their use is completely dependent on reliable availability of power or regular supplies of liquid nitrogen. Even short-term sample storage in remote regions of the world is therefore difficult, while even well-established repositories are vulnerable to power failures and equipment breakdown. In an effort to bypass these problems a number of research groups are investigating the feasibility of drying the samples and then keeping them in sealed containers at room temperature. Loi et al. (2008) showed that if freeze-dried nuclei were injected into enucleated sheep oocytes they would undergo the early stages of embryonic development, and several groups have shown that freeze-dried mouse spermatozoa can generate embryos after intracytoplasmic sperm injection (ICSI) of oocytes (Li et al. 2007a, b; Kusakabe et al. 2008). More recently Graves-Herring et al. (2013) have shown that isolated germinal vesicles can be dried and stored without losing the ability to resume meiosis after rehydration. Developments in this field, recently reviewed by Loi et al. (2013) would revolutionize cell preservation in its many forms. Human infertility clinics, animal breeding facilities, museums, and cell repositories of all types could dispense with their collections of freezers and liquid nitrogen containers in favour of sealed tubes stored at room temperature. No doubt this is an optimistic prediction when considered in the ever-present light of species differences, but the potential benefits of this approach are so important that they cannot be ignored. It is interesting to see that the pioneers in this field are largely drawn from the community of conservation-focused reproductive scientists.
Scientific insights and new technologies develop a synergy of their own, and in this book we have highlighted several examples directly relevant to conservation biology. After a good deal of concentrated effort, Janine L. Brown and her colleagues eventually managed to develop a successful method for artificial insemination in elephants (Brown et al. 2004; Hermes et al. 2007); however, the practical implementation of this method was preceded by focused research into the ovarian dynamics of the elephant, and in turn this has led to further basic research into relationships between body condition and reproduction. These investigations are fundamentally important in elephant management, but it is of interest that they are also important in understanding the likely implications of habitat modification and dietary changes. The elephant research is also relevant to human medicine, where the negative consequences of human obesity on the chances of conception are matters of considerable interest to infertility clinics.
The last decade has also seen advances in technologies that can be used to the benefit of dwindling and threatened wild populations, and it is clear that there has been a great deal of international effort invested in gaining more understanding of wild species and developing technologies to help prevent their extinction. Motivations vary; some major projects have underlying economic aims, such as the “Millennium Seed Bank” established by Kew in the UK (Madeley 1999), which aims to conserve seeds from most of the world’s plants and the National Animal Genetic resource program in the USA (Blackburn 2004, 2012). Other projects are often founded for nationalistic or cultural reasons; conservation of the giant panda (Ailuropoda melanoleuca) in China, the Mauritius pink pigeon (Nesoenas mayeri), Asian elephants in Thailand and nearby countries, the Global Tiger Initiative, and seahorse preservation in many different locations, exemplify projects taking place around the world. Many of these projects, whether established for economic, cultural or other reasons, can benefit from reproductive technologies and knowledge of species biology gained over recent years. Technologies such as artificial insemination and semen freezing still have a long way to go, mainly because success is species-dependent in many respects, even with closely related species within a taxon (e.g., Felidae). Long-term and well-focused studies are beginning to pay off where they are supported by detailed background research. Extensive work on amphibian-related technologies over the last decade is one example of a field that has progressed remarkably since the first reports of sperm motility recovery in cane toads, used in that instance as a model species (Browne et al. 1998) to the industrial scale production and reintroduction of Wyoming and Boreal toads in Colorado, reported in this book by Clulow and colleagues. The latter is an excellent demonstration that a laboratory based technique, mainly used for solving fertility problems in individuals, can be transformed into a tool for the conservation of whole populations. The preservation of coral cells and gametes is another example of eventual success being achieved through long-term efforts. Rather like the amphibian work, the aims of coral cryopreservation are directly focused on the preservation of coral reefs, but are focused on a population (the reef as an assemblage) rather than individuals. If corals can be maintained ex situ, with cryopreservation as a genetically supportive tool, it may be possible to repopulate extant coral reefs if and when the environment recovers. Incidentally, prospects for coral recovery are being assisted by studies of their related microflora (Hunt et al. 2012; Kvennefors et al. 2012) as there is some hope for coral restoration through rebalancing abnormal bacterial populations with methods such as antibiotic treatment (Sheridan et al. 2013). This demonstrates the complexity and unexpectedness of conservation measures in different fields.
The potential importance to reproductive success of maintaining well-adjusted microflora in corals parallels the situation in mammals where reproductive tract function is disturbed by infections. Studies in female dogs (bitches) have revealed that uterine infections interfere with sperm transport by reducing the sperm-binding ability of the uterine epithelia (England et al. 2012). More generally, reproductive tract infections are known to interfere with implantation and, much like peri-conception pollutant exposure, exert negative impacts on embryonic development with long-term epigenetic effects lasting into adulthood (Kwak-Kim et al. 2010; Silva et al. 2012; Yang et al. 2011). Recent genomic studies in humans have shown that the reproductive tract, especially the vagina, contains a highly complex but relatively constant microflora, but that shifts in the balance between bacterial species and communities can result in reproductive problems. After conducting a review of the literature, Reid et al. (2013) proposed that supplementing the altered microbiome with probiotic lactobacilli, which are antagonistic to pathogens, would improve reproductive health and reduce the number of pregnancy-associated complications. Speculatively, these insights probably should have practical relevance for assisted breeding technologies applied to non-domestic species, especially if they become stressed through handling and captivity. They may, however, explain some instances of poor reproductive success in the face of environmental stress.
Technologies for monitoring wild populations through faecal, urinary and blood analyses have remained rather laborious and need improvements. This is not entirely a technical problem as assays already exist for laboratory species and humans. The difficulty relates more to the scale of investment needed to develop and validate suitable antibodies, when the potential market is small and uneconomical. Perhaps advances in synthetic biology will enable the development of assays that are effective across multiple species. In fact, as noted by several authors in this book, hormone assay techniques are crucially important in understanding reproductive dynamics in many species. However, apart from the gradual transition from radioimmunoassay techniques to enzyme-linked immunoassays, there has not been a revolution in assay technology that matches some of the genomic techniques available today. It is now feasible to take a small, inexpensive and integrated PCR machine into the field to monitor multiple DNA sequences in river or pond water, thus obtaining an instant snapshot of the range of species present. By comparison, collecting multiple faecal samples in the field, drying and transporting them to a laboratory for analysis is a lengthy and labour-intensive process that in the wrong hands often fails for no apparent reason. Technological advances in this area would be very welcome. Future developments in this field will involve biosensors that can be implanted for long periods in free ranging species so that data can be collected remotely and uploaded via satellite links. Data such as body temperature, blood pressure, pH, blood glucose, etc. should be relatively easy to develop as the technologies already exist; biosensors capable of steroid hormone measurement already exist to some extent (Posthuma-Trumpie et al. 2009); for example, progesterone biosensors in milking lines can measure the hormone within 5 min (Friggens et al. 2008; Kappel et al. 2007), but have yet to be developed for long term remote use. Biosensor technologies would be especially useful for studies with wild marine species whose ranges enclose thousands of square miles; in fact, simpler devices that detect and report on swimming depth and location are already available and are being used with species such as turtles, sharks (Hays et al. 2001; Wang et al. 2012; Wearmouth et al. 2013) and eels (Breukelaar et al. 2009). Such an ability to remotely monitor reproductive or other health biomarkers would revolutionize our ability to assess physiological function in both captive and free-living species.
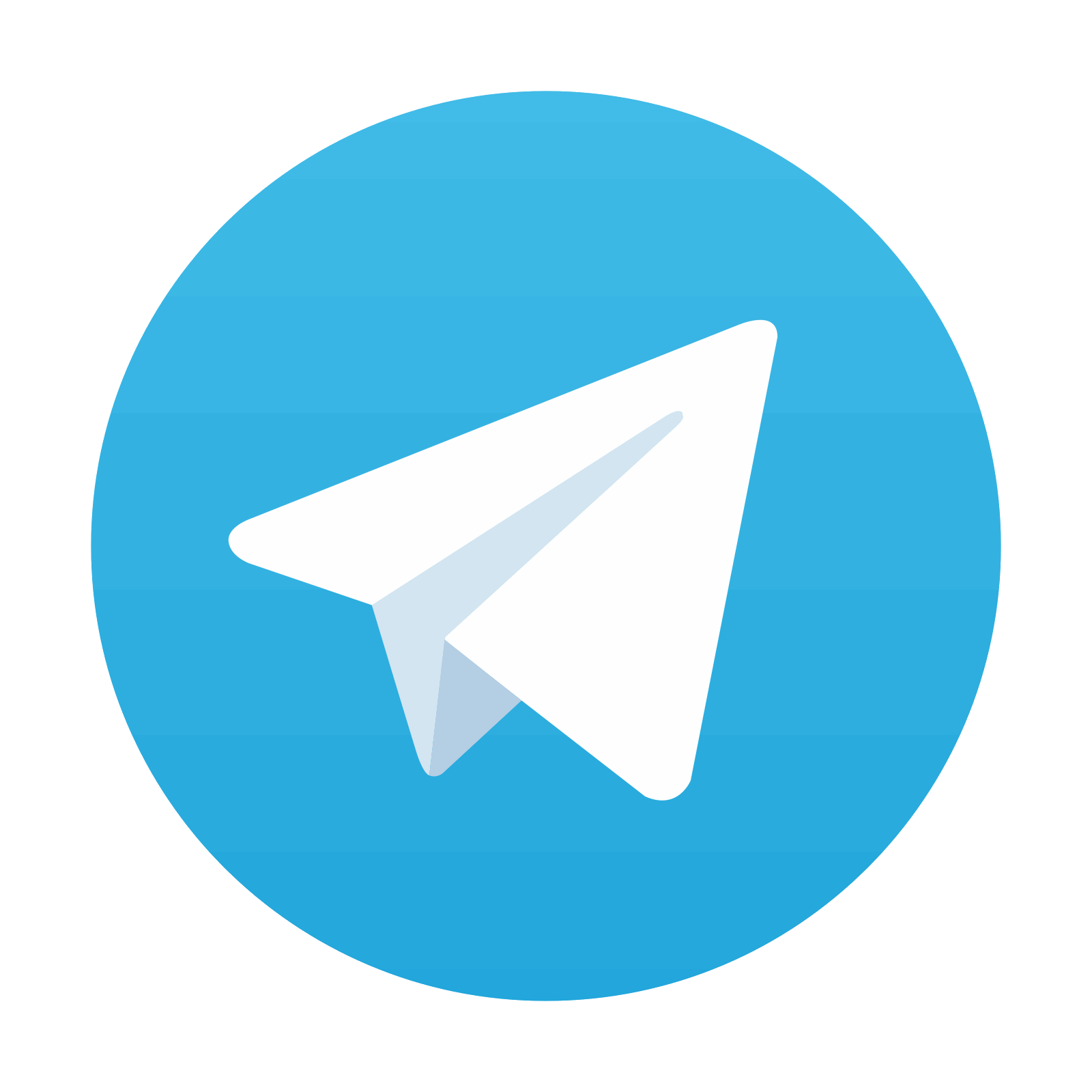
Stay updated, free articles. Join our Telegram channel

Full access? Get Clinical Tree
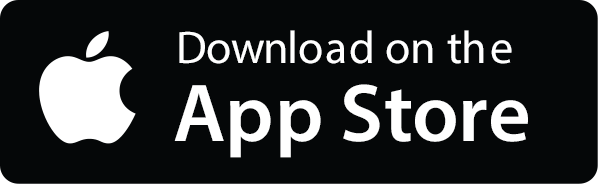
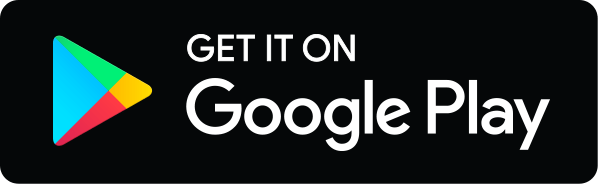