Chapter 33 Chemotherapy
Drugs used in chemotherapy cause their anticancer effects by interacting with important substrates or enzymes that are related to DNA synthesis or function. Therefore most anticancer drugs are ineffective against cells that are not actively proliferating. Tumors with a high mitotic index (e.g., lymphoma) are much more likely to be sensitive to chemotherapy than those in which mitotic activity is low. Because chemotherapeutic drugs are effective principally on cells that are actively replicating, it is important to have an understanding of the phases of the cell cycle before discussion of individual drugs (Figure 33-1). The part of the cell cycle in which active mitosis occurs has been termed the M phase; it is quite short in all cells, generally lasting less than 1 hour. The period during which DNA synthesis occurs for chromosome doubling in preparation for mitosis is called the S phase and ranges from 8 to 30 hours. When scientists began to learn about cell division, they realized that there were other phases in the cell cycle; because they initially did not understand what was occurring in the cell at these times, the phases were called G (G1 and G2) for gap. G1 follows mitosis, and protein synthesis and RNA transcription occur during this phase. G1 is extremely variable in length depending on the cell type, ranging from 7 to 170 hours. G2 precedes the next mitotic event and is usually brief, ranging from 1 to 4 hours. G0 has been used to describe those cells that are not actively cycling. Certain cell types, such as myocytes and neurons, enter G0 and never cycle again. Other cell types, such as hepatocytes, proliferate in young animals and then cease cycling at maturity but are capable of beginning to cycle again if cell replacement is necessary. Fibroblasts become terminally differentiated in connective tissue, but stem cells remain in the tissue. These can become reproductively active again to repopulate the tissue if a wound occurs.
Chemotherapeutic drugs can be classified into three groups on the basis of their activity in the phases of the cell cycle (Box 33-1). Agents that are considered to be lethal to cells in all phases of the cell cycle, with resting cells as sensitive as proliferating cells, are called cycle nonspecific. Examples include nitrogen mustard (the first chemotherapy agent; its activity was discovered in 1919 with the effects of mustard gas on the troops in World War I) and high-dose cyclophosphamide. Agents that are capable of damaging both resting and cycling cells (although cells in cycle are much more sensitive) include conventional-dose cyclophosphamide and doxorubicin. These drugs spare resting cells and are called cycle specific. Agents that are phase specific exert their lethal effects exclusively or primarily during one phase of the cell cycle, usually S or M; resting cells and cells in the other phases of the cell cycle are not killed. Examples include methotrexate, cytosine arabinoside, vincristine, and vinblastine.
Box 33-1 Sites of Action of Chemotherapeutic Drugs
There are three phases in the development of a new chemotherapeutic drug (Box 33-2). Many artificial chemicals and naturally occurring compounds are screened (generally by the National Cancer Institute or by industry) for cytotoxicity, first in cultures of cancer cells and then in mice or rats. If a particular compound looks promising, a phase I trial is conducted, providing an initial pharmacologic evaluation. The appropriate mode of administration is established for the drug, and common side effects are discovered. Patient tolerance of increasing dosage is also determined. Phase I trials are conducted on very small numbers of patients, generally with advanced and ultimately terminal cancers for which no conventional treatment is available, and doses tolerated by these patients may be below the ultimate therapeutic range. If the compound shows no prohibitive toxicity and shows even slight efficacy (a partial response in a few patients), the phase II trial begins. In this phase screening for efficacy of the drug against a variety of tumors is conducted. After the spectrum of activity is determined, dose–response relationships are determined. The phase III trial is then used to determine drugs that work effectively together, and the new combination protocol is ultimately compared with the existing best treatment. Phase IV trials are known as postmarketing surveillance trials. They involve safety surveillance after the necessary authorities permit the drug to be sold. This surveillance may be required by regulatory authorities. The chemotherapy agent may not have been tested for interactions with other drugs, for example. The safety surveillance is designed to detect any rare or long-term adverse effects over a much larger patient population and longer time period than was possible during the phase I through phase III clinical trials. Harmful effects discovered during phase IV trials may result in a drug being removed from the market.
Most cancers in animals and humans are diagnosed only after they are well advanced. In the 1960s, Skipper and coworkers1 used the rodent L1210 leukemia to illustrate this point and determine cell kill kinetics in tumors. The L1210 leukemia is a rapidly growing tumor with a growth fraction of 100% and a doubling time of only 12 hours. At this rate of growth, a billion cells would accumulate in the rodent only 19 days after injection of a single cell. After treatment of the leukemia with chemotherapy, the investigators determined that cytotoxic drugs kill by log kill kinetics—that is, a given dose of an effective drug kills a constant fraction of cells and not a constant number, regardless of the number of cells present. This principle is known as the fractional kill hypothesis. For example, if a certain drug is known to kill 90% of the tumor cells present, it will kill 90% of the cells whether the beginning number is 10 cells or 10 billion cells (Table 33-1). Thus it should be theoretically possible to cure with chemotherapy, with rapid successive administration of chemotherapy drugs. In fact, antineoplastic drugs kill an extremely variable fraction of cells, ranging from a very small fraction to a maximum of 99.99%; for many tumors the fractional kill is disappointingly small. What prevents theoretical “cures” with chemotherapy is (1) the inability to give drugs in rapid succession, because of host toxicity, and (2) the development of a drug-resistant population of tumor cells during the course of treatment.
Table 33-1 Tumor Depopulation Related to Successive Drug Cycles Assuming a 90% Fractional Cell Kill in a Model System
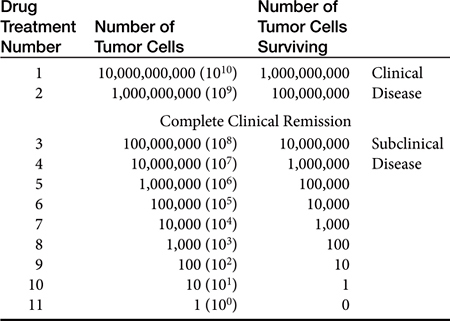
Tumors as small as 1 g (109 tumor cells—a billion) may be detected in the body, especially if they are located in areas such as the skin or mouth. However, it is far more common for tumors to escape detection until they are 10 g (1010 tumor cells) or more. The maximum malignant tumor mass compatible with human life is about 1 kg (1012 tumor cells). If it is assumed that a given tumor originates from a single cell, then a 1-g tumor (109 cells) has gone through 30 doublings from the original cell. To get to 1 kg, only about 10 more doublings will take place.2 It should be clear from these sobering numbers that a large, unresectable tumor burden with only modest sensitivity to chemotherapy cannot be cured or, in many cases, even palliated with conventional chemotherapy administration protocols. The average volume doubling time for various human solid (e.g., nonleukemic) tumors is about 2 months. However, for certain rapidly growing tumors such as embryonal nephroma, seminoma, lymphoma, and leukemia, the volume-doubling time is less than 1 month. For other tumors the volume-doubling time is as long as 1 year. Because chemotherapy affects rapidly dividing cells, tumors with short volume-doubling times are generally chemotherapy sensitive, whereas tumors with long volume-doubling times are generally chemotherapy resistant.
The common cancers in dogs and cats can be broadly divided into three categories:
A regimen of chemotherapy treatment can be divided into several phases. The period of induction is the initial intensive chemotherapy intended to produce remission. Remission is defined as the point at which no measurable tumor mass can be found; for lymphoma remission is declared when the enlarged lymph nodes, liver, and spleen have returned to normal size and malignant cells have disappeared from the peripheral blood and bone marrow. This does not mean that all (or even most) tumor cells have been killed, however, and consolidation therapy with different drugs may be given after apparent clinical remission to produce a larger tumor cell kill. For some tumors such as lymphoma, the animal may be given “pulse” doses of drugs after induction and consolidation to maintain the gains obtained with the induction protocol; this is called the maintenance phase of chemotherapy. Recently, intensification protocols have been described for tumors in which drug resistance is common. These protocols are administered during the maintenance period, when the patient is in apparent remission, and are an attempt to kill developing drug-resistant clones of cells by using one or two new drugs at high doses.
More recently, the use of BSA as a means of calculating doses for all chemotherapeutic agents has been questioned. For many chemotherapy drugs, myelosuppression is the most common toxicity and is dose limiting; it has been found that BSA does not correlate well with either stem cell number in the bone marrow or with resulting hematopoietic toxicity. In fact, correlation is highly significant between bone marrow effects of the cytotoxic drugs and body weight. A phase I study in dogs was performed to evaluate toxicity of doses of intravenous melphalan calculated by BSA. A significantly greater number of small dogs experienced significant toxicity than did large dogs. Another study compared marrow toxicity induced by doxorubicin given at 30 mg/m2 to that induced by the drug given at doses calculated at 1 mg/kg. It was found that a disproportionately greater number of dogs weighing less than 10 kg developed severe myelosuppression at the 30 mg/m2 dose than at the 1 mg/kg dose.3 Limited toxicosis was seen in dogs weighing more than 10 kg with either of the dosing schemes, however. Plasma doxorubicin concentrations were less after treatment at the 1 mg/kg dose in both large and small dogs than in those given 30 mg/m2, and it is possible that 1 mg/kg may be an inappropriately low dose for treatment of animals with cancer. For drugs that may produce severe myelosuppression, measurement of hematopoietic stem cell numbers for each individual patient would clearly provide the most information to prospectively calculate doses for chemotherapeutic agents. Until such a test is available, however, clinicians must use the doses available in the literature, always carefully taking into account the individual animal’s response to the previous drug dose before administering the next treatment. If a doxorubicin dose of 1 mg/kg is well tolerated by a dog or cat weighing less than 10 kg, the next dose may be increased slightly, gradually approaching the dose calculated by BSA; this is called dose escalation. In daily clinical practice veterinarians judge the adequacy of therapy by measuring the response of visible, measurable masses; only much later are they able to evaluate the results of their treatment by survival results. In a rodent model for osteosarcoma, reduction in the dose intensity of melphalan and cyclophosphamide caused a marked decrease in the cure rate long before there was a reduction in the rate of complete clinical remission. On average, it is estimated that a dose reduction of approximately 20% leads to a loss of 50% in the cure rate. A positive relationship between dose intensity and response rate has been demonstrated in many human tumors, including lymphoma and ovarian, colon, and breast cancers. Clinicians should administer the highest dose of a chemotherapeutic drug that can be tolerated by the patient if they are attempting to cure; if palliation is the only goal, a dose that will produce clinical remission without dose escalation may be appropriate, however. Careful patient monitoring for therapeutic response and toxicity is still the best way to titrate the drug dose for each individual patient (Box 33-3).
Box 33-3 Timing of Chemotherapy (Dose–Schedule Relationships)
Common Side Effects of Chemotherapy
Many adverse effects of chemotherapy can be minimized or prevented by careful management, but some animals experience unanticipated side effects that no amount of care or forethought could have prevented. Certain breeds, especially Collies and rarely Australian Shepherds and Shetland Sheepdogs, are carriers of a mutation of the P-glycoprotein multidrug resistance 1 (MDR1) gene. If dogs that are homozygous for this gene are given anthracyclines or vinca alkaloids, the cellular excretion of the drugs is diminished, and they have increased drug exposure and thus increased toxicity. It is estimated that 70% of Collies in the United States are heterozygous for this mutated gene, and 31.2% of Collies are homozygous, with much lower percentages for Australian Shepherds, Shetland Sheepdogs, and other herding dogs. A test for the mutation status of this gene is available through the Veterinary Clinical Pharmacology Laboratory at the College of Veterinary Medicine, University of Washington (http://www.vetmed.wsu.edu/depts-vcpl/). If this test is not performed, dogs of these breeds with cancer should be given conservatively low doses of anthracyclines and vinca alkaloids, or the drugs should not be given at all.
Cells of normal tissue are damaged by chemotherapy; most, given time, will recover. Tissues that are especially affected include those in which the cells have a short life span and require constant renewal (e.g., bone marrow, gastrointestinal mucosa, gonads, hair follicles). Box 33-4 provides a brief overview of the classes of body tissue and, if applicable, typical renewal properties.
Box 33-4 Three Growth Classes of Body Tissue
Myelosuppression
If myelosuppression is severe and life-threatening, recombinant G-CSF or GM-CSF is often administered. These products are human glycoproteins that regulate production of neutrophils within the bone marrow; they are produced in Escherichia coli bacteria. Both stimulate neutrophil progenitor proliferation, differentiation, and functional activity with minimal toxicity. Long-term (i.e., longer than 30 days) use of human G-CSF in the dog or cat results in antibody formation, however, with significant and prolonged decreases in neutrophil counts. At a daily dose of 5 μg/kg subcutaneously, the effects of canine G-CSF on the normal canine bone marrow are rapid and predictable: Mean neutrophil counts in normal dogs increased to 26,330/μL after one injection, with a maximum count of 72,125/μL by day 19 of administration. The neutrophil counts returned to normal in these dogs within 5 days after daily therapy was discontinued.4 Canine G-CSF is not commercially available, but recombinant human G-CSF has resulted in similar elevations of neutrophil counts in the dog. In cats 10 to 14 days of human G-CSF resulted in maximum neutrophil counts ranging from 20,370 to 61,400/μL.5 Thus a short course of G-CSF may be used in dogs or cats either before aggressive chemotherapy, in an attempt to ameliorate or prevent myelosuppression, or as a rescue after chemotherapy has induced significant neutropenia.
Phlebitis and Necrosis
Infusion of the chemotherapy drug should be terminated immediately if the patient shows signs of pain during drug administration or if there is blebbing at the catheter or needle entrance site. If the catheter or needle is still present, the clinician should aspirate any fluid from the extravasated area. A continuing dilemma in the management of extravasation injuries is the absence of evidence-based management strategies. Almost all the recommendations in the literature are (of course) based on anecdotes; even knowing for sure that an extravasation occurred is sometimes difficult, so assessing whether there has been a positive response to a particular treatment is also questionable. However, the current recommendations for vinca alkaloid extravasation are to inject 150 units of hyaluronidase (if available; hyaluronidase has become difficult to obtain) through the patent catheter or needle and then apply local heat for 15 to 30 minutes four times daily for 48 hours. For anthracycline extravasation, the clinician should apply an ice pack for 15 to 30 minutes four times daily and 90% dimethyl sulfoxide (DMSO) topically several times daily for 7 to 14 days; surgical removal should be considered if the area of extravasation is confined. DMSO is a free radical scavenger that causes potent vasodilation and has pain reduction and antiinflammatory mechanisms. Dexrazoxane is a drug that has been used to prevent anthracycline-induced cardiotoxicity. It may also decrease free radical formation, and when given intravenously immediately after extravasation (1000 mg/m2 intravenously, repeated at the same dose the next day), it appears to prevent the tissue necrosis associated with anthracycline extravasation. Growth factors regulate and coordinate wound healing, and they may also be helpful in altering damage from chemotherapy drug extravasation. In animal models both G-CSF and GM-CSF have been shown to be significantly better than saline or no treatment in decreasing the severity and extent of necrosis with doxorubicin extravasations. In one human patient who did not respond to injected dexrazoxane, GM-CSF injected into an ulcerated area of extravasation led to tissue granulation and complete healing within 8 weeks. With any luck, clinicians will never have to use the following protocol: A 1-mL vial of GM-CSF was diluted with saline. Several small injections were then given within the borders of the ulcer. As new granulation tissue formed, GM-CSF injections were given three times weekly for 2 weeks, then twice a week for 2 weeks. Because treatment of extravasation injuries is not yet uniformly effective in preventing the local irritation and necrosis caused by extravasation of these drugs, prevention is the best answer.
Palmar–Plantar Erythrodysesthesia
Palmar–plantar erythrodysesthesia (PPES), also known as hand–foot syndrome in humans, has been seen with constant-rate infusions of doxorubicin, cyclophosphamide, ifosfamide, 5-fluorouracil, and other agents in humans. In dogs PPES has been most commonly associated with the administration of liposome-encapsulated doxorubicin (Doxil).6 PPES is a primarily a dermal toxicity characterized by reddening of the skin. It is followed by edema and eventual ulceration of the skin. PPES tends to occur in areas of friction, such as the weight-bearing portions of the feet and the axillary regions. Histologically, these lesions are described as focal areas of parakeratosis, acantholysis, and chronic active inflammation of the skin and underlying dermis. PPES often resolves quickly after discontinuation of the drug but may recur if the drug is reinstituted. Oral pyroxidine may help ameliorate some of the side effects, although it will not completely prevent PPES.7
Human Health Hazards
Exposure of hospital personnel and owners to carcinogenic, mutagenic, and teratogenic drugs and drug-containing animal waste must be considered. In the days before clinicians took appropriate precautions when mixing and administering chemotherapy, there were many reports in the human literature of fetal loss and birth of infants with congenital defects among nurses and pharmacy staff members who were frequently exposed to chemotherapy drugs.8,9 Proper storage, preparation, and administration of chemotherapeutic agents, as well as proper disposal of cytotoxic drug waste and urine and stool of the animal being treated, should be a concern of every clinician who treats an animal for cancer.8,9 Reviews on proper handling of chemotherapy in the workplace are available; the Occupational Safety and Health Administration (OSHA) publication “Controlling Occupational Exposure to Hazardous Drugs” may be downloaded from OSHA’s website (www.osha.gov/dts/osta/otm/otm_vi/otm_vi_2.html).
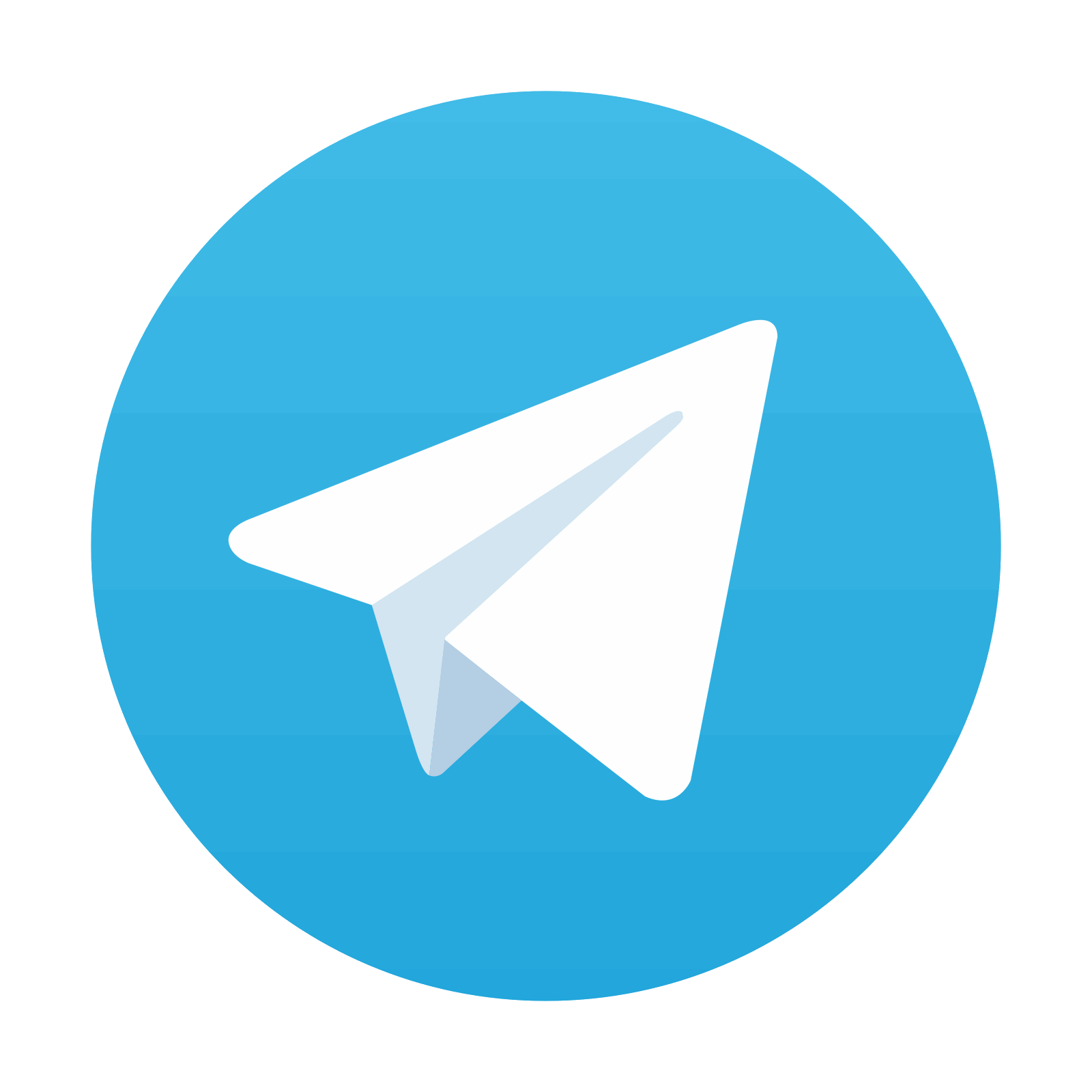
Stay updated, free articles. Join our Telegram channel

Full access? Get Clinical Tree
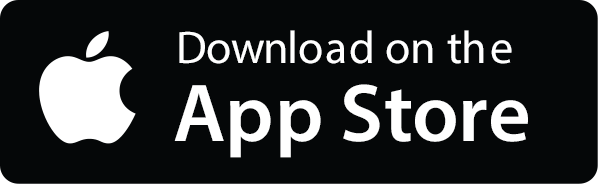
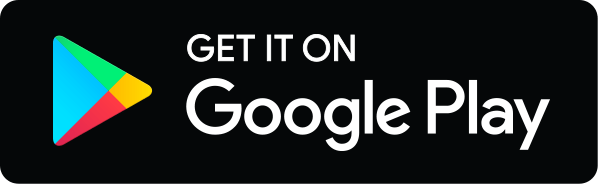