Chapter 13 Cerebellum
DEVELOPMENT
An understanding of the development of the cerebellum is pertinent to the determination of its normal microscopic characteristics and the pathogenesis of the diseases that affect this structure. The cerebellum is the dorsal portion of the metencephalon. The ventral portion is the pons.* The cerebellum develops primarily from the alar plate region of the metencephalon (Fig. 13-1). Its first appearance is a dorsal bulge of the alar plate, which extends the alar plate tissue dorsally and medially in the roof plate, where the growths from each side eventually join each other. The first growth of each alar plate is called the rhombic lip, which arises from the side of the rhomboid fossa of the fourth ventricle. This rhombic lip consists of proliferating cells from the germinal layer adjacent to the fourth ventricle.
The undifferentiated cells in this population of germinal layer cells follow one of two pathways. One group differentiates into primitive neurons or glioblasts that migrate into the substance of the rhombic lip. These immature neurons no longer divide but continue to grow and mature. These neurons give rise to the Purkinje neurons that form a layer throughout the cerebellar cortex and the neuronal cell bodies of the cerebellar nuclei found in the medulla of the cerebellum. The second pathway involves actively dividing germinal layer cells that continue to divide as they migrate to the surface of the rhombic lip, where they form a superficial layer termed the external germinal layer. As the cerebellar folia develop, these germinal cells will remain on the external surface of these folia. They continue to divide, forming an external germinal layer that is 10 to 12 cells in thickness.3 Differentiation occurs along the inner aspect of this thick layer of dividing cells, where these cells stop dividing and become primitive neurons or glioblasts that then migrate internally into the substance of the folium. Most of these cells continue past the Purkinje neurons and form the small granule neurons of the granular layer of the cerebellar cortex. This external germinal layer also contributes the few interneurons (stellate neurons) found in the most superficial layers of the cerebellar cortex, the molecular layer, which primarily consists of granule neuronal axons and dendritic zones of Purkinje neurons. Thus the three layers of the definitive cerebellar cortex are from external to internal, the molecular layer, the Purkinje neuron layer, and the granular (granule neuron) layer. The folding of the developing cerebellar cortex produces the cerebellar folia with the folial white matter lamina in the center of each folium. This folial white matter lamina contains the projecting axons of the Purkinje neurons and a plethora of axons of afferent neurons to the cerebellar cortex from the spinal cord and brainstem. After this neuronal development to populate the cerebellum, the remaining germinal layer cells at this level of the neural tube differentiate into a single layer of ependymal cells that form the lining of the fourth ventricle.
Purkinje neurons are formed by differentiation of rhombic lip germinal cells early in embryonic development. The entire population of Purkinje neurons are differentiated over just a few days.2 They grow and mature as they migrate into the developing cerebellar parenchyma. The total population of Purkinje neurons is well established before the fetus is born. In contrast, the external germinal layer on the surface of the folia continues to divide until late in gestation or after birth in some species that includes dogs and cats. The granule cell neurons complete the population of the granular layer after the formation of the Purkinje neurons and before birth in the horse and farm animal species. The process of neuronal migration and organization in these three layers has been studied extensively and involves the cellular action of radially organized astrocytes and the chemical action of growth factor proteins, one of which is known as brain-derived neurotrophic factor.19 This protein has mitogenic influence over the embryonic germinal layer cells and chemotactic effect on their migration. In addition, the leptomeninges adjacent to the developing folia play a role in the organization of the cerebellar cortex.123 If this layer is disrupted during development, the cortical layers will develop abnormally. In dogs and cats, external germinal layer cell division continues for the first few weeks after birth, and a few granule neurons continue to be formed as late as 10 weeks after birth. The degree of cerebellar development correlates well with the amount of motor function and its degree of coordination that you see in the newborn animal.55 The newborn animals, such as the foal and the species of farm animals that are able to walk with coordination at birth, have a more completely developed cerebellum than the kitten and puppy or the human baby who are essentially helpless at birth. In humans, as the cerebellum develops after birth, their motor function and coordination for ambulation improve. A direct correlation has been shown between the development of the cerebellar cortex and coordinated mobility in the kitten. The term altricial is used for species such as the cat, dog, and human that require a long period of nursing care after birth.
In the calf, the formation of Purkinje neurons is completed by approximately 100 days of gestation. After this differentiation is complete, these Purkinje neurons will grow and mature in conjunction with the continuing development of the cerebellum. In the horse, ox, sheep, goat, llama, and alpaca, the external germinal layer is more active late in gestation and has mostly exhausted its germinal role before birth with the formation of the granule neuronal layer. In the calf, the cerebellar primordium appears at approximately 37 days of gestation. The external germinal layer appears at 57 days of gestation and is maximal in thickness by 183 days, when it is composed of six cell layers. After this phase, it slowly decreases in thickness, reaching a layer that is two cells in thickness by 2 months postnatally and completely disappears by approximately 6 months postnatally. Pathologists should be aware of the presence of this external germinal layer in these young animals and avoid confusing it with meningitis. In the kitten and puppy, the external germinal layer does not reach maximal thickness until the end of the first postnatal week and starts to decrease in size after the second postnatal week. A poorly populated granule neuron layer is present at birth (Fig. 13-4). It grows rapidly in the first few weeks and continues to grow for up to 10 weeks postnatally. External germinal layer cells will persist for as long as 60 to 84 days in the kitten and 75 days in the puppy. When all of the cells have migrated into the molecular and granular layers, only the leptomeninges remain on the surface of the folia adjacent to the molecular layer. Figs. 13-2 through 13-7 illustrate features of this development of the cerebellum.
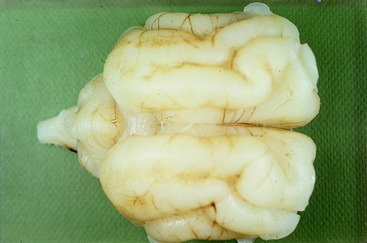
Figure 13-2 Dorsal view of a normal 2-day-old puppy brain. Note the degree of development of the cerebral gyri and the relatively small size of the cerebellum.
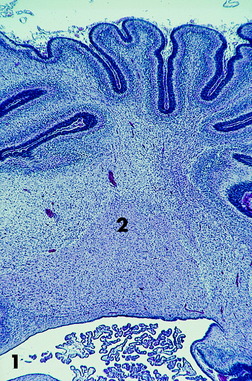
Figure 13-3 Transverse microscopic section of a portion of the cerebellum seen in Fig. 13-2. Note the fourth ventricle and a portion of the choroid plexus (1) at the bottom. Note the large cerebellar nucleus in the cerebellar medulla (2) and the thick external germinal layer covering the developing folia.
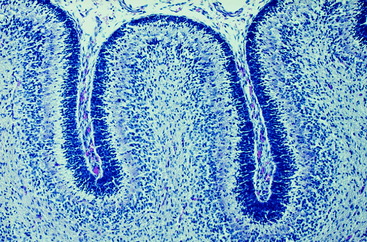
Figure 13-4 Higher magnification of the microscopic section seen in Fig. 13-3, showing the developing cerebellar cortex with the prominent densely populated external germinal layer.
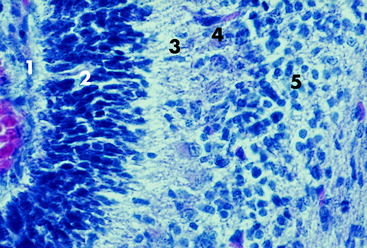
Figure 13-5 Higher magnification of the microscopic section seen in Fig. 13-4. The folial sulcus with meninges and capillaries (1) is at the left adjacent to the thick external germinal layer (2). Note the relatively thin molecular layer (3), the row of small Purkinje neurons (4), and the sparsely populated granular layer (5).
ANATOMY
The cerebellum consists of a central median region, the vermis, named for the wormlike contortions it presents caudally and a hemisphere laterally on each side of the vermis (Figs. 13-8, 13-9). The cerebellum is divided into two disproportionate regions: (1) the large body of the cerebellum and (2) the small flocculonodular lobe. These two regions are separated by the uvulonodular fissure (see Fig. 13-9). The flocculonodular lobe, also known as the archicerebellum or vestibular cerebellum, is confined to the ventral aspect of the cerebellum near its center. The nodulus is the most rostral part of the caudal vermis that is adjacent to the fourth ventricle. It connects laterally by a peduncle on each side to the flocculus, which is a small lobule on the ventral aspect of the cerebellar hemisphere. The much larger body of the cerebellum, consisting of the vermis and two hemispheres, is divided into rostral and caudal lobes by the primary fissure. Within each lobe, the folia are grouped into named lobules that reside in different portions of the vermis and hemispheres.
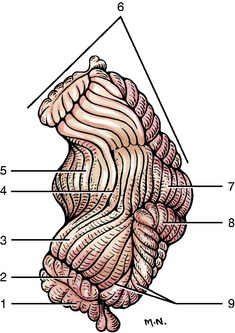
Figure 13-8 Dorsolateral view of the cerebellum. The primary fissure (4) divides the cerebellum into rostral and caudal lobes.
The cerebellum is attached to the brainstem by three groups of neuronal processes on each side of the fourth ventricle (Fig. 13-10). These processes are the cerebellar peduncles (see Fig 9 through 14). Although arranged in a medial to lateral plane, they are named from rostral to caudal based on their connections with the brainstem. The caudal cerebellar peduncle connects the spinal cord and medulla with the cerebellum. It contains primarily afferent processes projecting to the cerebellum. The middle cerebellar peduncle connects the transverse fibers of the pons with the cerebellum, which is entirely afferent to the cerebellum. The rostral cerebellar peduncle connects the cerebellum with the mesencephalon and contains mainly efferent processes passing out of the cerebellum.
When the cerebellum is sectioned transversely or longitudinally, an extensive area of white matter is visible in the center. This area is the cerebellar medulla, which should not be confused with the medulla (oblongata) of the brainstem. The cerebellar medulla has extensions of white matter into the overlying folia. As a group, these extensions appear similar to tree branches and are called the arbor vitae. Individually, each is the white lamina of a folium. The arbor vitae are covered by the three layers of the cerebellar cortex. In the cerebellar medulla are situated collections of neuronal cell bodies that comprise the cerebellar nuclei (see Fig. 2-13). These cell bodies are organized into three nuclei on each side of the median plane. From medial to lateral, these nuclei are the fastigial, interposital, and lateral cerebellar nuclei. For some reason, students have difficulty with this concept of cerebellar nuclei embedded in the white matter of the cerebellar medulla. This arrangement of cerebellar nuclei is directly comparable to the cerebrum, where the neuronal cell bodies are located either on the surface in the cerebral cortex or deep to the surface in basal nuclei. Their arrangement in both the cerebellum and cerebrum is determined by the degree and pattern of migration of the cells from the germinal layer.
The cerebellar cortex, which is composed of three layers, forms the outer portion of each folium and is similar throughout the cerebellum (Fig. 13-11). The folial and sulcal surfaces in the adult are covered by the leptomeninges. Adjacent to these leptomeninges is the most external of the three cortical layers, the relatively cell-free molecular layer. It is composed mostly of the axons and telodendria of granule neurons and the dendritic zones of the Purkinje neurons and a small population of interneurons and astrocytes. The molecular layer covers the middle layer that is a narrow single layer of large flask-shaped neurons, which comprise the Purkinje neuron layer. The deepest of the three layers is the granule neuron (granular) layer. This layer is thick and is composed of a remarkably large number of small neuronal cell bodies and their dendritic zones. This cell layer varies from 5 to 6 neurons thick where the cortex is continuous from one folium to another at the depth of a sulcus to 15 to 20 neurons thick at the top of a folium. Figs. 13-12 through 13-19 illustrate gross and microscopic features of the cerebellum.
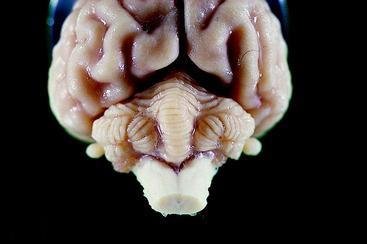
Figure 13-13 Dorsocaudal view of normal dog cerebellum. Note the prominent vermis and relatively small hemispheres when compared with some other animals like the bird, bear, sea lion, and primates in Figs. 13-21 through 13-31.
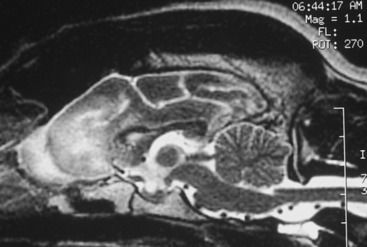
Figure 13-15 T2-weighted magnetic resonance image of the median plane of a normal dog brain. Compare this image with Fig. 13-14.
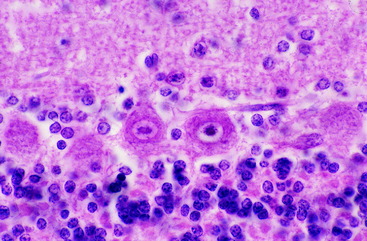
Figure 13-19 Higher magnification of the microscopic section seen in Fig. 13-18, showing the single layer of robust Purkinje neurons below the molecular layer and above the granular layer.
The cerebellar cortex is uniquely organized for the distribution of afferent information (see Fig. 13-11). Two major types of afferents to the cerebellum exist based on the morphology of their telodendrons: mossy fibers and climbing fibers. The more abundant mossy fibers have a widespread origin in the brainstem and spinal cord. As they pass into the cerebellum, collaterals of these axonal processes synapse on the cell bodies and dendritic zones of neurons in the cerebellar nuclei. The main axon continues through the cerebellar medulla into the white lamina of a folium and enters the granular layer, where it terminates on the dendritic zones of the granule neurons. These synapses are sometimes called glomeruli. They are adjacent to the small neuronal cell body of the granule neuron. The axon of this granule neuron projects externally through the Purkinje neuronal layer into the molecular layer, where this axon forms two branches that course in opposite directions parallel to the longitudinal axis of the folium. The dendritic zone of the Purkinje neuron is arranged in the molecular layer as a maze of branched axons that are oriented in a flat plane transverse to the axis of the folium. By this arrangement in the molecular layer, the axon of the granule neuron traverses the dendritic zone of numerous Purkinje neurons. Synapse occurs between these processes. This network can be likened to telephone wires (granule neuronal axons) coursing from one telephone pole (dendritic zone of Purkinje neurons) to another. Climbing fibers are the axons of olivary neurons that enter the cerebellum through the caudal cerebellar peduncle.34 Collaterals of these axons synapse on neurons in the cerebellar nuclei. The main axon continues through the cerebellar medulla into a white lamina of a folium and passes through the granule neuronal layer and the Purkinje neuronal layer into the molecular layer, where it entwines around the dendritic zone of the Purkinje neuron and terminates there in synapses.
The mossy and climbing fibers are facilitory at their synapse with neurons of the cerebellar nuclei and the granule and Purkinje neurons, respectively.58 Acetylcholine is the neurotransmitter released at the synapses of the mossy fibers and aspartate at the synapses of the climbing fibers. The granule neurons are facilitory to the Purkinje neurons with glutamate released at the synapses. Within the molecular layer are stellate neurons (outer and basket) that are inhibitory to the Purkinje neurons. Large stellate neurons (Golgi) are scattered through the granular layer. These neurons are inhibitory to granule neurons.56 The only axon that projects from the cerebellar cortex (an efferent axon) is that of the Purkinje neuron. These axons pass through the granular layer into the folial white matter lamina and continue into the cerebellar medulla. The majority of these terminate on the dendritic zones of the neurons in the cerebellar nuclei. A small population of Purkinje neuronal axons, the cell bodies of which are primarily located in the flocculonodular lobe, leave the cerebellum through the caudal cerebellar peduncle and terminate on the dendritic zones of neurons in the vestibular nuclei. At all of the telodendria of these Purkinje neurons, the inhibitory neurotransmitter gamma-aminobutyric acid is released.29 With the exception of these direct cerebellovestibular Purkinje neuronal projections, the efferent axons that project from the cerebellum to the brainstem are all from the cerebellar nuclei.54 This anatomy supports a major role of the cerebellar cortex in modulating the continual facilitation of the neurons in the cerebellar nuclei via Purkinje neuronal inhibition.
Cerebellar Afferents
Upper Motor Neuron
The projection of UMN information to the cerebellum is diffuse and complex. Brainstem nuclei involved in this cerebellar projection include the red, pontine, and olivary nuclei and the reticular formation. Many of these nuclei receive projections from the telencephalic basal nuclei and the areas of the cerebral cortex involved with motor function. The red nucleus is the source of rubrocerebellar axons that enter the cerebellum through the rostral cerebellar peduncle. Reticulocerebellar axons enter through the caudal cerebellar peduncle. Many of the extrapyramidal system nuclei of the telencephalon and brainstem project to the cerebellum via the olivary nuclei. The olivary nuclei are located in the ventrolateral portion of the caudal medulla (see Figs. 2-14 and 2-15). They extend rostrally to just caudal to the facial nucleus and caudally to a level just caudal to the obex. The olivary nuclei consist of three components on each side, all of which vary in size throughout the length of the nuclei. Where they are most developed, they have the appearance of three fingers oriented obliquely from dorsomedial to ventrolateral just dorsal to the pyramid and medial lemniscus. The hypoglossal axons course along their lateral border. The axons of the neurons in the olivary nuclei cross the midline and join the contralateral caudal cerebellar peduncle. These axons are the major source of the climbing fibers that enter the cerebellum. The olivary neurons are activated by both the neurons of the UMN system and the spinal cord afferents. The pontine nucleus serves as a major relay nucleus for projection axons from all areas of the cerebral cortex to the cerebellum. This cerebropontocerebellar pathway serves for many functions in addition to the UMN system. The axons of the projection neurons in the cerebral cortex enter the corona radiata of the gyrus where the cortical neurons are located. They continue through the centrum semiovale into the internal capsule, crus cerebri, and longitudinal fibers of the pons. The axons in the cerebropontocerebellar pathway leave the longitudinal fibers of the pons to synapse on ipsilateral pontine neuronal cell bodies. The neuronal cell bodies of the pontine nucleus surround the longitudinal fibers of the pons as the latter courses caudally dorsal to the transverse fibers of the pons (see Figs. 2-9 and 2-10). The axons of the neuronal cell bodies of the pontine nucleus cross the midline where they form the transverse fibers of the pons. They continue into the cerebellum via the contralateral middle cerebellar peduncle. This peduncle projects axons primarily to the folia in the cerebellar hemisphere. A direct relationship exists between the evolution of skilled motor function and the degree of development of the cerebral motor cortex, the pons, and the cerebellar hemisphere. In animals such as the human, who have highly skilled motor activity of the digits, the transverse fibers of the pons are so numerous that they extend caudally and cover the trapezoid body, and the vermis of the cerebellum is partly buried beneath the expanded cerebellar hemispheres (see Figs. 13-23, 13-27, 13-31).
Cerebellar Efferents
Cerebellar Nuclei
When the axons in the rostral cerebellar peduncle enter the caudal mesencephalon, most of these cerebellar efferents cross in the ventral tegmental decussation (see Fig. 2-8), occurring at the level of the caudal colliculi caudal to the rubrospinal decussation. These axons cross to terminate in the contralateral red nucleus, ventral lateral thalamic nucleus, or the pallidum. These collections of nuclei participate in a feedback circuit to the cerebral cortex. The most direct pathway is from the neurons in the ventral lateral thalamic nucleus, the axons of which enter the internal capsule via the thalamocortical fibers. They continue through the centrum semiovale into a corona radiata to terminate in an area of the cerebral cortex. A circuitry occurs between the cerebral cortex and the cerebellar cortex that provides immediate feedback from the cerebellum to the cerebrum at the moment when activity is generated in the cerebral cortex. The following example illustrates the structures that participate in this circuitry (Fig. 13-20): Motor cortex of the frontoparietal lobe, corona radiata, centrum semiovale, internal capsule, crus cerebri, longitudinal fibers of the pons, pontine nucleus, CROSS in the transverse fibers of the pons, middle cerebellar peduncle, cerebellar medulla, folial white lamina, granular layer neurons, Purkinje neurons, folial white lamina, cerebellar medulla, lateral cerebellar nucleus, rostral cerebellar peduncle, CROSS in ventral tegmental decussation, ventral lateral nucleus of thalamus, thalamocortical fibers, internal capsule, centrum semiovale, corona radiata, and motor cortex of frontoparietal lobe. This model reflects the intimate relationship between the cerebellum and cerebrum and the important role the cerebellum plays in many functions of the cerebrum.
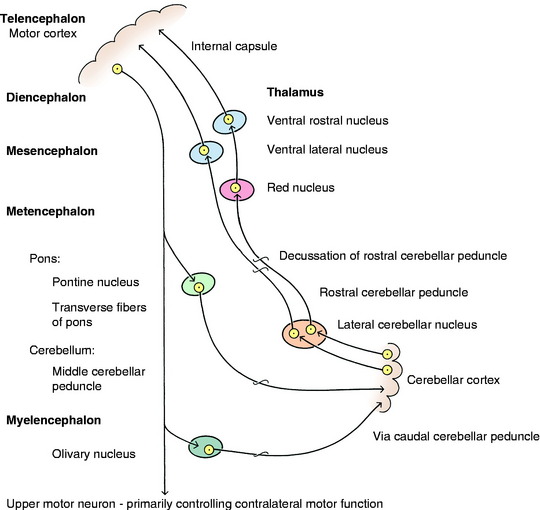
Figure 13-20 Role of the cerebellum in control of motor function: feedback circuit to cerebral cortex from the cerebellum.
FUNCTION
More neurons are found in the cerebellum than in all the remaining areas of the brain combined, and the arrangement of these cells allows for circuitry that makes the action of the cerebellum extremely rapid. Thus the cerebellum has been likened to a computer, with this anatomy being the hardware used for information processing. We tend to limit our understanding of the function of the cerebellum to its role in motor activity because aberrations of this structure are what we see with cerebellar disorders. However, the cerebellum has connections to many regions of the brain, including those involved in sensory systems, cognition, language, and emotions.95 Consider the immense circuitry between the cerebellum and the cerebrum. The information processing that occurs in the cerebellum allows the cerebellum to be involved in both motor dexterity and mental dexterity. We are just beginning to appreciate the diffuse role the cerebellum plays in various brain functions.
In an attempt to correlate structure and function, the cerebellum has been divided topographically in different ways.27,28 On a phylogenetic basis, the cerebellum can be divided into three regions. The archicerebellum includes primarily the flocculonodular lobe, which is concerned with vestibular system activity. The paleocerebellum includes primarily the vermis of the rostral lobe and adjacent hemisphere and is mostly concerned with spinal cord function and postural tonus. The neocerebellum includes the vermis of the caudal lobe and most of the cerebellar hemispheres and is more concerned with regulation of skilled movements.
The cerebellum can also be divided into three longitudinal zones of cortex and related nuclei. The medial zone includes the vermis and fastigial nuclei and is concerned with regulating tone for posture and locomotion and equilibrium of the entire body. The intermediate zone includes the paravermal cortex and interposital nuclei and is more concerned with adjusting motor tone and posture to regulate skilled movements. The lateral zone includes the lateral portion of each hemisphere and the lateral nuclei. This zone primarily functions in regulating skilled movements of the limbs. This portion is more highly developed in primates in which skilled limb and hand movements are more common. A similar remarkable development of the cerebellar hemispheres is seen in a few species of exotic animals. Figs. 13-21 through 13-31 show some of the variations in gross morphology of the cerebellum that we have observed in animals.
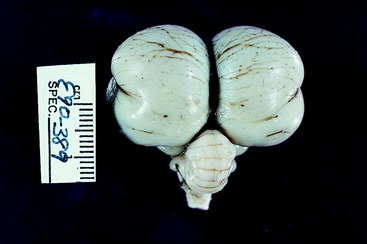
Figure 13-21 Figs. 13-21 through 13-31 show some of the variations in the gross morphology of the cerebellum in a few species of animals. Fig. 13-21 is the brain of a great horned owl, which is representative of most avian species where the cerebellum consists only of a vermis. Note the normal absence of any cerebral gyri.
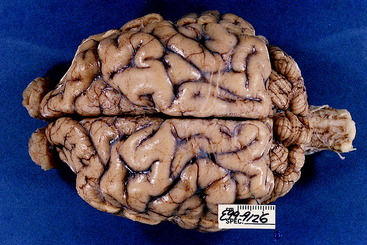
Figure 13-22 This is a dorsal view of the brain of an American brown bear. Note the marked width of the cerebellar hemispheres.
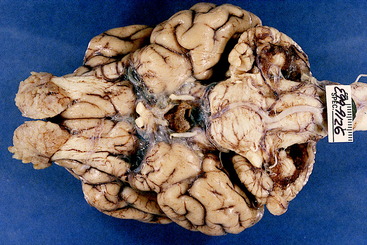
Figure 13-23 Ventral view of the brain of the American brown bear. Note the lateral extent of the cerebellar hemispheres and the associated large area composed of the transverse fibers of the pons that cover the trapezoid body.
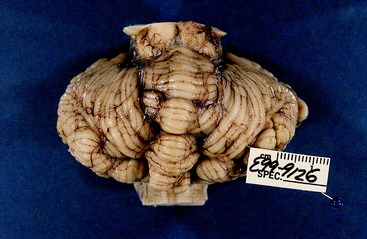
Figure 13-24 Dorsal view of the entire cerebellum dissected from the brainstem of the American brown bear.
The rostral portion of the cerebellum is also concerned with the inhibition of LMNs to the antigravity extensor muscles of the neck and thoracic limbs. It thus participates with the UMN in the support of the body against gravity.
CLINICAL SIGNS OF CEREBELLAR DISEASE
The resting posture of the patient may show a broad-based stance with the thoracic limbs and a truncal ataxia, which is a swaying of the body from side to side, forward and backward, or occasionally dorsoventrally. These truncal swaying motions are sometimes called a titubation. These motions may appear as gross jerky movements of the entire body. A fine head and neck tremor is characteristic of a cerebellar disorder and is usually augmented by the initiation of voluntary movements, such as when the head is reaching for food or a toy. This tremor is described as an intention tremor, or a form of dysmetria involving the head and neck. Lesions limited to the cerebellum will not cause a diffuse, whole-body tremor, as described in Chapter 8. In severe cerebellar disorders, the patient may lie in lateral recumbency, unable to right itself to stand and with its head and neck extended in a position of opisthotonus.
The rostral lobe of the cerebellum is especially inhibitory to the stretch reflex mechanism of antigravity muscles (extensor muscle tone).116 Lesions in this area may result in opisthotonus with rigidly extended thoracic limbs. In some instances, the pelvic limbs may be flexed forward, ventral to the trunk, by hypertonia of the hypaxial muscles that flex the hips. The combination of extended neck and thoracic limbs with flexed hips is called a decerebellate posture. If the rostral lobe lesion involves the ventral lobules, the pelvic limbs may be extended away from the trunk, similar to the thoracic limbs as in a decerebrate posture.
With unilateral lesions, the clinical signs of cerebellar ataxia are ipsilateral with spasticity, hypermetria, and abnormal postural reactions. The disturbance to the vestibular system components of the cerebellum may cause a balance loss and head tilt toward or away from the side of the lesion. Remember what we described in Chapter 12 as the paradoxical central vestibular syndrome that occurs with unilateral cerebellar medullary lesions involving the caudal cerebellar peduncle or flocculonodular lobe of the cerebellum. With these lesions, the clinical signs of vestibular system dysfunction, such as the head tilt, will be contralateral to the lesion. The abnormal nystagmus is usually positional and has no consistency to its direction, which can change with different positions of the head.
Occasionally with unilateral lesions of the fastigial or interposital nuclei, an anisocoria with a dilated pupil may be observed that is slowly responsive to light. The third eyelid may protrude, and the palpebral fissure may be enlarged. The pupillary changes usually occur in the eye that is ipsilateral to an interposital nuclear lesion and contralateral to a fastigial nuclear lesion.71
Animals with significant cerebellar disease often fail to respond to the menace test used to evaluate the visual system. In the presence of normal vision and facial muscle function, these animals with cerebellar disease fail to close their eyelids when threatened by a menacing gesture. Varying degrees of this deficit have been observed in all species of domestic animals with diffuse cerebellar lesions, especially deficits that involve the interposital and lateral cerebellar nuclei. The fact that the entire central visual pathway from the retina to the visual cortex, as well as the facial neurons, must be intact for the normal menace response to take place is known. However, the unknown factor is whether the pathway between the visual cortex and the facial nucleus is direct via projection axons from the visual cortex through the internal capsule, crus cerebri, longitudinal fibers of the pons, and corticonuclear fibers to the facial nucleus. Alternatively, the visual cortex may project rostrally to the motor cortex, and the latter projects to the facial nucleus, as just described. Also unknown is whether the rostral colliculus plays any role in this pathway. Where does the cerebellum fit into this scheme? We believe two possibilities exist. Either (1) the pathway responsible for the menace response goes through the cerebellum, or (2) input occurs from the cerebellum to the pathway. In the former instance, the cerebrocortical projection pathway must pass through the cerebellum via the cerebropontocerebellar route, with the cerebellum projecting to the facial nuclei. In the latter instance, the loss of cerebellar activity that normally projects to the cerebral cortex interferes with this menace response at the level of the cerebral cortex, and therefore a pathway through the cerebellum is unnecessary. We have seen unilateral cerebellar lesions in the dog and horse that resulted in a lack of this menace response on the same side as the lesion. If you study Fig. 14-14, you can see why this ipsilateral relationship would be expected. Note that crossing of axons occurs at both the optic chiasm and again at the pons.
CEREBELLAR DISEASES
Malformation—Abiotrophy
Dogs
Clinical signs of a diffuse cerebellar disorder that is first observed when the puppy tries to stand and walk and is not progressive is most likely the result of a developmental abnormality. These abnormalities are uncommon and have no proven cause in the dog.* No recognized common in utero viral infection in dogs exists that affects primarily the developing cerebellum. If a puppy survives the systemic effects of an infection with the canine herpesvirus in the first week of life, it may be left with a cerebellar ataxia from the effect of the virus on the developing cerebellum.110 This event is rare. One report has been published of a possible exposure to the canine parvovirus associated with a cerebellar malformation.118 Cerebellar cortical abiotrophy is common in dogs and affects many breeds, which causes a progressive clinical disorder that usually starts at a few weeks of age. In a few breeds, the onset is at approximately 1 year of age or later. These disorders are inherited disorders that usually involve an autosomal recessive gene.
Malformation
See Video 13-1. This video shows Hope, a 3.5-month-old male Labrador retriever. He was presumed to be normal until 2 weeks old when, unlike his littermates, he was unable to stand and would flop from one side to the other. When examined at 1 month of age, he was alert and responsive and would struggle to stand to walk but would constantly lose his balance and fall in all directions. By 3 months of age, he was able to stand and walk a short way but with great difficulty before he fell. The owner made an interesting observation that Hope could swim in the nearby lake much better than he could walk. The referring veterinarian noticed an occasional head and neck tremor. Study the video. These clinical signs are typical of a severe cerebellar disorder with significant involvement of the vestibular components of the cerebellum. The age of onset and the mild improvement over the first few weeks correlate well with an in utero developmental defect in the cerebellum. Little improvement occurred by 4 months of age, and Hope was euthanized. At necropsy the only gross and microscopic lesions involved the cerebellum, most of which was absent (Figs. 13-32 through 13-34). No vermis was noted, and only small asymmetric remnants of the most lateral portions of the hemispheres was observed. Only a thin membrane connected the two hemispheric remnants, and this had small clusters of cerebellar parenchyma in it. Although no perinatal viral disease has been proven in dogs to cause an inflammation of the developing cerebellum resulting in cerebellar hypoplasia and atrophy, this lesion in Hope has features similar to that which we see in calves infected in utero with the bovine virus diarrhea virus. Typically in these calves, no evidence was noted after birth of the inflammation that occurred between 100 and 200 days of gestation. A severe in utero compromise of the blood supply from the cerebellar arteries might cause this lesion, but this possibility has not been reported in any domestic animal and would be difficult to prove.
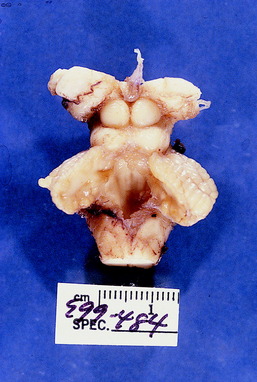
Figure 13-33 Dorsal view of the cerebellum and brainstem of the brain seen in Fig. 13-32 with the cerebral hemispheres removed.
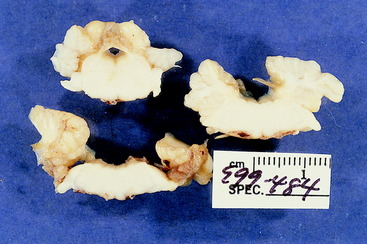
Figure 13-34 Transverse sections of the cerebellum, pons, and medulla of the brain seen in Figs. 13-32 and 13-33. Note the severity and asymmetry of the lesion.
See Video 13-2. This video shows a 4-month-old female miniature schnauzer that was unable to stand when her littermates began to walk. By 2 months of age, she could stand and walk a few feet but then would fall to either side. This sign is evident on the video. Not shown are occasional episodes of opisthotonus and extensor rigidity of the limbs. She also occasionally exhibited a mild neck and head tremor. The presence of these clinical signs of cerebellar dysfunction at the time this puppy should be able to stand and walk and the lack of progression of the clinical signs strongly suggests an in utero developmental abnormality. A computed tomographic (CT) scan showed an area of hypodensity on the midline ventral to the tentorium cerebelli centered where the vermis should be located. This dog was euthanized, and necropsy revealed the absence of the entire cerebellar vermis caudal to the primary fissure. This area included the medullary portion with the fastigial nucleus. Microscopic study showed no inflammation. The symmetry of this malformation suggests a primary genetic developmental abnormality rather than a destructive process that a viral agent would produce. In children, partial or complete absence of the cerebellar vermis is called Dandy-Walker syndrome. This syndrome is sometimes accompanied by agenesis of the corpus callosum and other cerebral malformations. No cause has been determined. Dandy-Walker syndrome has been reported in dogs.94,119
See Video 13-3. This video shows Buddy, a 4-year-old male Samoyed that has exhibited the gait abnormality that you see on this video since he first tried to walk as a puppy. The owners had difficulty training him, but they were devoted to providing him with the best quality of life possible. At 4 years of age, he began to have generalized seizures. Magnetic resonance (MR) imaging was performed as part of his diagnostic study for the seizure disorder. This effort revealed bilateral symmetric lissencephaly and a very small cerebellum. We have observed this same combination of brain malformations in a litter of wire fox terriers and a litter of Irish setters. A similar syndrome occurs in children, which is inherited as an autosomal recessive gene defect. The abnormal gene thought to be involved in neuronal migration is called the RELN gene.50,72,85 This gene codes for the protein reelin, which is involved with neuronal migration during development. Dogs with lissencephaly are typically difficult to train and often develop generalized seizures after one year of age. Buddy’s seizures were partially controlled with anticonvulsants.
See Video 13-4. The two wire fox terriers in this video were from a litter of three puppies. In the first portion of the video, they are approximately 4 to 5 weeks of age and had never been able to stand to walk. One puppy was euthanized, and necropsy showed a severe cerebellar malformation and bilateral lissencephaly (Fig. 13-35). The cerebellar malformation is responsible for their inability to right themselves to stand and walk. The second portion of the video shows the remaining puppy at approximately 7 weeks of age, and the same dog appears in the last portion of the video at approximately 1 year of age. Three years later the clinical signs of cerebellar dysfunction still had not changed. However, this dog developed generalized seizures at 4 years of age, which presumably reflects the dysplasia associated with the lissencephalic malformation. The cerebellar malformation consisted of a symmetric reduction in its overall size to approximately one third of normal with small blunt folia. These rudimentary folia had no normal cortical organization. Purkinje neurons were haphazardly scattered through clusters of granule neurons. We have observed a similar combination of lissencephaly and cerebellar hypoplasia and dysplasia in a litter of Irish setters (Figs. 13-36 through 13-39).
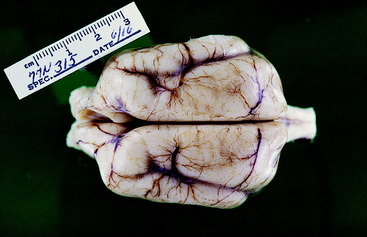
Figure 13-37 Dorsal view of the brain of the dog in Fig. 13-36. Note the extent of the lissencephaly and the lack of any visible cerebellum.
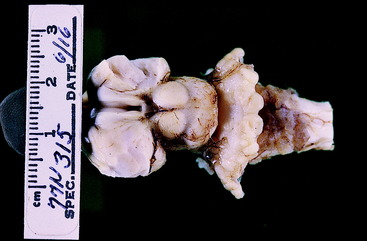
Figure 13-38 Brainstem and hypoplastic cerebellum from the dog in Figs. 13-36 and 13-37 after removal of the cerebral hemispheres. Note the symmetry of the cerebellar malformation, which would not be expected if an in utero viral infection was the cause.
Abiotrophy
Cerebellar cortical abiotrophy is usually a postnatal syndrome that occurs in many breeds of dogs.* The affected animal is normal at birth and develops a slowly progressive cerebellar ataxia at a variable time after birth. Most often, this development starts at a few weeks of age after a brief period of normal activity. In a few breeds, the onset of recognizable clinical signs may not occur for a year or more. The rate of progression varies between the breeds that are affected with this disorder. In some breeds, progression is fairly rapid and results in the inability to coordinate to stand. In others, the gait disorder is very mild and does not incapacitate the animal. As a rule, the primary lesion is an intrinsic degeneration of the Purkinje neurons. The term abiotrophy describes this form of degeneration. It refers to a cell that dies prematurely as a result of some intrinsic genetically determined abnormality within the cell’s metabolic system. By definition, abiotrophy (a-bio-trophy) means a lack of (a-) a vital biological substance (bio) necessary for maintenance of that cell (-trophy).65 Neurons should last for the normal life span of the animal. Any degeneration before this is premature, and if it is caused by some intrinsic defect in that neuron, the degeneration is abiotrophic. The degree of degeneration seen at necropsy depends on how long the degeneration has been progressing. In acute cases, you may see an ischemic type of degeneration in the Purkinje neurons. In chronic cases, no Purkinje neurons may be present, and in their place may be an accumulation of astrocytes that are sometimes called Bergman astrocytes. In most animals, the granular layer neurons will also be depleted. This status is thought to be a retrograde form of degeneration because these neurons no longer have any dendritic zones for their synapses once the Purkinje neurons have degenerated. In these chronic cases, astrogliosis will also be present in the cerebellar nuclei that is secondary to the loss of the telodendria of the Purkinje neurons that terminate here. Most breeds have no lesions elsewhere in the brain. The Kerry blue terrier and Chinese crested breeds are exceptions to this rule because they also have degenerative lesions in extrapyramidal nuclei.42,46,102,103 A cerebellar cortical abiotrophy was first described in Kerry blue terriers in 1976. Since then, a plethora of descriptions have been published of cerebellar cortical abiotrophy in a large number of canine breeds. Where enough data are available, an autosomal recessive form of inheritance has usually been established as the most common cause. Once the anatomic diagnosis of a cerebellar disorder is made, the differential diagnosis for a progressive disorder is abiotrophy, inflammation, cystic malformation, and neoplasia. These patients usually have an onset of clinical signs at an age that is uncommon for neoplasia. Cerebellar medulloblastoma (primitive neurectodermal tumor) is a rare neoplasm seen in young dogs and cattle.125 Epidermoid or dermoid cysts are uncommon, but the most common site for these cysts to occur is in the caudal cranial fossa, where the cerebellum can be affected by their progressive enlargement as a result of their secretory activity.96 Inflammation is common at this young age when many of the abiotrophies occur, but its limitation to the cerebellum is unexpected. However, an exception to this rule exists. Neosporosis in young adult dogs has a predilection for the cerebellum.77 The reason for this predilection is not known, but the slowly progressive clinical signs of a cerebellar disorder that occurs is very similar to those caused by abiotrophy. See Case Example 13-1 that follows in the section on other cerebellar diseases. MR imaging is necessary to help rule out these other diagnoses. The MR images will be normal in the early stages of abiotrophy. In the late stages of chronic abiotrophy, atrophy of the folia is present. The T2-weighted median plane sagittal image is the best image to show an increase in the size of the sulcal spaces between the atrophied folia (Fig. 13-40). Molecular studies are in progress to identify the genomic basis for these inherited abiotrophies, which is necessary for identifying the carrier animals and eliminating them from the breeding programs.
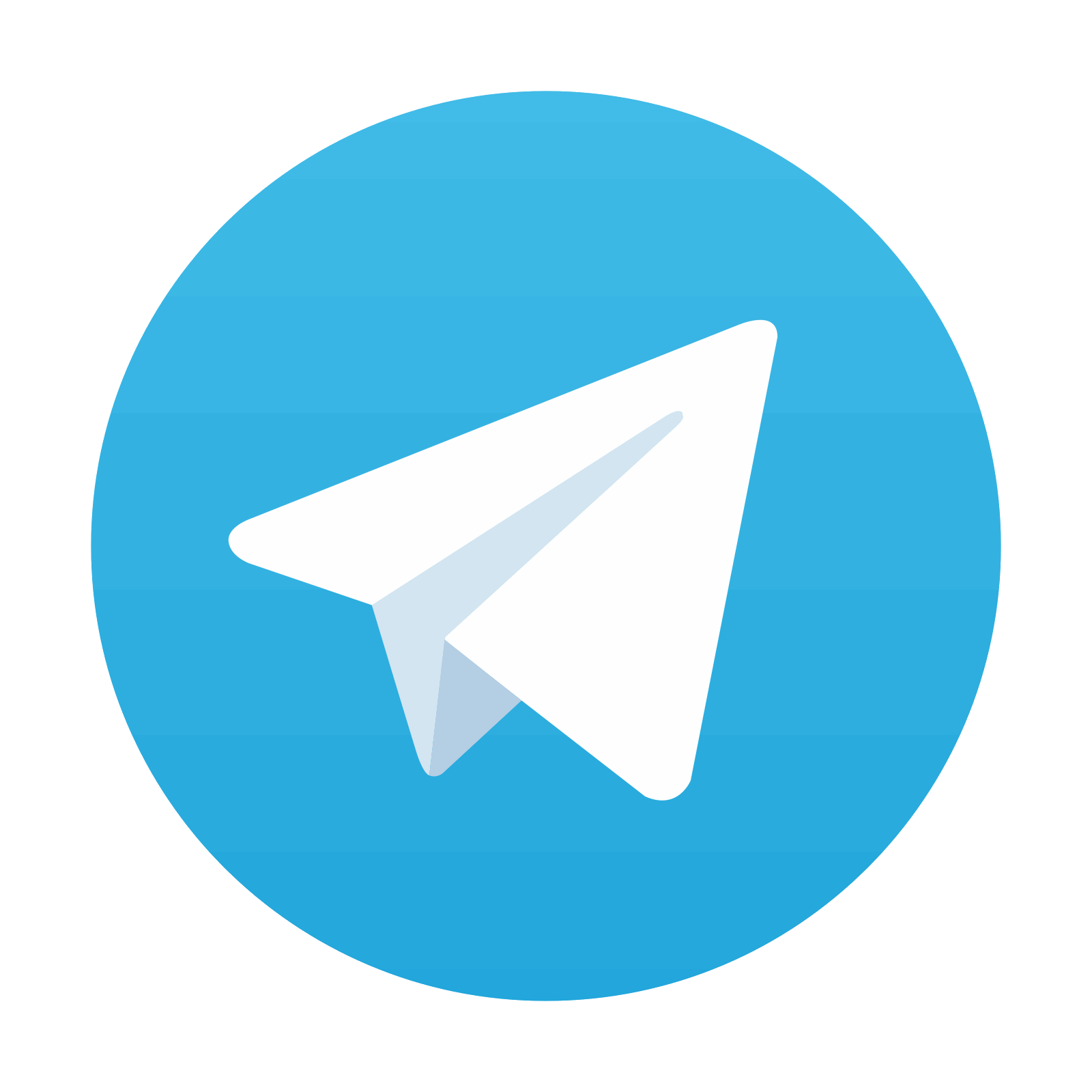
Stay updated, free articles. Join our Telegram channel

Full access? Get Clinical Tree
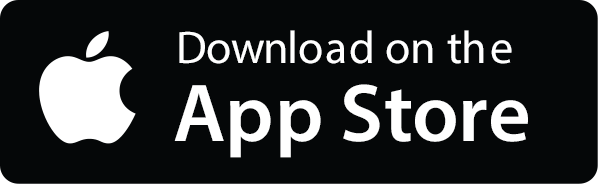
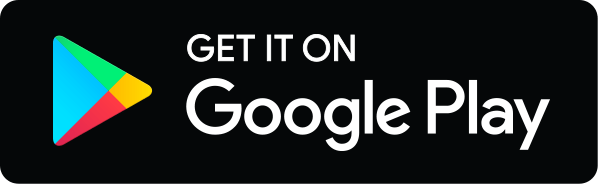
