Chapter 5 Chronic Renal Failure
Introduction
A. Chronic renal failure (CRF) occurs when the compensatory mechanisms of chronically diseased kidneys are no longer able to maintain adequate functions to excrete waste products; regulate electrolyte, water, and acid-base homeostasis, degrade hormones; and synthesize endocrine hormones. The resultant retention of nitrogenous solutes; derangements of fluid, electrolyte, and acid-base balance; and failure of hormone production constitute the syndrome of CRF.
1. Azotemia does not develop until 75% or more of the nephron population has become nonfunctional. Isosthenuria occurs when greater than 66% of the nephron population has become nonfunctional; the ability to elaborate maximal urine concentration is lost earlier.
B. Renal functions affected are:
1. Excretory: Retention of solutes handled by glomerular filtration rate (GFR) (e.g., urea, creatinine).
2. Regulatory: Derangements in solutes handled by GFR and some combination of reabsorption and secretion can result in abnormalities of water, electrolytes, and acid-base balance. Depending on the solute in question, retention or loss can result.
3. Degradative: Many small peptides (e.g., gastrin) normally are filtered by the kidney, reabsorbed, and degraded in the proximal tubular cells. Loss of this clearance function can result in metabolic derangements because many of these small peptides are hormones.
C. International Renal Interest Society (IRIS) staging of chronic kidney disease (CKD).
1. IRIS is an international special interest group seeking to identify and disseminate information on better methods for the diagnosis and treatment of dogs and cats with renal disease.
2. The term CKD is recommended over chronic renal disease (CRD) or CRF to avoid confusion during conversations with pet owners.
3. IRIS has proposed a staging system for CKD based on the serum creatinine concentration of the stable patient on at least two occasions (Table 5-1). A substage then is assigned based on whether or not the animal is proteinuric (Table 5-2) and the absence or presence (and extent) of hypertension (Table 5-3).
a. Serial measurements of serum creatinine concentration should be made by the same laboratory. Interassay variability for creatinine determinations is minimal within a given laboratory but not among laboratories.
4. Staging of CKD should allow more rational treatment strategies to be employed based on stage of disease. The goal of treatment is to slow or prevent progression of disease.
a. Most studies of treatment for CKD have been reported for dogs and cats with overt azotemia and clinical signs (i.e., IRIS stages 3 and 4; see Table 5-1).
5. The low cutoff values for maximal serum creatinine concentration considered to be normal will allow identification of more animals with CKD but also occasionally will include normal animals (see Table 5-1).
TABLE 5-1 Serum Creatinine Concentrations for Assignment of International Renal Interest Society Stage of Chronic Kidney Disease in Dogs and Cats
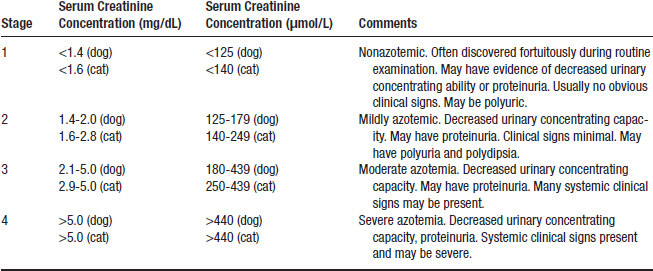
TABLE 5-2 Proteinuria∗ for Assignment of International Renal Interest Society Substage of Chronic Kidney Disease in Dogs and Cats
Urine Protein/Creatinine Ratio | Classification |
---|---|
<0.2 (dogs) <0.2 (cats) | Nonproteinuric |
0.2-0.5 (dogs) 0.2-0.4 (cats) | Borderline proteinuric |
>0.5 (dogs) >0.4 (cats) | Proteinuric |
∗ Assessed by urine protein/creatinine ratio.
TABLE 5-3 Systemic Blood Pressure for Assignment of International Renal Interest Society Substage of Chronic Kidney Disease in Dogs and Cats
Systolic Blood Pressure (mm Hg) | Diastolic Blood Pressure (mm Hg) | Risk Level |
---|---|---|
<150 | <95 | Minimal |
150-159 | 95-99 | Low |
160-179 | 100-119 | Moderate |
≥180 | ≥ 120 | High |
6. Many laboratories report reference ranges for serum creatinine concentration much wider than those proposed in the IRIS guidelines and some animals with serum creatinine concentrations at the upper end of such reference ranges likely have CKD.
7. Guidelines for serum creatinine concentrations are based on average-sized adult dogs. Extremely small or large dogs may have lower or higher normal serum creatinine concentrations. Breed-specific results for serum creatinine concentration are not addressed in the IRIS guidelines (e.g., normal Greyhounds tend to have higher serum creatinine concentrations than other breeds). Also, young dogs normally have lower serum creatinine concentrations than mature dogs.
8. Prerenal and postrenal conditions also must be excluded based on results of history, physical examination, urinalysis, and urinary tract imaging as necessary.
9. Complete IRIS classification requires assessment of proteinuria (see Table 5-2) and systemic blood pressure (see Table 5-3).
Causes of Chronic Renal Failure in Dogs and Cats
Dog
2. Chronic pyelonephritis (can be difficult to distinguish histologically from chronic tubulointerstitial nephritis).
3. Chronic glomerulonephritis (can be difficult to distinguish histologically from chronic tubulointerstitial nephritis).
4. Amyloidosis (familial in Shar-pei dogs; see Chapter 6, Familial Renal Diseases of the Dog and Cat).
Cat
2. Chronic pyelonephritis (can be difficult to distinguish histologically from chronic tubulointerstitial nephritis).
3. Chronic glomerulonephritis (can be difficult to distinguish histologically from chronic tubulointerstitial nephritis).
4. Amyloidosis (familial in Abyssinian cats; see Chapter 6, Familial Renal Diseases of the Dog and Cat).
5. Polycystic kidney disease (familial in Persian cats; see Chapter 6, Familial Renal Diseases of the Dog and Cat).
Pathophysiology of Chronic Renal Failure
Uremia
1. Uremia is a syndrome that occurs after substantial reduction in renal function so that azotemia develops in conjunction with clinical signs. A variety of laboratory findings (hematologic, endocrine, serum biochemistry) usually accompany clinical signs recognized by the owner (lethargy, weight loss, decreased appetite, and vomiting).
2. A uremic toxin is any compound that accumulates in excess due to decreased renal function and contributes to the clinical signs of uremia.
a. Urea, guanidine compounds, products of bacterial metabolism (e.g., polyamines, aliphatic amines, indoles), myoinositol, trace elements, and “middle molecules” (i.e., some hormones and other compounds with molecular weights of 500-3000) have been considered as potential uremic toxins.
b. Many compounds are involved in the pathophysiology of uremia, and no single compound is likely to explain the diversity of uremic symptoms.
Hyperfiltration
1. Hyperfiltration occurs as a result of an increase in single nephron GFR (SNGFR) above the normal range in surviving remnant nephrons. In most instances, increased SNGFR also is associated with increased transglomerular pressure (Figures 5-1 and 5-2).
a. A fixed and dilated afferent glomerular arteriole allows delivery of increased pressure to the glomerular capillaries.
b. Although not well documented, efferent arteriolar vasoconstriction could contribute to increased transglomerular pressure.
c. Intraglomerular hypertension is a term used to describe increased hydrostatic pressure in remnant nephron glomerular capillaries. Intraglomerular hypertension is not necessarily related to the presence of systemic hypertension.
d. Increased glomerular volume in glomeruli of remnant nephrons also may contribute to hyperfiltration, but does not necessarily increase glomerular pressure. Increased glomerular volume itself may contribute to adverse consequences such as proteinuria.
2. Renal disease tends to be inexorably progressive once a critical number of nephrons has been destroyed, even when the initial cause of renal injury has been removed.
3. Total GFR represents the sum of the SNGFR for all nephrons of both kidneys. There are approximately 1,000,000 nephrons in a dog (500,000 per kidney) and 400,000 nephrons in a cat (200,000 per kidney). In a healthy animal, the range of SNGFR is fairly narrow.
4. During progressive renal disease, the decline in total GFR is offset by an increase in SNGFR in functional remnant nephrons (so-called glomerular hyperfiltration). Thus, the normally narrow range of SNGFR widens during development of CRF because diseased nephrons have low SNGFR and remnant nephrons have supra-normal SNGFR. Glomerular hyperfiltration has been incriminated as one important factor contributing to the progressive nature of renal disease based on experimental studies in animals.
5. Glomerular hyperfiltration has been demonstrated to occur experimentally in dogs and cats and in the few clinical dogs and cats it which it was measured. It is assumed that glomerular hyperfiltration is present in azotemic dogs and cats with primary renal disease.
6. The mechanisms responsible for this increase in SNGFR result from alterations in the normal determinants of SNGFR. In dogs, hyperfiltration results primarily from increases in the glomerular ultrafiltration coefficient (Kf) associated with increased glomerular volume by hypertrophy and increased glomerular capillary plasma flow and increased transglomerular capillary pressure largely due to afferent arteriolar dilatation. Experimentally, dogs and cats develop glomerular hyperfiltration after ablation of 75% or more of renal mass.
7. Following nephron loss, adaptation occurs to recoup lost GFR. This is to such an extent that total GFR will increase approximately 40% to 60% in the remnant renal tissue over a period of 4 weeks after renal ablation. For example, if one kidney is removed from a dog with GFR of 40 mL/min, GFR will immediately decline to 20 mL/min but within one month will stabilize at approximately 30 mL/min due to the effects of hyperfiltration in remnant nephrons.
8. Proteinuria and glomerular sclerosis in remnant nephrons are adverse functional and morphological consequences of glomerular hyperfiltration that may lead to progressive deterioration of the remaining renal tissue. These glomerular lesions are more prominent in rats with experimental renal disease than in dogs and cats in which tubulointerstitial lesions predominate. Also, proteinuria often is mild in dogs and cats with experimental renal disease and its contribution to progression is less certain than in rats.
9. The extent of renal damage that results in progressive deterioration of the remnant kidney is not known for all species. In dogs, 85% to 95% of renal tissue must be destroyed to result in progression, whereas progression occurs in rats after 75% to 80% renal ablation. Cats with 83% reduction in renal mass did not show evidence of progression over a one-year period of time in an experimental study.
Factors Contributing to the Progression of Chronic Renal Disease
Species Differences
a. Clinical experience indicates that naturally occurring renal disease often is progressive in dogs. In experiments in dogs with subtotal nephrectomy, renal disease may not become progressive until 85% to 95% reduction in renal mass has occurred.
b. Limited information is available in cats. Naturally occurring renal disease often is progressive in cats, but it seems to progress much more slowly than in dogs. Cats with experimentally induced renal disease (5/6 nephrectomy) showed minimal evidence of progression over a 1-year period of time, but longer study may be needed to identify progression.
Functional and Morphologic Changes in Remnant Renal Tissue
a. Hyperfiltration increases movement of protein across the glomerular capillaries into Bowman’s space and the mesangium, a process referred to as “protein trafficking.”
b. Increased filtration of proteins through the glomeruli is toxic to the kidney and may contribute to renal disease progression (Figure 5-3). The magnitude of proteinuria correlates with rate of progression of renal disease in dogs and cats but whether or not proteinuria causes progression or is simply a marker of progression is not resolved.
(1) The increased filtered load of protein may overload the proximal tubular cells leading ultimately to rupture of lysosomes and exposure of tubular cells and renal interstitium to damaging enzymes.
(2) Tubular cells possess receptors for hormones and growth factors, some of which are small molecular weight proteins (e.g., insulin-like growth factor 1 [IGF-1], transforming growth factor β) that are filtered excessively and taken up by the proximal tubular cells where they may promote cellular proliferation and extracellular matrix deposition leading to tubulointerstitial damage.
c. Increased filtration of serum proteins also occurs into the mesangial space, which results in proliferation of mesangial cells and accumulation of increased mesangial cell matrix, both of which contribute to the development of glomerulosclerosis.
d. Accumulation of lipids in the mesangium also may promote mesangial cell proliferation, excess production of mesangial matrix components such as collagen and proteoglycans and ultimately glomerular sclerosis.
f. Typical lesions of CKD in dogs and cats include a varying degree of lymphoplasmacytic tubulointerstitial nephritis, fibrosis, tubular dropout, hypertrophy of some tubular epithelium, mineralization of tubular basement membrane and interstitium, glomerulosclerosis, and obsolescent glomeruli (Figures 5-4 and 5-5).
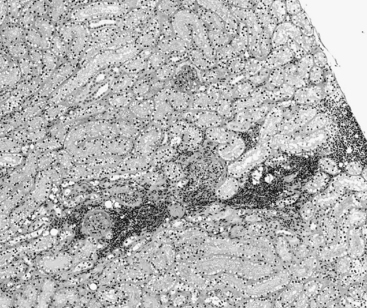
FIGURE 5-4 Chronic renal disease histopathology–early lesions. (Hematolxyin and eosinstain, x600).
(Courtesy of Dr. Steven Weisbrode, Columbus, Ohio.)
Systemic Complications
a. Systemic complications of renal insufficiency may affect progression.
(4) Unidentified uremic toxins likely contribute to renal disease progression because nonspecific adsorbent treatment ameliorates progression in rats with experimentally induced renal disease and humans with naturally occurring CRF.
(5) Therapeutic interventions with modified dietary intake, phosphorus binders, angiotensin-converting enzyme (ACE) inhibitors and other antihypertensive agents, and treatment of secondary hyperparathyroidism with calcitriol also potentially can alter clinical signs and progression of CRF (see “Treatment”).
Solute Balance
1. Despite distortion of renal architecture by disease, glomerular and tubular functions in remaining functional nephrons are as closely integrated in diseased kidneys as in normal kidneys (i.e., glomerulotubular balance is maintained).
2. For any given solute, the diseased kidney maintains glomerulotubular balance as GFR declines by decreasing the fraction of the filtered load of that solute that is reabsorbed and increasing the fraction of the filtered load of that solute that is excreted.
3. In some instances, the mechanisms of the adaptive changes have adverse effects on the animal as explained by Neil Bricker’s trade-off hypothesis: “The biological price to be paid for maintaining external solute balance for a given solute as renal disease progresses is the induction of one or more abnormalities of the uremic state.”The classic example of the trade-off hypothesis is maintenance of normal calcium and phosphorus balance at the expense of renal secondary hyperparathyroidism and bone demineralization. Other examples include bone buffering by carbonate at the expense of bone demineralization, and increasing SNGFR by glomerular hyperfiltration at the expense of proteinuria, glomerular sclerosis, and progressive destruction of remnant renal tissue.
4. Some of these ultimately maladaptive mechanisms and their consequences can be prevented by a proportional reduction in the intake of the responsible solute. This strategy will avoid the need for the kidneys to alter the fractional reabsorption and excretion of the solute in question. Using this approach with dietary phosphorus in patients with CRD has been shown to prevent or reverse renal secondary hyperparathyroidism.
Water Balance
1. Both the ability to produce concentrated urine (i.e., to conserve water) and the ability to excrete a water load are impaired in CRF.
2. The development of this concentrating defect is noted clinically by the onset of polyuria and compensatory polydipsia (more so in dogs than in cats).
3. Increased solute load per residual functioning nephron rather than architectural damage to the tubules and interstitium probably is the most important factor contributing to the concentrating defect (i.e., the remnant nephrons experience an osmotic diuresis).
4. Another contributing factor is the limited ability of the distal nephron to respond to antidiuretic hormone (ADH), possibly due to higher tubular flow rate in remnant nephrons.
5. In most instances, impairment of concentrating ability develops when 67% of the nephron population has become nonfunctional. This concentrating defect is recognized clinically by the presence of isosthenuria (urine osmolality of 300-600 mOsm/kg or urine specific gravity [USG] of 1.007-1.015) when obvious azotemia has developed.
6. For unknown reasons, some cats with CRF retain considerable concentrating ability even after development of azotemia. In one study, cats with 58% to 83% loss of functional nephrons could produce concentrated urine (USG 1.022-1.067). Thus, cats with azotemia and relatively concentrated urine do not necessarily have prerenal azotemia.
7. Urinary concentrating ability also may be preserved in the presence of renal azotemia in some dogs with CRD and early CRF. In these instances, GFR is decreased but postglomerular blood flow is thought to be sufficiently preserved so that concentrated urine still can be produced.
8. In some diseases, damage to the distal nephron or distortion of the normal architecture of the medullary interstitium may play a more important role in the concentrating defect and accounts for the early appearance of impaired concentrating ability (e.g., medullary amyloidosis in cats, pyelonephritis, polycystic renal disease, obstructive nephropathy, hypercalcemic nephropathy).
Diagnosis of Chronic Renal Failure
Clinical History in Chronic Renal Failure
Polyuria and Polydipsia
a. Polyuria and polydipsia may be the first abnormalities noted by observant owners of dogs and cats. Nocturia may be the first thing noticed in dogs when they awaken their owners so as to be allowed outside to urinate during the night. Polydipsia and polyuria frequently are not noticed by owners of cats. Polyuria in cats may be identified if questions about the weight of the litter box are included in the history.
b. Some owners misinterpret polyuria as incontinence or pollakiuria because the animal is urinating in the house when it never did so in the past. The owner must be questioned to differentiate incontinence (lack of voluntary control; dribbling) from polyuria (normal voluntary control; increased volume of urine) and pollakiuria (normal control, urinating small volumes frequently and sometimes accompanied by straining or pain).
Gastrointestinal Signs
a. Vomiting: Common in uremic dogs but much less so in cats. Stimulation of the chemoreceptor trigger zone by a uremic toxin may be the cause. Gastrointestinal ulcers also may contribute, as may increased gastric hyperacidity.
b. Gastroenteritis with hemorrhage (especially in uremic dogs) can occur. Many factors may contribute to uremic gastropathy:
(3) Erosions or ulcers due to ammonia production from urea by bacteria in the gastrointestinal tract are less common in dogs and cats than in humans with CRF.
(6) Increased concentration of circulating gastrin due to impaired renal degradation has been documented in both dogs and cats. Excess gastrin stimulates acid secretion in the stomach and contributes to uremic gastroenteritis.
Weight Loss
Lethargy, Weakness, and Neurologic Signs
c. Uremic encephalopathy.
(1) Uremic encephalopathy may occur when GFR decreases to less than 10% of normal. Encephalopathy is more readily recognized when the onset of uremia is rapid (as in ARF) but it also can be severe in CRF.
(2) Calcium influx in the brain mediated by high PTH concentration may play a role in uremic encephalopathy of CRF. Amino acid alterations due to malnutrition or accumulation of uremic toxins may alter neurotransmitter function. Electroencephalographic abnormalities in dogs with experimentally induced and naturally occurring renal failure have been noted that parallel the severity of the observed clinical signs.
Physical Findings in CRF
Weight Loss Including Loss of Lean Muscle Mass
Poor Hair Coat (i.e., Dull, Dry)
Oral Lesions (More Common in Dogs Than Cats)
b. Erosions and ulcers of the buccal mucosa and tongue (often the lateral margins of tongue) may be observed. Ulcers may be due to excretion of urea into saliva and breakdown to ammonia by oral bacteria.
c. Tongue tip necrosis may result from fibrinoid necrosis and arteritis with focal ischemia, necrosis, and ulceration. This lesion is much more common in dogs than in cats. It is more commonly described in patients with CRF but also can develop in those with ARF.
Abdominal Palpation
Blindness
Systemic Hypertension
1. The prevalence of hypertension in dogs with CKD has been reported to be as low as 9% and as high as 93%, and in cats with CKD the prevalence of hypertension has been reported to range between 19% and 65%. Many factors including differences in the types of renal diseases present, methodological differences, differences in body size, and interaction with human beings, (so-called white coat effect) all likely have contributed to variability in the reported range of prevalence of hypertension in dogs and cats with CKD. We estimate that 20% to 30% of cats and dogs with CKD have systemic hypertension at the time of diagnosis in our hospital, and that another 10% to 20% develop hypertension within a year of the initial diagnosis. Standardization of blood pressure measurement methodology and longitudinal studies will be required to better understand the natural history of hypertension in dogs and cats with CKD.
2. Normal blood pressure in dogs and cats is similar to that of human beings (i.e., systolic 120 mm Hg, diastolic 80 mm Hg). The white coat effect can increase the blood pressure of dogs and cats.
3. Because of white coat artifact, it is difficult to diagnose hypertension in dogs and cats.
a. Some studies have used a systolic blood pressure of >175 mm Hg on 2 occasions or >175 mm Hg in the presence of ocular lesions as indicating hypertension in cats.
b. Dogs with CRF and in-hospital systolic blood pressures of 145 to 165 mm Hg are considered mildly to moderately hypertensive and those with systolic blood pressures of 165 to 200 mm Hg are considered moderately to severely hypertensive. Those with systolic blood pressures >200 mm Hg are considered to have severe hypertension.
4. Factors contributing to hypertension in CKD include:
c. Plasma volume expansion when sodium excretion is impaired at very low levels of GFR (i.e., <5% of normal).
Laboratory Findings in CRF
Hemogram
a. A nonregenerative (normochromic, normocytic; hypoproliferative) anemia is common in CRF but variable in severity. The severity of the anemia is roughly correlated with the severity of the CRF as judged by serum creatinine concentration. Anemia may be masked by dehydration, which causes hemoconcentration. Thus, the hematocrit should be evaluated in conjunction with the serum total protein concentration.
(1) The primary cause of anemia in CRF is an absolute or relative deficit in the production of erythropoietin in diseased kidneys by peritubular interstitial cells so that the uremic patient cannot meet the demand for new red cells necessitated by loss from hemolysis, hemorrhage, and normal turnover. Erythropoietin stimulates the final differentiation of committed erythroid progenitor cells in the bone marrow into mature red blood cells.
(2) The life span of red cells in uremic patients is approximately 50% that of healthy individuals. The decreased life span is thought to be due to a toxic factor (possibly PTH) in uremic plasma that promotes hemolysis.
(4) Increased red blood cell (RBC) 2,3-diphosphoglycerate due to hyperphosphatemia lowers hemoglobin affinity for oxygen and enhances oxygen delivery to tissues. This partially compensates for the anemia but reduces the hypoxic stimulus for erythropoiesis.
(6) Some dogs and cats with CRF have microcytosis and iron deficiency based on evaluation of serum iron concentrations.
c. Normal platelet numbers but platelet function may be abnormal.
(1) The qualitative platelet function defect (platelet numbers are normal) is most important, and risk of hemorrhage is best correlated with the buccal mucosal bleeding time (normally <2-3 min). Other coagulation tests (e.g., one stage prothrombin time [OSPT], activated partial thromboplastin time [APTT], activated coagulation time [ACT]) usually are normal.
(2) Abnormalities of platelet function include abnormal platelet aggregation, abnormal platelet factor 3 release, abnormal platelet adhesiveness, decreased clot retraction, and decreased thromboxane production by platelets.
Routine Serum Biochemistry
Blood Urea Nitrogen (BUN) and Serum Creatinine Concentrations
(1) By definition, to be in renal failure, BUN and serum creatinine concentrations are increased above normal. Usually, both are increased simultaneously.
(a) Early in the course of CRF in some cats, the serum creatinine concentration may be increased while the BUN concentration remains normal. Anorexia or poor appetite may contribute to the lower BUN concentration in this situation.
(b) In animals with substantial muscle wasting, serum creatinine concentration may not reflect the severity of CRF.
Sodium
(1) Serum sodium is normal in most patients with compensated CRF. Hypernatremia is observed in some patients with decompensated CRF due to dehydration or alternatively due to sodium retention with advanced loss of nephron mass and extremely low GFR (i.e., <5% of normal). Hyponatremia occurs sporadically in CRF if water retention has occurred (e.g., administration of hypotonic fluids and impaired renal excretion of an acute water load).
(a) To maintain balance for sodium as renal disease progresses, the kidneys must reduce the fraction of filtered sodium that is reabsorbed (and increase the fraction that is excreted). Natriuretic hormones play an important role in this adaptive process. For example, a higher concentration of atrial natriuretic peptide enhances sodium excretion by the kidneys in CRF.
(b) Patients with CRF are able to maintain sodium balance despite very low GFR but they are much less flexible than normal individuals in their response to abrupt changes in sodium intake (i.e., they take longer to excrete an abrupt sodium load). If sodium intake is gradually reduced over several months, patients with CRF can gradually reduce sodium excretion.
Potassium
(1) Most animals with CRF have normal serum potassium concentrations. Hyperkalemia usually does not develop unless the animal becomes oliguric or anuric. Hypokalemia may occur in 10% to 30% of dogs and cats with chronic CRF due to some combination of anorexia, loss of muscle mass, vomiting, and polyuria.
(a) A significant body deficit of potassium can exist without the development of hypokalemia. Serum potassium levels can underestimate total body potassium since most of the body’s potassium lies within the cells. As potassium is lost excessively from the plasma, potassium leaches out of the cells down a concentration gradient masking the initial loss of potassium.
(2) Potassium balance is maintained by an adaptive increase in the fractional excretion of potassium as renal disease advances. Increased secretion of potassium per functional remnant nephron occurs in the distal nephron. Adaptive changes include increases in the activity of Na+-K+ adenosine triphosphatase (ATPase) and in the basolateral surface area of principal cells in the cortical collecting ducts. This adaptive response is facilitated by (but does not require) aldosterone.
(3) Patients with CRF are less flexible in their response to added potassium. They have a reduced ability to tolerate an acute potassium load and may require 1 to 3 days to reestablish potassium balance when the intake of potassium is abruptly increased.
(4) Hypokalemic (kaliopenic) nephropathy has been described as a specific syndrome in clinical cats either as a result of CKD or resulting in CKD.
(b) Hypokalemia can contribute to anorexia, depression, and weakness independent of the effects of CRF. Severe muscle weakness (the “hanging-head” posture, truncal ataxia) is seen in many cats with serum potassium <2.5 mEq/L and in some with <3.0 mEq/L (Figure 5-6).
(c) The fractional excretion of potassium into urine is high despite the low serum potassium. Cats with normokalemic CKD also have high fractional excretion for potassium as part of the adaptive nephron response to maintain potassium balance.
(d) Hypokalemia can be associated with functional and or structural changes in the kidneys.
(ii) Decreased GFR initially can be a result of hemodynamic changes, later due to structural changes.
(iii) Structural lesions of tubulointerstitial inflammation and fibrosis associated with CRF have been reported to develop in cats fed marginally potassium replete diets that are highly acidifying and magnesium restricted.
(iv) Some cats with CRF and hypokalemia will improve their excretory renal function following correction of the hypokalemia. Whether this improvement is due to correction of prerenal factors, intrarenal hemodynamics, or resolution of some intrarenal lesions is not clear.
Phosphorus
(1) In early stages of CKD (i.e., IRIS stage 1 and early stage 2), serum phosphorus concentration often is normal due to the corrective effect of renal secondary hyperparathyroidism.
(2) Hyperphosphatemia develops with advancing CRF when 85% and more of the nephrons become nonfunctional. At this point, the corrective effect of high PTH concentration is overwhelmed.
(3) Serum phosphorus concentration in normal adult dogs and cats should be <5.5 mg/dL. Many laboratories report a higher upper end of the normal range for serum phosphorus concentration, presumably because young animals with active bone growth have been included in the population used to determine the normal reference range.
Calcium
(1) Serum total calcium concentrations are normal in the majority of CRF patients but can be low or high in some patients.
(2) Serum total, ionized, protein-bound, and complexed calcium concentrations vary considerably in dogs and cats with CRF. Differences in total calcium concentration tend to be attributable primarily to changes in the complexed calcium component. The complexed calcium fraction can vary from 6% to 39% of serum total calcium in dogs with CRF.
(3) Serum total calcium concentrations are an unreliable predictor of ionized calcium status in CRF. Measurement of serum ionized calcium concentration is the method of choice for accurate assessment of calcium status in dogs with CRF.
(a) Dogs. In a recent study, 22% of 490 CRF dogs were classified as hypercalcemic and 19% were classified as hypocalcemic on the basis of serum total calcium concentrations. Using serum ionized calcium concentrations, however, 9% of the CRF dogs were classified as hypercalcemic and 36% were classified as hypocalcemic. Serum total calcium concentration incorrectly predicted serum ionized calcium concentration in 36% of dogs with CRF. Use of an adjustment formula based on serum albumin or total protein concentration only increased the amount of misdiagnosis.
(b) Cats. In 102 cats with CRF, 20% were classified as hypercalcemic, and 11% were classified as hypocalcemic based on serum total calcium concentration. Using serum ionized calcium concentrations, 29% were classified as hypercalcemic, and 10% were classified as hypocalcemic. Serum total calcium concentration incorrectly predicted serum ionized calcium concentration in 32% of cats with CRF.
(4) Hypocalcemia.
(a) Dogs. The percentage of hypocalcemic CRF dogs is underestimated when using serum total calcium concentration to evaluate calcium status. Based on serum ionized calcium concentration, between 30% and 40% of CRF dogs can be expected to be hypocalcemic.
(b) Between 8% and 15% of cats with CRF have been reported to be hypocalcemic based on serum or plasma total calcium concentration determinations. In a study of 102 cats with CRF, 10% were found to have ionized hypocalcemia.
(c) The Mass Law Effect decreases serum calcium as a consequence of increased serum phosphorus concentration. The amounts of calcium and phosphorus that can remain in solution together are defined by the [Ca(mg/dL)] × [Pi(mg/dL)] product. When this value is greater than 60 to 70, soft tissue mineralization may occur. Decreased production of calcitriol by the diseased kidneys also results in impaired intestinal absorption of calcium.
(d) Vitamin D3 activation (conversion of 25-hydroxycholecalciferol to 1,25-dihydroxycholecalciferol) occurs primarily within mitochondria of the proximal tubules. This activation becomes limited with advanced loss of nephron mass and phosphorus retention.
(e) Hypocalcemia in CRF usually is asymptomatic (i.e., tetany is not observed) because metabolic acidosis leads to an increase in the ionized component of total serum calcium. This occurs because of a decrease in net negative charge on plasma proteins that occurs during acidosis which reduces protein binding of calcium.
(5) Hypercalcemia.
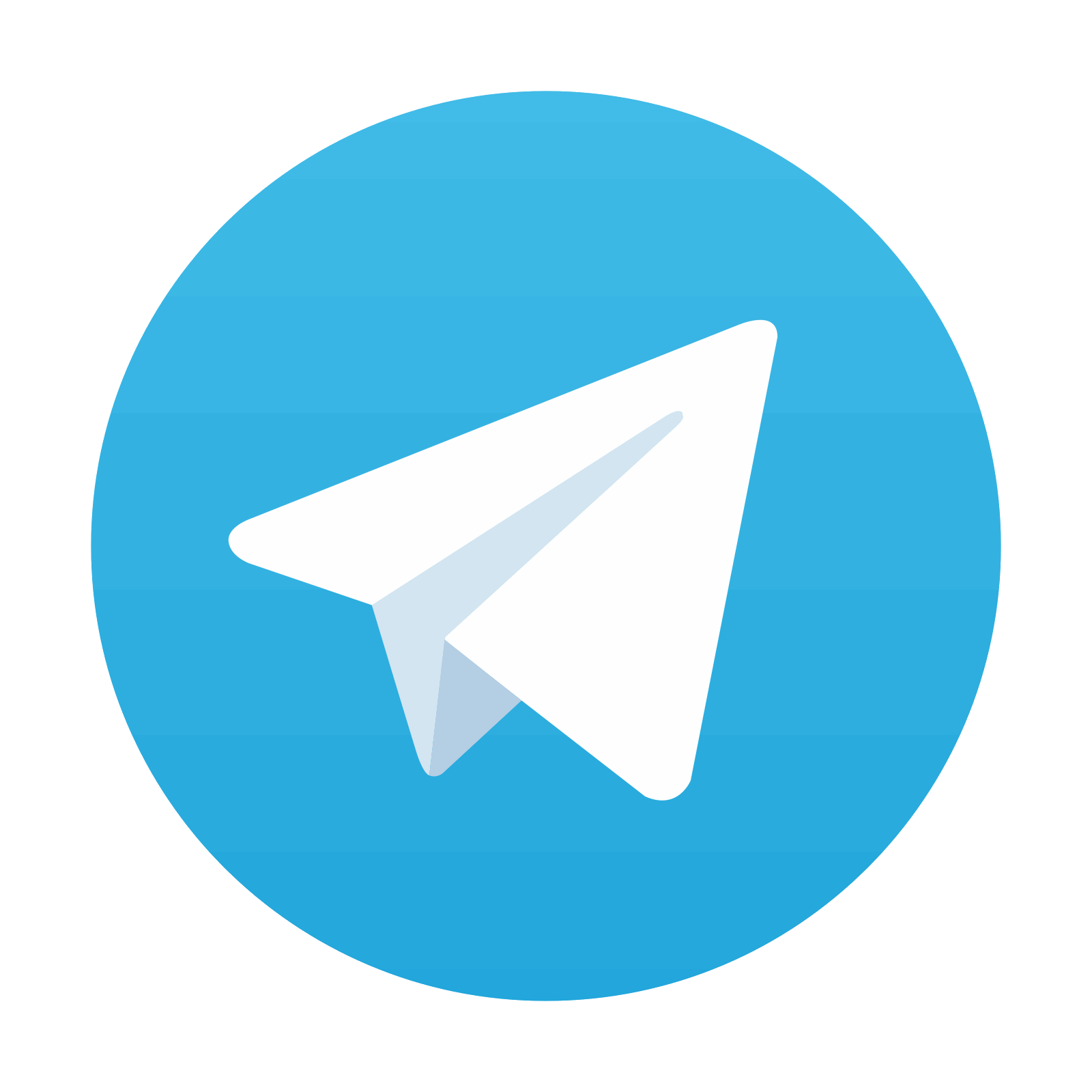
(a) Dogs. In 490 dogs with CRF, 22% were classified as hypercalcemic based on serum total calcium concentration. When ionized calcium concentration was evaluated, only 9% were hypercalcemic. Hypercalcemia was greatly overestimated in dogs with CRF when serum total calcium was measured.
(b) Cats. In 102 cats with CRF, 20% were classified as hypercalcemic based on serum total calcium concentration. When ionized calcium concentration was evaluated, 29% were hypercalcemic. Hypercalcemia was underestimated in cats with CRF when serum total calcium was measured.
< div class='tao-gold-member'>
< div class='tao-gold-member'>
Only gold members can continue reading. Log In or Register a > to continue
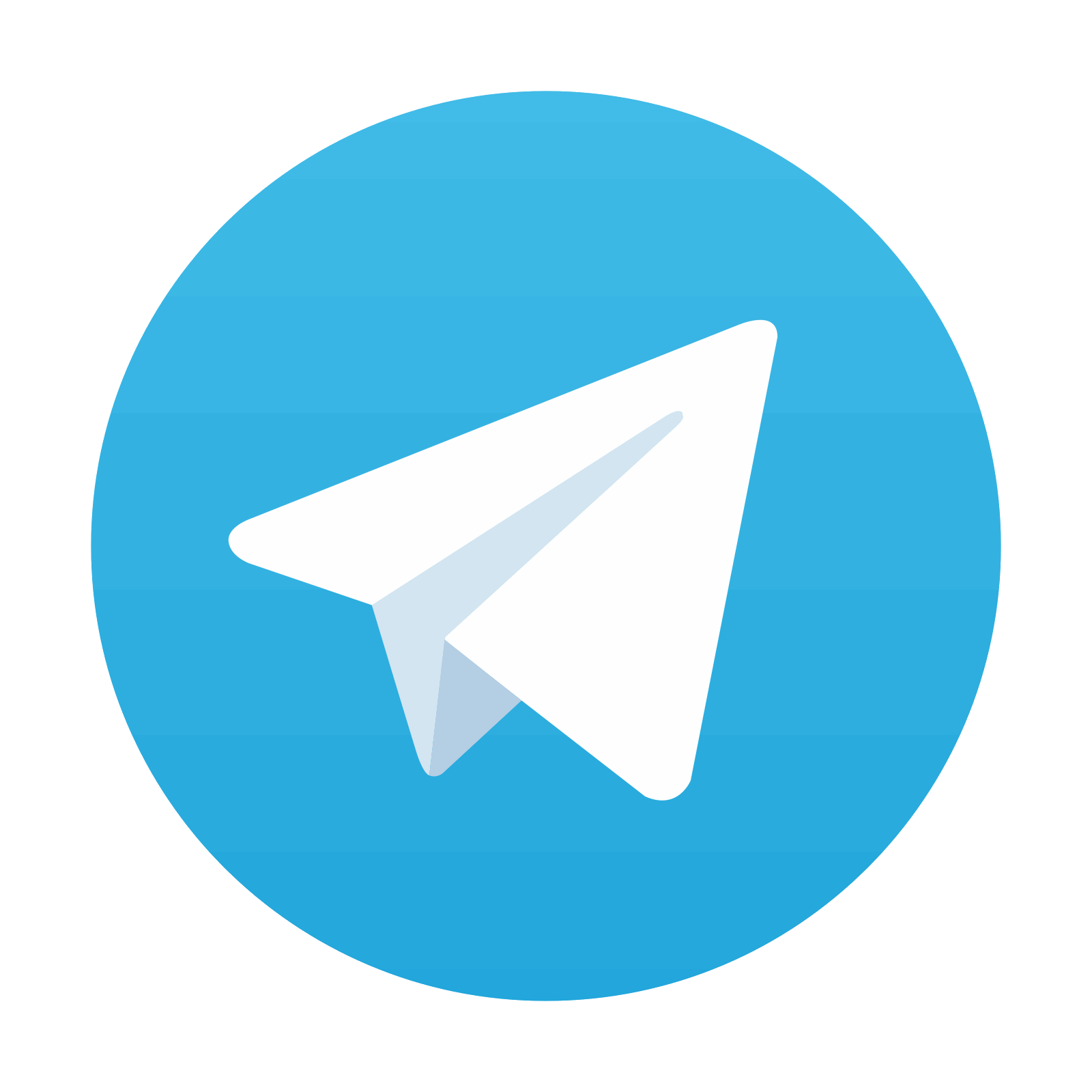
Stay updated, free articles. Join our Telegram channel

Full access? Get Clinical Tree
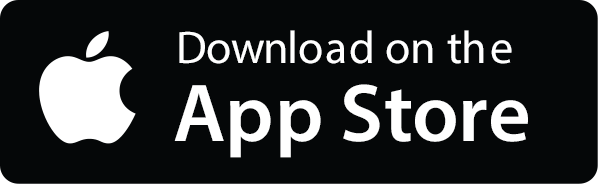
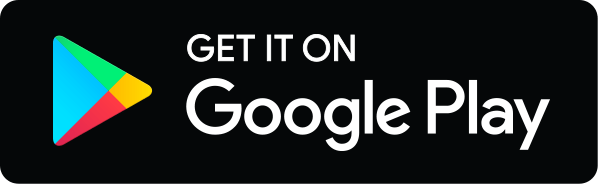
