© The Author(s) 2016
Arpan Bhagat, Giorgia Caruso, Maria Micali and Salvatore ParisiFoods of Non-Animal OriginSpringerBriefs in Molecular Science10.1007/978-3-319-25649-8_22. Clostridium Botulinum and C. perfringens in Vegetable Foods: Chemistry of Related Toxins
Abstract
The problem of food-borne human diseases by Clostridium botulinum and C. perfringens remains one of the most interesting research arguments in the field of food technology. Actually, there are two different lines of research depending of the peculiar microorganism and related food-borne disease outbreaks. The clinical syndrome of Botulism is related to C. botulinum and a few other species of the same genus. Botulism is caused by botulinum toxin, a potent neurotoxin with the capability of attacking neuromuscular synapses. 90 % of the total number of episodes is correlated to vegetable foods and preparations. Because of the variability of C. botulinum (three different subtypes are reported at present), four different neurotoxins at least are known with concern to Botulism. Basically, these molecules are zinc-proteins with endopeptidasic activity; the general structure is functional with reference to the attacking mechanism to pre-synaptic receptors of human or animal cells. At the same way, C. perfringens should be considered: the detection in unwashed vegetables and soups by vegetable ingredients may not be excluded. C. perfringens is reported to have five different subtypes: one of these bacteria is particularly lethal because of the production of 10 different toxins, one enterotoxin and one neuraminidase. All these molecules are associated to a specified and maybe lethal pathological action.
Keywords
Clostridium botulinumClostridium perfringensEndotoxinsExotoxinsFood additivesFood-borne botulismNeurotoxinsPasteurisationSterilisationAbbreviations
HC
Carboxy-terminal
CDC
Centers for Disease Control and Prevention
ELISA
Enzyme-linked immunosorbent assay
H
Heavy
MW
Molecular weight
HN
Nitrogen-terminal
NaCl
Sodium chloride
USA
United States of America
Aw
Water activity
2.1 Introduction to Biological Toxins
The action of pathogenic bacteria is mediated by the production of specific virulence factors such as toxins: exotoxins (produced mainly by Gram-positive agents) and endotoxins (produced only by Gram-negative bacteria).
Exotoxins are proteins with molecular weights (WM) ranging from 10 to 900 kDa. These molecules are often controlled by extrachromosomal genes (plasmids) excreted into the surrounding medium (Aoki 2003; Di Bonaventura 2011; Rackley and Abdelmalak 2004). They can also be released after cell lysis; subsequently, the binding to specific cellular receptors (cell specificity) can be observed, with toxic or lethal effects for host cells. Exotoxins have a high toxicity for animals; in addition, these proteins can become highly antigenic (immunogenic) stimulating the formation of neutralising antitoxins. Antitoxins are non-pyrogenic substances: these molecules can be converted to toxoids or anatoxins after treatment with formalin, acids or under heating conditions (Di Bonaventura 2011).
A further classification of exotoxins is made on the basis of two different criteria: the specificity of the target (cell tropism) and the mechanism of action (Middlebrook and Dorland 1984). With relation to targets and related specificity, the following classification can be proposed:
Neurotropic toxins, if they act at the level of central or peripheral nervous system. Examples: tetanus and botulinum toxins
Enterotoxins, if they act on the intestinal mucosa. Examples: Cholera toxin, enterotoxin of Escherichia coli
Pantropic toxins, if the diffusion of cellular receptors is observed. Examples: diphtheria, pertussis, Shiga toxins.
With concern to observed mechanisms of action, the following types can be identified (Kotb 1995; Middlebrook and Dorland 1984):
(1)
Superantigens (type I toxins). These proteins, produced by Staphylococcus aureus and Streptococcus pyogenes, are able to stimulate the immune response even with a concentration of 10–15 g/ml. In fact, superantigens can notably stimulate the immune response
(2)
Exotoxins (type II toxins). These molecules can damage membranes of the host cell by means of the production of spores in the cell membrane. They can also digest cellular materials and alter the composition of membranes
(3)
AB-toxins and other toxins (type III toxins). These substances can interfere with vital functions of the host cell.
With concern to C. perfringens, related exotoxins are reported to have haemolytic and necrotising effects. In addition, the relaxation of tissues, caused by the by gas produced by the fermentation of carbohydrates and the consequent production of gaseous substances, can favour the spread of gas gangrene (myonecrosis). Moreover, a mixed form of diarrhoea-dysentery may be ascribed to the action of these toxins. Anyway, C. perfringens exotoxins are usually subdivided in the following way (Bakker 2012; Carli 2009; McDonel 1980):
(1)
α-toxin (lecithinase). This toxin is reported to increase the permeability of capillaries and muscle cells by destroying the lecithin present in the cytoplasmic membrane. This phenomenon may cause edema when myonecrosis is reported
(2)
κ-toxin (collagenase). It is considered responsible for injuries of soft gas gangrene. The production of collagenase is due to the degradation of connective tissues and muscles
(3)
µ-toxin (hyaluronidase). The production of this molecule is due to the breakage of intercellular bonds in the tissue.
Dimeric AB-toxins can be chemically subdivided in two parts: the active part A and the binding component B. These toxins are produced by both Gram- positive and Gram-negative bacteria and secreted in external environment; the component B is responsible for the target specificity because it can bind to the receptor surface of the host cell. Subsequently, the AB-toxin can be transferred across the membrane for endocytosis, whereby the component A is separated from B to migrate into the cytoplasm. However, certain AB-toxins can partially enter into host cells by means of the introduction of the component A only through a pore membrane.
The main host defence against toxins is represented by the production of specific toxin antibodies. After the connection to the antibody, the toxin can no longer bind to the cell surface receptor. Good examples of AB-toxins are the enterotoxin of C. perfringens and botulinum toxin of C. botulinum.
C. botulinum produces an endopeptidase: this substance is able to block the release of acetylcholine at the neuromuscular junction. Botulinum toxin cleaves proteolitically synaptobrevin interfering with the formation of synaptic vesicles. The result of this action is flaccid paralysis (bilateral descending weakness of peripheral muscles, paralysis of respiratory muscles with consequent death); the toxin is called ‘neurotropic’ because it interferes with the release of neurotransmitters (Karalewitz and Barbieri 2012; Carli 2009).
2.2 Clostridium Botulinum and Related Toxins
C. botulinum is a rod-shaped bacterium with Gram-positive features when speaking of young cultures. It produces a protein toxin that can cause flaccid paralysis of botulism in humans and animals, also defined neurotoxin (CDC 1998). The ability to synthesise the botulinum neurotoxin is the only common feature that unifies all life forms of this species, even though these are very heterogeneous. Following the isolation of other strains of C. botulinum from clinical (human), food and environmental samples, researchers have deducted that botulinum toxins produced by these strains are not identical, in spite of the similarity of botulism diseases and related mechanisms of action. In fact, antisera produced against a specific toxin have been recognised unable to neutralise the toxicity of other types. At present, seven different antigenic variants of botulinum neurotoxins—named with capital letters from A to G—have been reported (CDC 1998). Botulinum A, B, E and rarely F toxic types cause botulism in humans, while animal botulism is caused by botulinum C and D toxins; in addition, botulism is not apparently associated with the remaining botulinum G toxin, until now.
In the most part of situations, C. botulinum X (where X = A, B, C and so on) is reported to produce one toxin type only: this substance is named with the same capital letter. For instance, the botulinum toxin F is produced by C. botulinum type F. However, there are also rare C. botulinum strains which can produce two toxins at the same time, including new types (Barash and Arnon 2014): generally, one of the two toxic molecules is produced in greater quantities than the other substance.
Another type of microbial classification can be considered with concern to the subdivision of life forms (species) according to their metabolic and physiological features (CDC 1998). In detail, four different groups have been considered: each category includes strains with similar properties, even if they produce neurotoxins of different types.
2.2.1 Proteolytic and Non-proteolytic Clostridia
A selected group of life forms—‘proteolytic’ strains—can obtain metabolic energy by means of the simple scission of proteins, while other ‘non‐proteolytic’ life forms are accustomed to use sugars by means of different mechanisms. Just after the production of catabolites, foods contaminated with C. botulinum usually assume more or less unpleasant organoleptic characteristics, depending on the peculiar food matrix (CDC 1998; Lund and Peck 2013).
Life forms of these two groups also differ in their physiological features. All life forms are mesophilic but:
(a)
Proteolytic clostridia grow at temperatures up to 40 °C (on the other hand, they are not able to live below 10 °C)
(b)
Non-proteolytic life forms can survive and increase their number at 3.3 °C, although optimal temperatures are reported to be around 30 °C.
As a result, the environmental distribution of strains is different. The first group (proteolytic strains) is reported to be normally found in dry and temperate geographical areas, while the latter category non-proteolytic life forms—can be often observed in more humid and cold climates. Furthermore, spores of the first group (proteolytic species) have a higher resistance to chemical (pH, water activity, salt concentration) and physical (temperature and oxidation-reduction potential) conditions, compared to those of the second group. In other words, the enhanced resistance of proteolytic strains can explain the survival of life forms in extreme environmental conditions, with some possible sanitary reflection when speaking of food safety and teÉchnology problems (food preservation).
2.2.2 Animal Botulism-Related Strains and Asaccharolytic Clostridia
Strains which cause botulism in animal species have features. In brief, they grow with difficulty only in conditions of strict anaerobiosis. In addition, these life forms produce few spores. Moreover, differently from other C. botulinum strains, these clostridia are strongly haemolytic if cultured on blood agar plates because of the known haemolysin activity. These strains are also capable of producing two botulinum toxins other than those defined as C2 and C3. This classification is needed because of the existence of another type C neurotoxin. Actually, the role of these strains in the pathogenicity has to be clarified yet.
Finally, C. botulinum type F remains to be considered. These strains are distinguished because they are completely asaccharolytic (José et al. 2014) and do not produce lipases. However, their physiological proÉperties are not completely known for two reasons: these asaccharolytic life forms have been isolated recently (in the 1970s); in addition, no episodes of botulism have been ascribed to toxin-type F until now.
2.3 Botulinum Neurotoxin
The main route of entry for toxins is generally correlated with the digestive system. For this reason, toxins should show notable resistance to proteolytic reactions and denaturation into the gastric apparatus. Otherwise, toxic molecules could not remain in the intestinal tract.
By the chemical viewpoint, the solution appears linked to the dimension of toxins: similar substances are produced as complex systems (MW: 300, 500 and even 900 kDa) with associated non-toxic proteins that allow them to resist in the gastric environment. Because of the instability of the multimeric complex at alkaline pH values (in the intestinal tract), the toxin is easily released and subsequently absorbed into the bloodstream.
Similarly to tetanus toxin, botulinum toxins are metal proteins with recognised endopeptidase activity: the metal is zinc (Schiavo et al. 1992). The general structure is shown as a double chain with MW of about 150 kDa (approximate value). The double chain can be subdivided (CDC 1998) in a heavy (H) structure of 100 kDa and a lighter (L) chain of 50 Kda; chemical structures are linked together by means of a disulfide bridge (non-covalent interactions are not reported). The heavy chain is in turn constituted by the nitrogen-terminal (HN) and the carboxy-terminal (HC) domains. The L chain performs the catalytic function of the toxin while HC domain (on the H structure) is responsible for binding action to pre-synaptic receptors for internalisation. Moreover, HN is also called ‘translocation domain’: in fact, the translocation of the L chain from the lumen to the endosomal cytoplasm of cell is ascribed to this domain (CDC 1998).
Botulinum toxins are produced in an inactive form as single polypeptide chains of about 150 kDa; later, a proteolytic cut generates the previously described active double-chain form. In many situations, the cut is carried out by proteases produced by clostridia themselves. However, some strains do not possess these enzymes: as a result, toxins may be released in the single-chain form; the subsequent activation is performed by other proteases in the host tissue.
H and L chains of various serotypes are composed of 840 and 430 amino acids, respectively (average estimation); in addition, these structures show homologous regions separated by other regions with little or no homology. The most preserved segments of the L chain are represented by one hundred of amino terminal residues; moreover, the central area contains the ‘HExxH’ zinc—protease consensus sequence (Schiavo et al. 1992). On the other side, the H chain is the less preserved structure, especially in the carboxy-terminal part. Finally, cysteine units are totally preserved: their role is related to the formation of the inter-chain disulfide bridge.
The determination of the crystallographic structure of botulinum neurotoxins, types A and B (Lacy et al. 1998; Swaminatan and Eswaramoorthy 2000), reveal that these structures are composed of three distinct domains. The presence of a peculiar handle in the HN domain should be considered in fact, the catalytic domain remains inaccessible to the active site because of the protection offered by this area around the perimeter.
2.3.1 Binding Domain of HC
The crystallographic structure of the HC domain reveals a structural subdivision in two subdomains. The first of them can be defined nitrogen-terminal subdomain (HCN) while the other may be named carboxy-terminal subdomain (HCC).
HCN is composed of two sheets with a peculiar ‘jelly-roll’ topology (Gallego del Sol et al. 2002), similarly to certain legume lectin crystal structures (carbohydrate-binding proteins). This subdomain is highly preserved in clostridial toxins.
The HCC subdomain is formed from a pattern called ‘β-trefoil’ structural motif. This feature is present in various proteins involved in ‘recognition’ and binding functions such as interleukin-1, fibroblast growth factors and Kunitz-type trypsin inhibitor. The amino acid sequence of this subdomain is poorly preserved between clostridial neurotoxins (Montecucco et al. 2004).
2.3.2 The HN Translocation Domain
This portion of the toxic molecule has a high homology in various serotypes; the same thing can be affirmed with relation to the expected secondary structure. The central part is formed by a pair of 10 nm-helices: the complete structure is similar to certain proteins that interact with membranes. Two similar examples are colicine and hemagglutinin of influenza virus: these proteins are able to show structural variations when placed in acidic environments. As previously mentioned, an important feature of HN domain is the existence of a loop wrapped around the catalytic domain with the consequence of preventing the catalytic activity (Lacy et al. 1998).
2.3.3 The Catalytic Domain
The light L chain is the part of the toxin with catalytic activity. This domain consists of a set of helices and sheets with the helix of the zinc binding motif in the central portion. The central helix consists of a peculiar amino acid sequence preserved in all zinc—peptidase systems: this structure is generally named ‘histidine-glutamic-XX-histidine’ (HExxH), where H stands for histidine, E means glutamic acid and X stands for a generic amino acid (Schiavo et al. 2000). Involved amino acids, whose chains appear to be closer to zinc, are: His 223, Glu 224, His 227, Glu 262 and Tyr 366. Imidazolic rings of histidine are intended to interact with zinc as well as the side chain of Glu 262. The amino acid Glu 224 is particularly important because of the coordination of a water molecule which is needed in the hydrolysis reaction of the peptide bond of the targeted protein (Rossetto et al. 2004). The organisation of the active site is similar to that of thermolysin (Morante et al. 1996). With relation to Tyr 366, the phenolic ring is not completely located behind the metal: the distance is about 5 Å. It may be supposed that this ring performs functions of coordination with the substrate: substitutions of this amino acid with glycine have demonstrated an inability of proteolysis of the toxin. In conclusion, we can say that the structural organisation of botulinum toxins collimate perfectly with their mechanism of action. This mechanism is based on four key events (Montecucco and Schiavo 1994):
(1)
Binding to receptors
(2)
Internalisation
(3)
Translocation into the cytoplasm
(4)
Enzymatic modification of the target.
Once entered into a body and introduced in the body fluids, toxins are able to arrive at the muscle junctions. Subsequently, after the internalisation step, they can perform the typical toxic activity on nervous impulses: the blockage of transmissions.
2.4 Different Forms of Botulism
Botulism is a serious neuroparalytic syndrome that affects both man and animals, caused by botulinum neurotoxin in neuromuscular synapses. The toxic activity is expressed by blocking the release of neurotrasmitter acetylcholine; the subsequent step is the blockage of nervous transmissions at the neuromuscular junction (flaccid paralysis). Signs and symptoms of the disease are those characteristic of bulbar palsy with typical symmetrical descending trend and possible respiratory paralysis, in severe situations (Montecucco and Schiavo 1994).
Actually, the diversity of clinical diseases is strictly correlated with the collection of epidemiological data in different Countries and the difficult estimation of the actual incidence. Basically, the availability of data on botulism (incidence and prevalence, clinical forms and causes) depends on the existence of a surveillance system. Botulism has to be obligatorily notified in many industrialised countries; however, the exact moment of notification is variable.
2.4.1 Wound Botulism
Wound botulism is an infectious form reported for the first time in the United States of America (USA) about 50 years ago, due to the germination of C. botulinum spores and toxin formation in wounds. Neurological symptoms of flaccid paralysis are not different when compared with food-borne botulism except for some unique features (CDC 1998):
(a)
It is a very rare event, involving a single case per situation
(b)
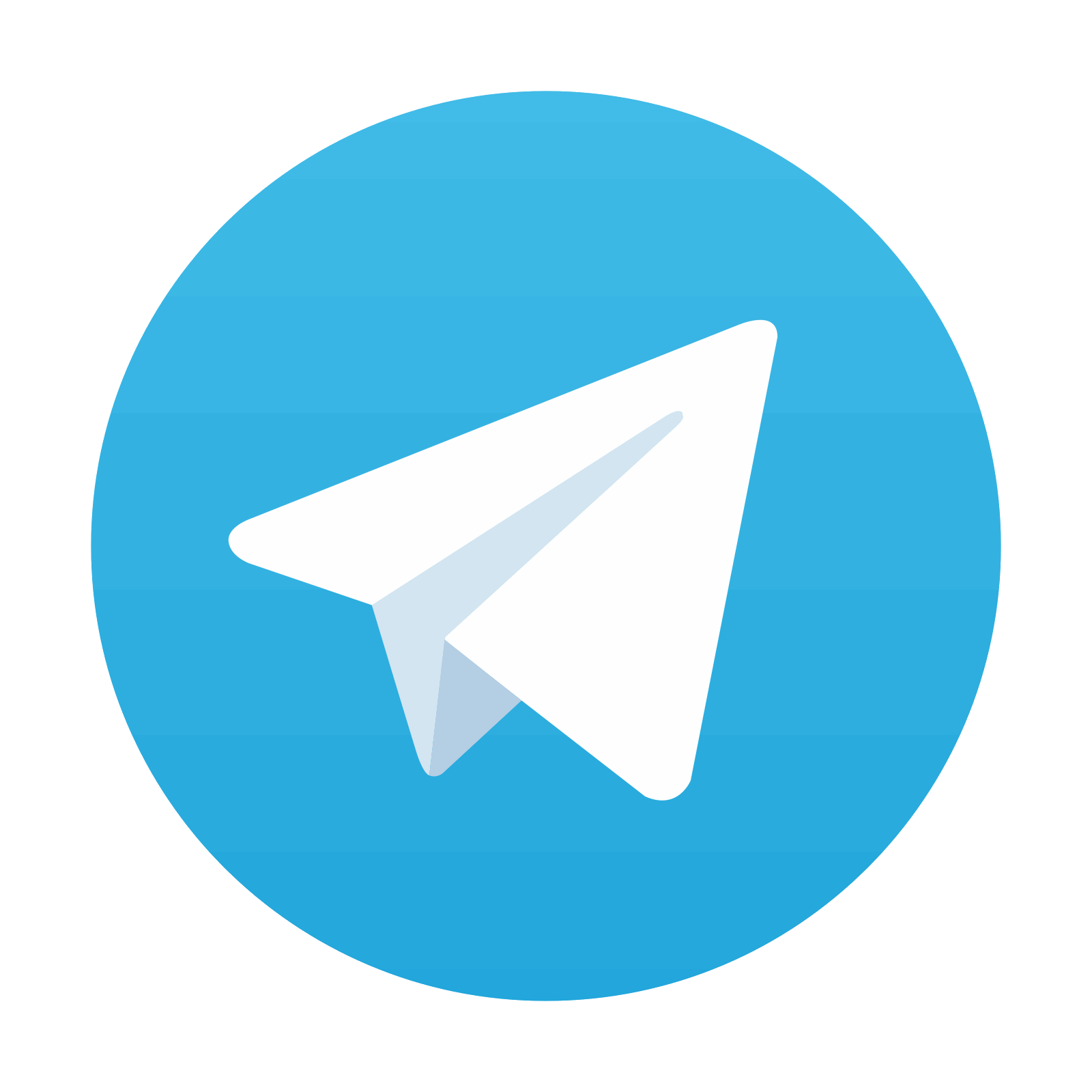
The incubation period is longer compared to food-borne disease (between four and 14 days)
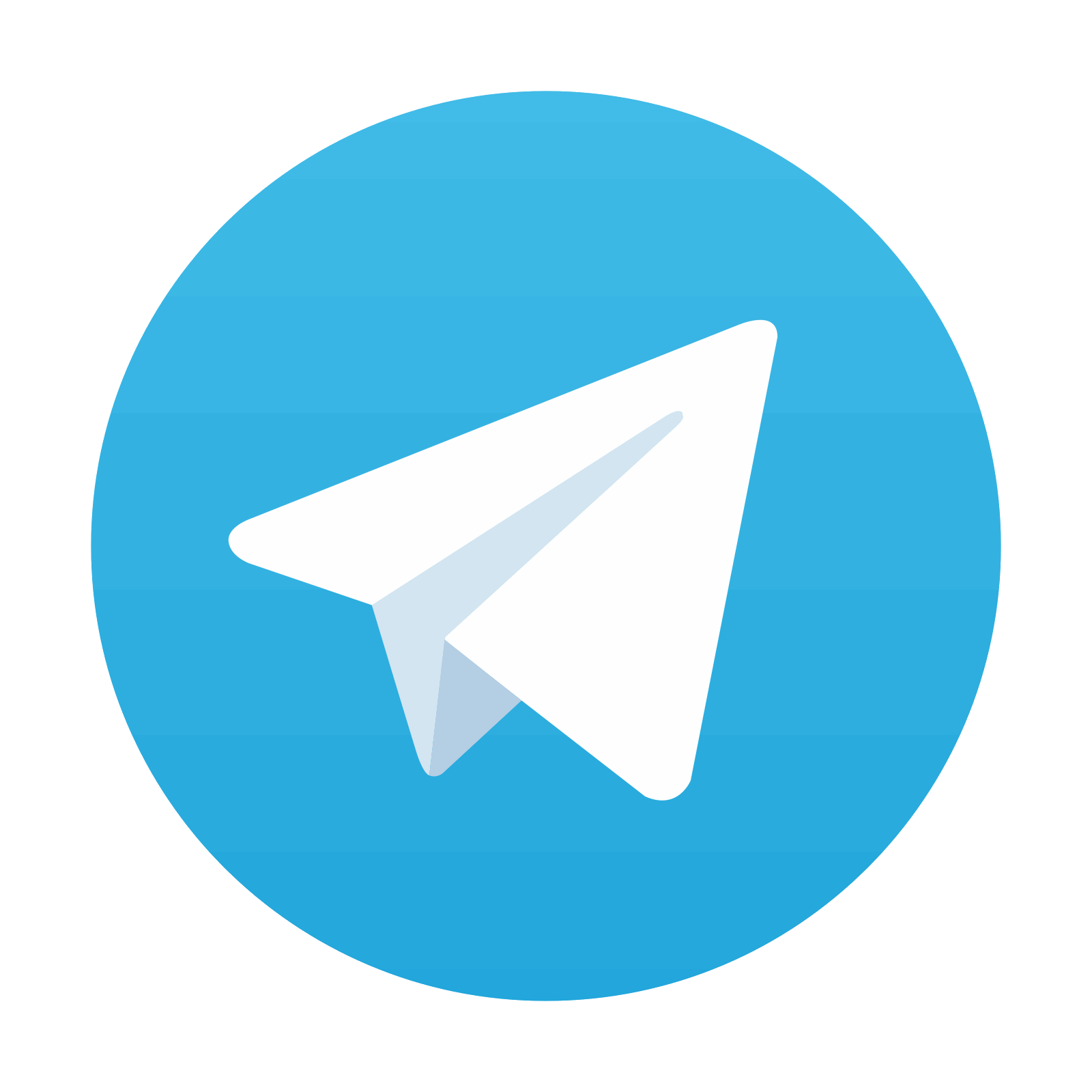
Stay updated, free articles. Join our Telegram channel

Full access? Get Clinical Tree
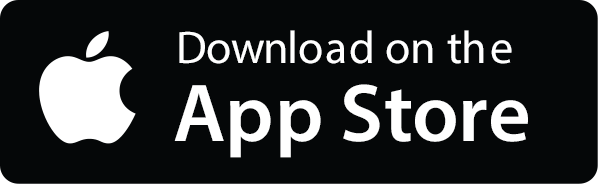
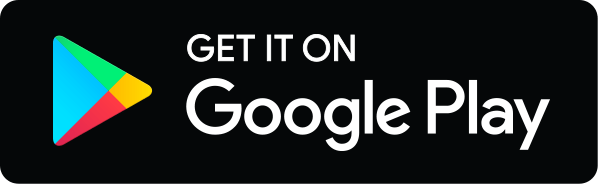
