Fig. 1.1
Gross ocular anatomy. Human and nonhuman primate eyes are similar, with the NHP eye at approximately half the scale of the human eye. Other species presented for comparison demonstrate the more obvious differences such as lens size, vitreous and aqueous chamber size, and corneal thickness
1.2 Eyelids
The eyelids are often ignored in ocular studies being considered “in the way” of the true region of interest, the eye itself. However, the eyelids do play a key role in ocular maintenance in the form of the blink. The true mechanism of blink control is not fully understood. It is likely a combination of a central nervous system mediated “blink center” that receives sensory input from the ocular surface as well as reflex from visual and mental sources. In humans, blinking can occur automatically (but not at truly fixed intervals), as a reflex to a visual or sound stimulus and at will. Most mammals can do the same. Blinking cleans the surface of the eye, results in the application of the lubricating tear film to the ocular surface, and prevents complete photobleaching of the photoreceptors by providing brief instances of darkness. In humans, the duration of a blink is approximately 250–400 ms [43], which translates into an additional 5–7 min of “darkness” each day during normal waking hours for humans. Although blinking speed has not been well studied in laboratory animals, it has been observed in dogs and nonhuman primates to be in the range of approximately 100–300 ms [11], which is not dissimilar to humans. In addition to applying the tear film, the eyelids are also involved in draining excess tear film, or topical drops for that matter, from the ocular surface. All mammals have two functional eyelids; however, they are not all created equally. Of interest to the toxicologist is the drainage system since it will impact on the duration of the presence of a liquid eye drop on the surface of the eye and by inference the amount of systemic exposure that might occur. Normal tear film is drained from the eye by small openings in the eyelids called puncta. The puncta are connected to nasolacrimal ducts which drain into the sinus onto the nasal mucosa. Their location on the eyelid can vary somewhat, but in general they are located in the medial canthal area, near the edge of the eyelid where the conjunctiva and skin meet. Most laboratory species as well as humans have one punctum on each upper and lower eyelid. Minipigs (pigs in general) only have puncta on the upper eyelid and rabbits only on the lower eyelid. In humans, approximately 75–80% of tear volume is drained with each blink. It has been estimated that up to 80% of a topical drop can be absorbed systemically [32, 51], with some absorption through the conjunctival vasculature and most of the fluid arriving directly on the highly absorbent nasal mucosa via the nasolacrimal duct system. Mechanically, the drainage occurs by the suction force created when the upper and lower eyelids part, when fluid is drawn into the nasolacrimal drainage system. The size and shape of the eyelids also plays a role in systemic absorption. A species with looser eyelids and larger conjunctival sac such as a rabbit may have more “drop” available for absorption on a subsequent blink than a nonhuman primate which has eyelids more tightly pressed to the eye, where runoff onto the surrounding skin and/or fur from the initial blink is likely to be higher (refer to Fig. 1.2). Similar systemic absorption after topical ocular instillation has been observed in dogs and rabbits [11]. The concern with the systemic absorption is the potential for unwanted systemic side effects. In adult humans, the unintended systemic dosage is usually relatively low on a body weight basis, and consequently, the risk of side effects in the general population is also low, with exceptions for certain susceptible populations. However, when the dose/body weight ratio becomes higher such as in children, the side effects can be more serious [23]. Scale that effect to a 2-kg rabbit or nonhuman primate and the potential for systemic toxicity increases further.
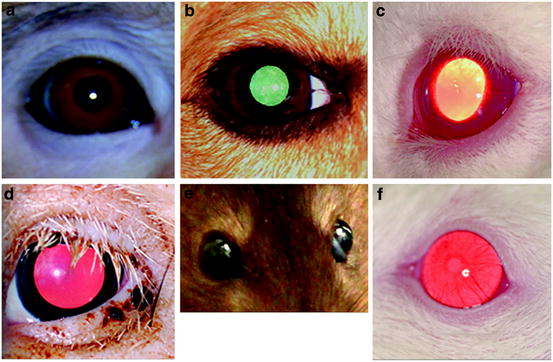
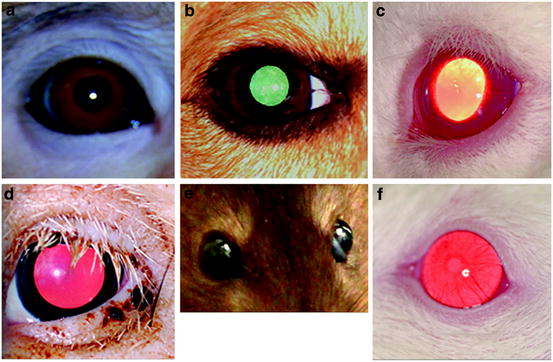
Fig. 1.2
Relative eyelid configuration and eyeball exposure. (a) Cynomolgus monkey, (b) dog, (c) New Zealand White rabbit, (d) Göttingen minipig, (e) Brown Norway rat, (f) albino mouse
Because blinking can influence the residence time of a topically applied test substance in the eye as well as the tear film, the blink rate may provide some insight into interspecies local or systemic reaction to treatment. A faster blink rate can result in decreased residence time on the eye as well as alter the systemic absorption characteristics of a topically applied product compared to a slower blink rate. Blink rates for the various species are presented in Table 1.1.
The nictitating membrane, or 3rd eyelid, is a translucent to opaque structure that supplies additional lubrication and cleaning of the corneal surface. It is not easily visible in most laboratory species, and though it has some sympathetic innervation with minor musculature in some species (like cats), its motion is mostly passive occurring with slight retraction of the eyeball and/or the action of blinking. It is also thought to be a protective structure in the animals’ natural environment. The presence of this structure in laboratory species needs to be considered when conducting certain ocular evaluations such as scoring of local irritation or when comparing tear film or corneal changes between species. For example, a protruding or inflamed nictitating membrane may be confused for severe conjunctival hyperemia or may physically mask other ocular changes, and the absence or presence of the third eyelid could be the difference in why one species exhibits symptoms of ocular dryness or erosion and another does not after receiving the same topical drug (Table 1.2).
Table 1.2
Species with nictitating membranes
Human | Nonhuman primate | Minipig | Dog | Cat | Rabbit | Rat | Mouse |
---|---|---|---|---|---|---|---|
No | No | Yes | Yes | Yes | Yes | Yesa | Yesa |
1.3 Conjunctiva
The conjunctivae are the transparent membranes that line the underside of the eyelids and cover the sclera. There are three primary classifications: (1) the palpebral, covering the underside of the eyelids, is thick and can be reddish in appearance; (2) the bulbar, covering the sclera, is thinner, vascularized, and transparent but may contain some pigment in more heavily pigmented animals, such as nonhuman primates; and (3) the fornix, forming the junction where the palpebral turns to meet the bulbar. The conjunctival sac or cul-de-sac is the space formed by this arrangement.
The conjunctivae serve several purposes. They provide lubrification, help to hold the eye in place, and allow it to move smoothly within the eye socket.
The amount of conjunctiva visible when observing an eye varies by species (as seen in Fig. 1.2), with a larger amount visible in rabbits compared to nonhuman primates. The differences in the amount of visible conjunctiva can make it more or less difficult to evaluate surface irritation (conjunctivitis) after administration of a test substance.
1.4 Pre-corneal Tear Film and Ocular Glands
The pre-corneal tear film is composed of aqueous and lipid layers and is secreted by several glands mostly located around the eye. The tear film is spread over the cornea during blinking, and its composition is related to the blink rate of the various species. A more aqueous tear film is subject to more evaporation and requires more frequent reapplication than a more lipid-based tear film. The glands involved in the secretion of the tear film include the lacrimal gland (located in the orbit), the Harderian gland (located on or near the nictitating membrane and therefore not found in the primates), accessory lacrimal glands, Meibomian glands (located on the eyelid margin), and goblet cells (located in the conjunctiva (palpebral and fornix). In general, the tear film has three layers. A mucin layer secreted by the goblet cells is the innermost layer. The middle layer is more aqueous and is secreted by the lacrimal glands with contributions from the Harderian gland in some species such as dogs and cats (where the Harderian gland is more similar to a lacrimal gland). The outermost layer is a lipid layer secreted by Meibomian glands and Harderian glands. In rodents, the Harderian glands also secrete porphyrins, which when over-secreted can cause a reddish deposit around the eye [16]. The reason for the presence of porphyrins in the Harderian gland of rodents is unknown, but it suggests a sensitivity to light and a possible relationship to the pineal gland [8].
1.5 Cornea
The cornea is a transparent multilayered structure at the front of the eye that is responsible for allowing light to enter the eye as well as for approximately 2/3 of light refraction (focusing). It joins the sclera in a zone called the limbus. The cornea is avascular, but it has the highest concentration of nerve endings in the body.
The layers of the cornea from outer to inner generally consist of:
Epithelium: The corneal epithelium is several cell layers thick and is continuous with the bulbar conjunctiva. It desquamates at the surface and rapidly regenerates. The epithelium is the primary barrier within the cornea to drugs and bacteria. It is damaged easily and, therefore, alterations to the corneal epithelium may alter drug penetration into the anterior section of the eye. It does not present a uniform surface, and the mucin layer of the pre-corneal tear film fills in the gaps to provide the required optical quality.
Bowman’s membrane: This layer underlies the epithelial layer and acts as a barrier protecting the stroma. Not all species have this structure, including rabbits, dogs, cats, and rodents [30, 56].
Stroma: This is the thickest layer. It is composed of parallel collagen fibrils and is responsible for the refractive power of the cornea.
Descemet’s membrane: This layer underlies and supports the stroma. It is collagenous and elastic and acts as the basement membrane of the corneal endothelium.
Endothelium: The corneal endothelium is a single layer of cells that maintains the proper relative water content of the stroma. Additionally, it transports nutrients to the stromal cells from the aqueous humor and removes waste. It is effectively non-regenerative. In the event of damage to this layer, some cells will enlarge to fill in gaps left by dead cells. A healthy endothelial layer is critical for corneal function. An unhealthy endothelial layer will eventually result in corneal edema (thickening due to increased water content) which will interfere with vision.
The cornea, being avascular, obtains its nourishment from sources such as the tear film, aqueous humor, and a ring of perilimbal vessels located approximately 1–3 mm from the edge of the corneoscleral junction (limbus).
Corneal thickness varies by regions within the cornea, with the time of day, with age, with external influences (e.g., contact lenses), damage, disease, and species. Because of this variability, average central corneal thickness is usually the parameter that is measured and quoted for comparison purposes. Some measurements for laboratory species are presented in Table 1.3.
1.6 Sclera
The sclera is the protective white fibrous sheath around the eye. It is continuous with the cornea and is composed of the same type of collagen fibrils as the stroma. However, as opposed to being aligned in a parallel fashion, the fibrils are in a cross-matrix pattern, which results in the white reflective appearance. Other scleral components include proteoglycans and mucopolysaccharides.
Scleral thickness varies over the ocular surface as well as among species. However, direct comparison of quantitative data is difficult due to the inconsistent methods used to determine thickness. Methods used include measuring thickness on excised fresh tissue with calipers, measuring fixed tissue with calipers or microscopically, as well as in vivo with various imaging techniques. In many species, including NHPs, dogs, and cats, the sclera is thickest at the limbus and thinnest at the equator and somewhere in between near the optic nerve. In humans, it is thickest near the optic nerve, thinnest at the equator, and thicker again near the limbus. In pigs, however, the thickest region is approximately 5–6 mm from the limbus [45], being otherwise comparable to human. In rabbits, measurements are about half as thick as human over most of the sclera surface, thickening only at the limbus [44, 48]. Rodents tend to have thinner scleras than the larger-eyed species.
Much has been said about the relative sclera thickness of various species compared to human and its relationship to penetration of externally applied drugs into the eye. However, though the sclera does play a role in this respect, hydrophilic molecules pass through the sclera fairly easily. An additional significant barrier to ocular penetration appears to be Bruch’s membrane and the vascular choroidal layer which can easily carry away a drug in the circulation.
1.7 Aqueous Humor (Part I)
The aqueous humor is a clear, watery fluid that contains ions, proteins, and other nutrients. It provides nutrients to avascular structures such as the cornea, lens, and trabecular meshwork and removes waste products. It plays a significant role in ocular pressure and maintaining the shape of the globe and therefore the optical quality of the eye.
Aqueous humor is in a constant state of relatively rapid flow and is subject to diurnal fluctuations. The flow rate is an important consideration when evaluating the relative kinetics of a drug in the anterior portion of the eye. A higher flow rate may contribute to increased clearance. Complete turnover can take as little as an hour. Average aqueous humor flow rates are presented in Table 1.4 and estimated aqueous volumes are presented in Table 1.5.
Table 1.4
Average aqueous humor flow (μL/min)
Mouse | Rat | Rabbit | Dog | Cat | Pig | NHP | Human |
---|---|---|---|---|---|---|---|
0.18 [1] | 0.35 [41] | 2.7 [19] | 4.5 [62] | a | 1.95 [47] | 2.8 [46] |
1.8 Iris, Ciliary Body, Trabecular Meshwork, and Aqueous Humor (Part II)
The iris is the muscular diaphragm that controls the amount of light entering the eye by enlarging or narrowing the pupil. It is circular in shape except for cats where it is in the form of a vertical slit-shaped oval. The iris separates two chambers in the anterior segment of the eye. The anterior chamber represents the space between the cornea and the iris and the posterior chamber the space between the lens and the iris. The iris joins the cornea at the irido-corneal angle.
The ciliary body lies in the posterior chamber and is responsible for production of aqueous humor, lens accommodation, and uveoscleral outflow. It consists primarily of ciliary muscle but has extended villus-like components called ciliary processes that are responsible for the production of the aqueous humor. The ciliary processes are also connected to the lens via proteinaceous filaments called zonules. The zonules hold the lens in place and allow the ciliary muscle to exert force on the lens for accommodation.
The plasma-derived aqueous humor is secreted from the epithelial cells of the ciliary processes into the posterior chamber. It flows through the pupil into the anterior chamber where most of it flows out of the eye at the irido-corneal angle via the trabecular meshwork.
The trabecular meshwork, located in the irido-corneal angle in the anterior chamber, consists of a net of cross-linked collagen fibers with some endothelial-like cells. It filters the aqueous humor into Schlemm’s canal (not specifically present in all species), scleral collector channels, the episcleral veins, and finally into the general venous circulation. This constitutes the conventional outflow pathway and accounts for most of the aqueous humor drainage. Damage to the trabecular meshwork or narrowing of the irido-corneal angle can result in reduced outflow and subsequent increased intraocular pressure.
The unconventional pathway, also known as uveoscleral drainage, consists of drainage of aqueous humor through the supraciliary spaces in the ciliary body through to the sclera and choroid. It is difficult to measure, and therefore, there are a wide range of values associated with the amount of aqueous it actually drains. Some estimates place it as a major contributor. For example, in humans, estimated uveoscleral drainage can account for as little as 4% and as much as 60% of total outflow [64]. In rabbits, 3–8% has been reported [6] and in nonhuman primates, up to 60% has been reported [5]. Currently, it is considered to be a secondary outflow pathway in laboratory species.
1.9 Lens
The lens provides the final fine tuning for focus of incoming light and is comprised of three major components:
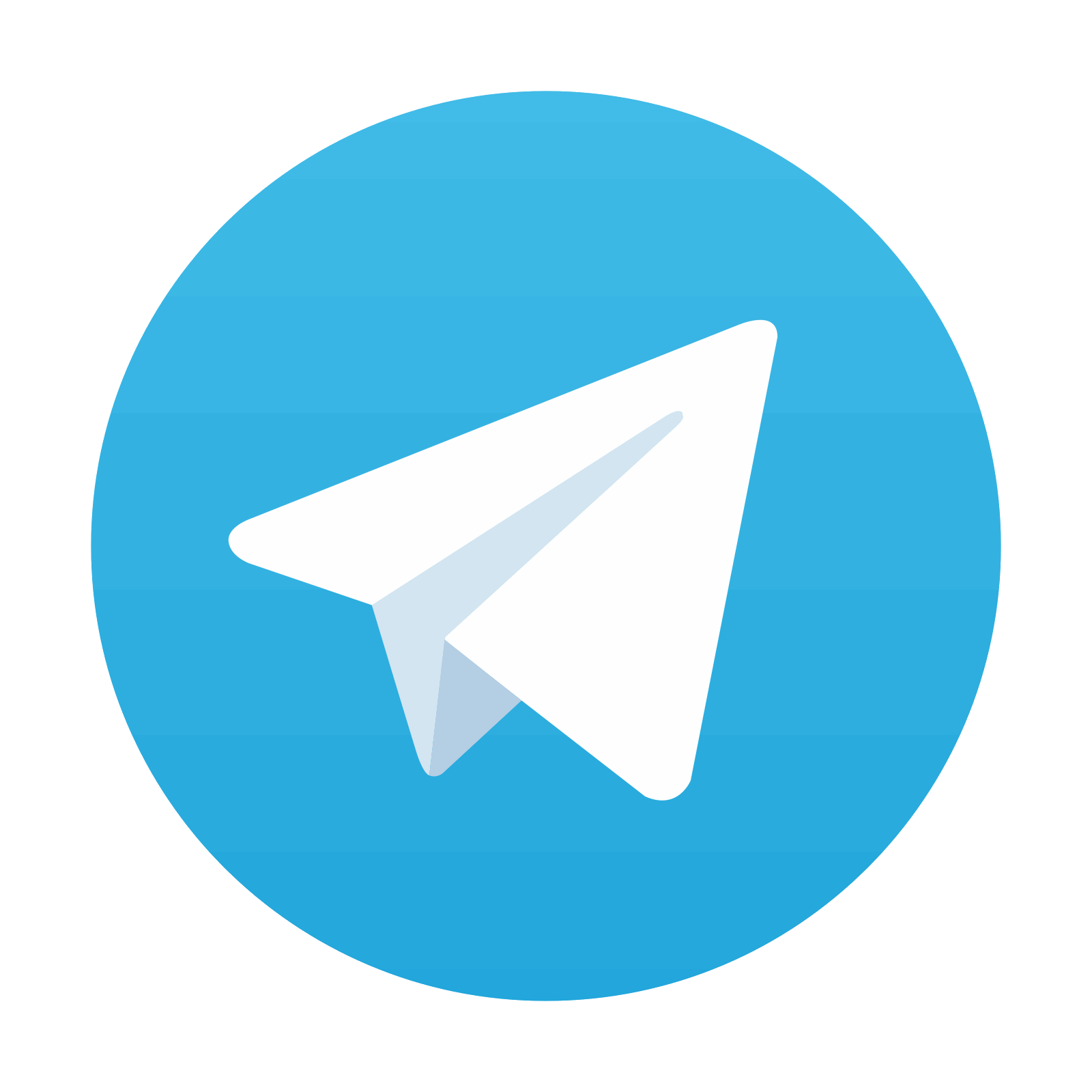
The capsule: The lens capsule is a collagenous membrane that surrounds the lens and provides support by elastic tension.
The lens epithelium: This structure is located in a layer beneath the anterior capsule. The cells of this structure provide homeostatic support and are regenerative. As they age, they migrate to the lens equator, compress into an elongated form, lose their nucleus, and become new lens fibers.< div class='tao-gold-member'>Only gold members can continue reading. Log In or Register a > to continue
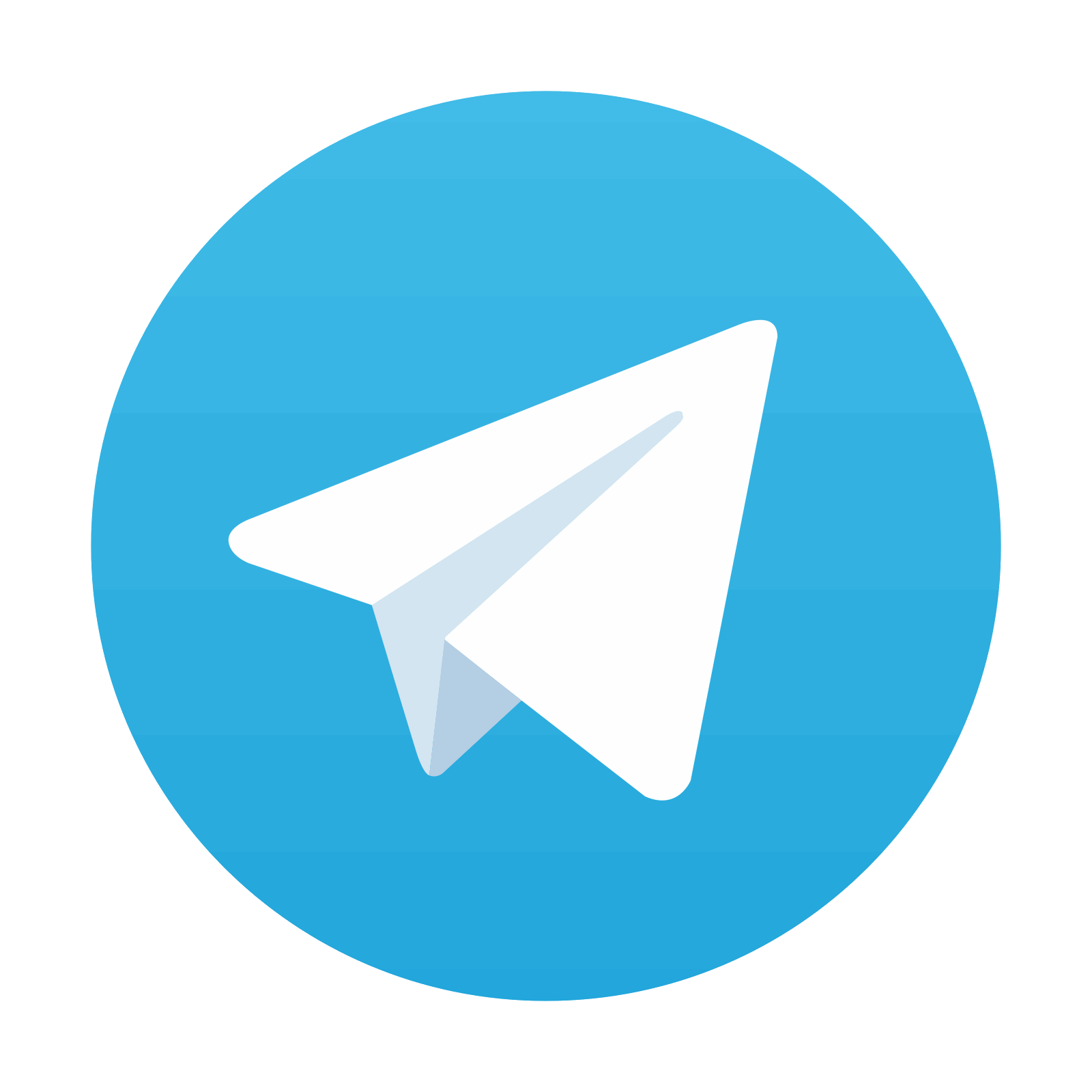
Stay updated, free articles. Join our Telegram channel

Full access? Get Clinical Tree
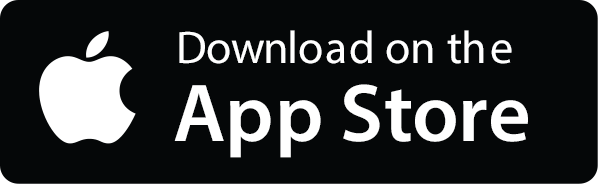
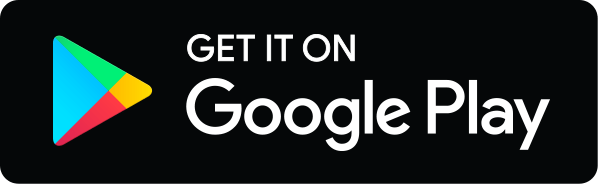