CHAPTER 12 Sean G. Sanders The cerebellum is unique both structurally and functionally within the central nervous system (CNS). Although only occupying approximately 10% of brain parenchyma in dogs and cats, it is primarily responsible for the efficient and accurate processing of motor function. The cerebellum is also recognized as having a multifunctional role in several integrative functions, including behavior processing, memory, associative learning functions, and nociceptive processing (pain). A basic understanding of the cerebellum’s role in the nervous system is helpful when considering how pathology within the cerebellum affects clinical functions. The cerebellum is responsible for the modification, rather than the initiation, of movement. The cerebellum functions to coordinate segmental movements so they are fluid in nature and to aid in ensuring movements are performed in an efficient manner. The cerebellum helps to regulate posture, unconscious proprioception (the position of the body in space), and muscle tone. The cerebellum is also believed to influence conscious thought processes, such as judging the timing of events and solving spatial and perceptual reasoning problems. The cerebellum can be conceptually divided in numerous ways, according to developmental, anatomical, and functional features. Depending upon the clinical situation and clinician preference, an understanding of the cerebellum can be framed in the context of these different categorization schemes. Phylogenetically, the cerebellum can be divided into three parts. The archicerebellum is the oldest part and is composed of the flocculonodular lobe. The paleocerebellum is next in line and consists of the most rostral portion of the cerebellum, rostral to the primary fissure. Finally, the neocerebellum is the newest and largest portion of the cerebellum and is composed of the hemispheres and paravermal portion caudal to the primary fissure. Anatomically, the structure of the cerebellum contains the two lateral hemispheres, a median portion (the vermis), and a small ventral portion (the flocculonodular lobe). The superficial surface folds of the cerebellum are known as folia (Fig. 12.1). On the cut section, the cerebellum has many branching, infolded sections known as arbor vitae (meaning “tree of life”). Embedded within the deep white matter are the three paired “roof” nuclei. From medial to lateral, these are known as the fastigial, interposital, and dentate nuclei (Fig. 12.2). The dentate nucleus is also referred to as the lateral nucleus in some texts. These nuclei are synaptic centers for both afferent and efferent information. The cerebellum makes up the dorsal half of the metencephalon, with the pons comprising the ventral half. The cerebellum is attached to the brain stem by the three paired cerebellar peduncles, which act as conduits for both afferent and efferent information related to cerebellar function. Figure 12.1 Gross anatomy of the cerebellum, ventral aspect. (The Ohio State University. Reproduced with permission.) Figure 12.2 Cut section of cerebellum. Deep cerebellar nuclei are from medial to lateral fastigial, interposital, and dentate. Facial nucleus at level of cerebellomedullary junction. (The Ohio State University. Reproduced with permission.) Finally, and probably most importantly, the cerebellum can be functionally divided into the cerebrocerebellum, spinocerebellum, and vestibulocerebellum. Each functional division is related to its associated anatomical structures (Fig. 12.3). The cerebrocerebellum consists of the cerebellar hemispheres, is associated with the dentate nucleus, and is primarily responsible for limb movements. This portion of the cerebellum is necessary for the coordinated implementation of voluntary, planned, multijoint movements. Additionally, the cerebrocerebellum is involved in the cognitive process of the intention to move. Studies have shown that neurons of the dentate nucleus fire before the onset of movement and before the neurons in the cerebral motor cortex responsible for that particular movement. In general, the cerebrocerebellum is required for the control of movement direction, timing, and force. It accomplishes this function through influencing the cerebral cortex motor output. Figure 12.3 Functional divisions of the cerebellum. (The Ohio State University. Reproduced with permission.) The spinocerebellum is composed of the vermis and the intermediate zone (medial portions of the hemispheres). It receives somatosensory information from the spinal cord. This division of the cerebellum is associated with the fastigial and interposital nuclei. It is primarily responsible for regulating muscle tone and unconscious motor movements necessary for posture and gait. The vestibulocerebellum is made up of the flocculonodular lobe (the nodulus and two flocculi) and the fastigial nuclei. This portion of the cerebellum primarily projects to the vestibular nuclei of the brain stem. It is principally responsible for the maintenance of equilibrium and coordinating movements of the head and eyes. The inner portion of the cerebellum is the medullary substance. It contains the deep cerebellar nuclei (i.e. fastigial, interposital, dentate nuclei). The outer portion is the cerebellar cortex. The cerebellar cortex is made up of three distinct layers. From outer to inner, these are the molecular cell layer, the Purkinje cell layer, and the granular layer (Fig. 12.4). The sophisticated arrangement of the cells that make up these layers allows the cerebellum to distinguish between errors in movement and the intended movement. The complex interactions of the distinct synapses allow the cerebellum to recognize both temporal and spatial events. Figure 12.4 Cellular arrangement of the cerebellar cortex. (The Ohio State University. Reproduced with permission.) The molecular layer is a comparatively cell-free area. It contains two distinct cell types and the axons of neurons from the granular layer that send their projections to the molecular layer (parallel fibers). The cells are known as basket cells and stellate cells. Both cell types are inhibitory. The axons of basket cells descend to the Purkinje cell layer and make terminal arborizations with Purkinje cell bodies. The stellate cells make synaptic contacts with Purkinje cell dendrites that extend into the molecular layer. The Purkinje cell layer contains the highly ordered and uniformly arranged large cell bodies of the Purkinje cells. This layer, located between the molecular layer and the granular layer, contains the cells responsible for the output of the cerebellum. Purkinje cells are large, highly metabolically active, and therefore very susceptible to ischemic or toxic damage. These inhibitory neurons utilize gamma-aminobutyric acid (GABA) as their neurotransmitter. The myelinated axons of Purkinje cells leave the cerebellar cortex to synapse on the deep cerebellar nuclei. Along the way, these axons will send collateral projections off in the granular layer to synapse on neurons located there. Additionally, the Purkinje cells will send fanlike dendritic processes into the molecular layer. These projections run perpendicular to the parallel fibers in the molecular layer. The parallel fibers run through the dendritic processes of the Purkinje cells much like electrical wires running through a bushy tree, making cross synapses with the Purkinje dendrites as they travel throughout the molecular layer. The deepest layer of the cerebellar cortex is the granular layer. Two types of neurons are present there: the granule cells and the Golgi cells. This layer is filled with densely packed cells that look like lymphocytes when Nissl stained. The granule cells have unmyelinated axons that ascend to the molecular layer where they function as parallel fibers making cross synapses with the Purkinje dendrites (the electrical wires through the bushy trees). Golgi cells are located in the upper portion of the granular layer. They are inhibitory neurons that utilize GABA as their neurotransmitter. Dendrites from the Golgi cells extend throughout all layers of the cerebellar cortex. Their axons form specialized synapses at the cerebellar glomeruli. The glomeruli are chiefly made up of axonal endings of mossy fibers (one of the two types of afferent projections into the cerebellum). Dendrites and axons from the Golgi cells, as well as dendrites from the granule cells, will synapse in the cerebellar glomeruli. The cerebellum receives sensory information from the entire nervous system yet projects its regulatory information to specific areas of the brain and spinal cord. The ascending and descending spinal cord tract related to cerebellar function are summarized in Fig. 12.5. The three different functional subdivisions of the cerebellum each receive primary information from specific portions of the nervous system (Fig. 12.3). The main contributions to each subdivision will be discussed; however, it is important to realize that afferent information to the cerebellum is distributed to all subdivisions in varying degrees. The cerebrocerebellum (hemispheres) receives input from the cerebral cortex via the pons (corticopontine fibers). Axons of the corticopontine fibers originate from the cerebral cortex, synapse on the deep pontine nuclei, cross midline as the transverse fibers of the pons, and ascend through the middle cerebellar peduncle. This information assists the cerebrocerebellum with motor planning. Figure 12.5 Transverse section through the spinal cord (second spinal cord segment) showing the descending and ascending spinal tracts related to the cerebellum. (The Ohio State University. Reproduced with permission.) The vestibulocerebellum (flocculonodular lobe) primarily receives afferent projections from the vestibular labyrinth indirectly via the vestibular nuclei in the medulla and directly via connections from the vestibulocochlear nerve (cranial nerve [CN] VIII). It also receives information from the lateral geniculate nuclei and rostral colliculi via the pontine nuclei (corticopontine tracts). All information entering the cerebellum from the pontine nuclei ascends through the middle cerebellar peduncle. This information will help the cerebellum with balance (both while standing and ambulating) and eye movements. The spinocerebellum (vermis and intermediate zone) receives afferent information regarding joint position and lower motor neuron (LMN) status from the spinal cord (spino-olivary tracts, spinocerebellar tracts, cuneocerebellar tracts). The neural pathways’ names are a combination of their place of origination in the nervous system and their place of termination. For example, the spinocerebellar tracts originate in the spinal cord and terminate in the cerebellum; therefore, they are termed spinocerebellar. In general, the spinocerebellar tracts convey information from the limbs and body to the ipsilateral cerebellum. Proprioceptive information from the lower body and pelvic limbs is conveyed to the cerebellum via the dorsal and ventral spinocerebellar tracts (Fig. 12.6), whereas such information from the cervical region and thoracic limbs is conveyed to the cerebellum primarily via the cuneocerebellar (spinocuneocerebellar) and rostral (cranial) spinocerebellar tracts (Fig. 12.7). The dorsal spinocerebellar tract sends information from cutaneous, muscle, and joint receptors regarding proprioception from the pelvic limbs and caudal region of the body. This tract is located in the dorsal portion of the lateral funiculus. The information is projected to the ipsilateral cerebellum and enters via the caudal cerebellar peduncle. The ventral spinocerebellar tract, which also conveys information from the pelvic limbs and caudal body region, is also located in the lateral funiculus, ventral to the dorsal spinocerebellar tract. It sends information from muscle receptors and Golgi tendon organs, which ultimately reaches the ipsilateral cerebellum (after crossing midline twice) via the rostral cerebellar peduncle. The spinocuneocerebellar tract (cuneocerebellar tract), located in the lateral portion of the dorsal funiculus (fasciculus cuneatus), primarily sends proprioceptive information from cutaneous, muscle, and joint receptors from the ipsilateral thoracic limb and cranial part of the body. It projects to the cerebellum via the caudal cerebellar peduncle. It is named by its synaptic connections with the lateral cuneate nucleus in the medulla (rostrolateral to the medial cuneate nucleus), where spinal projections synapse prior to entering the cerebellum. The rostral spinocerebellar tract (medial to the ventral spinocerebellar tract) also conveys information from the thoracic limbs and cranial aspect of the body. Axons of this tract enter the ipsilateral rostral and caudal cerebellar peduncles. The cervicospinocerebellar tract is a pathway conveying proprioceptive information from the neck region (first four cervical spinal cord segments) to the contralateral caudal cerebellar peduncle. The axons of this tract synapse in the rostral vermis. The cerebellum indirectly (via the pontine nuclei) receives visual and auditory information through the collicular nuclei via the middle cerebellar peduncle and vestibular input from the vestibular nuclei via the caudal cerebellar peduncle. This information is organized somatotopically and represents entire maps of the body projected in the region of the vermis and intermediate zone of the cerebellar hemispheres. Figure 12.6 Schematic illustration of the ascending proprioceptive tracts to the cerebellum from the lower body and pelvic limbs—the dorsal and ventral spinocerebellar tracts. (The Ohio State University. Reproduced with permission.) Figure 12.7 Schematic illustration of the ascending proprioceptive tracts to the cerebellum from the cervical region and thoracic limbs—the cuneocerebellar and rostral spinocerebellar tracts. (The Ohio State University. Reproduced with permission.) All of the projections to the cerebellum are either mossy fibers or climbing fibers (Fig. 12.4). These excitatory fibers alone control the activity and therefore the output of the Purkinje cells. For the most part, afferent axons enter the cerebellum through either the caudal or middle cerebellar peduncles. In general, information from the pons (metencephalon) enters the cerebellum through the middle cerebellar peduncle and information from the medulla (myelencephalon) enters through the caudal cerebellar peduncle. Exceptions include the ascending proprioceptive information from the pelvic limbs coursing in the ventral spinocerebellar tract and a portion of the ascending information from the thoracic limbs conveyed by the rostral spinocerebellar tract. These axons enter the cerebellum through the rostral cerebellar peduncle. Mossy fibers primarily originate from the spinal cord, reticular formation, vestibular nuclei, and pontine nuclei. They indirectly influence the firing of Purkinje neurons through their interactions with granule cells in the granular layer. The granule cells are excitatory interneurons. The interaction of mossy fibers and granule cells occurs at the cerebellar glomeruli, where mossy fiber terminals come into contact with Golgi cell axons and granule cell dendrites. A single mossy fiber will typically activate a cluster of granule cells, the axons of which will ascend into the molecular layer. Along the way, the granule cell axons will send off collaterals to Purkinje cells. Once in the molecule layer, these granule cell axons will become parallel fibers where they then again have the opportunity to interact at cross synapses with the Purkinje dendrites that extend into the molecular layer. Three additional fiber types innervate all areas of the cerebellar cortex. All of these fibers enter the cerebellum as mossy fibers. One of the fiber types originates from the locus ceruleus and releases norepinephrine. These fibers interact directly with Purkinje cells. The second fiber type originates from the raphe nuclei and contains serotonin. These fibers do not synapse on Purkinje cells. Both of these fibers are thought to possibly play a role in emotional states and cerebellar function. A third fiber type originates from undefined brain-stem nuclei and releases acetylcholine. Climbing fibers originate from a single source: the olivary nucleus (rostral myelencephalon). The olive receives descending input from the cerebral cortex and brain-stem upper motor neuron centers, as well as ascending input from the ventral spinocerebellar tract. Information from the olivary nucleus projects to the cerebellum, sending off collaterals to the cerebellar nuclei, and continues into the Purkinje cell layer to form direct excitatory contact with Purkinje neurons. A Purkinje neuron receives input from a single climbing fiber, and a single climbing fiber will interact with several Purkinje neurons. Of all the excitatory synapses in the CNS, the climbing fiber’s interaction with the Purkinje neuron is one of the most powerful. An action potential from a climbing fiber will produce a prolonged depolarization of the target Purkinje cell. While mossy fibers rely on temporal and spatial summation to excite a Purkinje neuron, the climbing fiber can accomplish this with a single action potential. As stated previously, the only output of the cerebellar cortex is via Purkinje axons. The Purkinje neurons exert an inhibitory influence on the tonically active deep cerebellar nuclei. As with the afferent organization, the efferent projections from the cerebellum can be categorized based upon the three major subdivisions (Fig. 12.3). Purkinje neurons from the most lateral subdivision, the cerebrocerebellum, project to the most lateral cerebellar nuclei: the dentate nuclei. From the dentate nucleus, fibers descend through the contralateral rostral cerebellar peduncle to the ventral lateral nucleus of the thalamus. By crossing back to the contralateral rostral peduncle, the cerebrocerebellar projections will project to the ipsilateral cerebral cortex. As described earlier, the corticopontine fibers, which project to the cerebrocerebellum, cross in the pons to project to the contralateral cerebrocerebellum; the efferent projections cross back to maintain an ipsilateral circuit. The thalamic projections influence the motor and premotor areas of the cortex. Additionally, the dentate nucleus sends projections to the red nucleus in the mesencephalon. These projections form a complex feedback circuit back to the cerebellum through the olivary nucleus. They do not make up any portion of the rubrospinal tract (a descending spinal projection that originates from the red nucleus). The efferent projections of the cerebrocerebellum are responsible for the coordination and planning of limb movements. Some of the vestibulocerebellum’s Purkinje neurons project directly to the vestibular nuclei in the medulla. These arise primarily from the flocculonodular lobe. Neurons of the fastigial nucleus also project to the vestibular nuclei. The vestibular nuclei give rise to the lateral and medial vestibulospinal tracts, which run in the ventral funiculi of the spinal cord white matter (Fig. 12.5). The main output is necessary for the control of axial and proximal limb muscles in order to maintain balance. Vestibulocerebellar projections are also responsible for coordinated eye and head movements, as well as vestibular reflexes. The spinocerebellum’s output is projected through the fastigial and the interposital nuclei. The fastigial nucleus receives output from the vermis. Some of these fibers ascend to the cerebral cortex; however, most efferents descend to the vestibular nuclei and the reticular formation, both of which contribute fibers to the medial descending motor systems. The interposital nucleus receives output from the intermediate zone (medial hemispheres). Axons of the interposital nuclei continue on to influence lateral descending motor tracts. The areas of the cerebellum contributing to the medial descending motor pathways are responsible for the regulation of axial and proximal musculature. Axons from the interposital nuclei descend through the rostral cerebellar peduncle to the contralateral red nucleus. They continue to the ventral lateral nucleus of the thalamus and eventually the cerebral motor cortex. These projections help to regulate the function of distal limb muscles by exerting their influence on both the corticospinal and the rubrospinal tracts, which are two of the lateral descending motor pathways. Efferents of the spinocerebellum coordinate the actions of axial and limb muscles to smooth out intended movements and dampen oscillations of ongoing movements. The cerebellum has traditionally been thought of as a modulator or regulator of movement initiated by the cerebrum. Experiments have shown that neurons in the dentate nucleus (cerebrocerebellum) actually begin firing before neurons in the cerebral motor cortex that are responsible for the intended movement. Neurons in the fastigial and interposital nuclei (spinocerebellum) fire after the movement has begun, and their activity is directly related to the velocity and force of the movement. This suggests that the cerebrocerebellum is actually involved with the intention to move and therefore linked to cognitive function at some level, whereas the spinocerebellum monitors the consequences of the evolving movement. It is well accepted that the cerebellum plays a much greater role in an animal’s cognitive awareness and output related to its environment. The roles of the cerebrocerebellum and spinocerebellum in the initiation and execution of movement can be hypothetically mapped out (Fig. 12.8). The upper loop involves the cerebrocerebellum, which, along with the premotor cortex, is important for the programming of future movements. The lower loop involves the spinocerebellum, which, along with the cerebral motor cortex, regulates evolving movements. Figure 12.8 Hypothetical map of cerebrocerebellar and spinocerebellar involvement in the planning and execution of movement. The cerebellum is responsible for the optimization of motor performance. To that end, it plays an important role in “learning” the most efficient method for the initiation, execution, and modification of conscious and unconscious movements. The firing of Purkinje neurons is modified indirectly through the action of mossy fibers on climbing fibers so that, in a sense, the climbing fibers are “teaching” the Purkinje neurons to generate a new response. Clinical signs of cerebellar dysfunction can generally be referred to as abnormalities in the rate, range, direction, and force of motor movements. Lesions of the cerebellum typically result in ipsilateral deficits. This physiological phenomenon is due to the fact that output through the rostral cerebellar peduncle is crossed and primarily acts to modify movement generated through the corticospinal and rubrospinal tracts, which are also crossed. Pure cerebellar disease does not result in paresis (weakness). There are usually no conscious proprioceptive deficits with lesions restricted to the cerebellum; however, during a neurologic evaluation, it is important to consider that a specific lesion may involve multiple areas of the nervous system. For example, a cervical lesion may affect the ascending spinocerebellar tracts, leading to signs of cerebellar dysfunction, while at the same time affecting conscious proprioceptive pathways, resulting in apparent proprioceptive deficits (such as articular-process (facet)-associated cervical vertebral spondylomyelopathy in young, large-breed dogs). Disorders of cerebellar function may result in specific alterations in motor function. These deficits may exhibit themselves as hypermetria or hypometria, ataxia, dysmetria, and tremors. It is more common for dogs and cats with cerebellar disease to exhibit signs of hypermetria. This results from the delay in the cessation of the intended movement and typically manifests itself at gait as the animal takes on a “toy soldier” appearance. The limbs are stiff and frequently overreaching. There is usually a broad-based stance, and the intended movement will overshoot the goal with an excessive rebound. Ataxia can be thought of as the manifestation of a constellation of signs resulting from hypermetria, dysmetria, and asynergia. Dysmetria refers to abnormalities in the rate and force of movement. Asynergia is the lack of coordination in the execution of multisegmental movements. Since the cerebellum is usually affected in a diffuse manner, the clinical sign of ataxia is a common one. The word ataxia comes from the Greek and means “without order.” When considered in this manner, it is easy to see how ataxia can be one of the primary manifestations of cerebellar disease. The clinician will recognize the animal having a “drunken” gait or the “lack of an axis.” This may be thought of as movement away from the axis of the body (a straight line drawn from the nose to the tail). The animals will have a broad-based stance and sway from side to side at gait. The head will often oscillate, as the vestibulocerebellar function is also affected. Affected patients tend to have difficulty maintaining their balance and are easily tipped over. Since the spinocerebellum is somatotopically organized, a lesion of the vermis or fastigial nucleus will produce titubation. Titubation is a shaking of the trunk and head while sitting or standing, which is commonly observed as forward and backward oscillations of the body. Tremors of cerebellar origin are referred to as intention tremors. These tremors are manifested when the animal initiates voluntary movement. They typically appear as the to-and-fro movements or oscillations of the head and neck, which become more severe as the animal begins to reach the goal (such as a food bowl). Intention tremors imply a lesion of the cerebrocerebellum, the more lateral areas of the cerebellar hemispheres, or the dentate nucleus. This is the area of the cerebellum primarily influencing the application of voluntary movement. Lesions of the vestibulocerebellum (flocculonodular lobe), vestibular nuclei, or fastigial nucleus may cause nystagmus, strabismus, loss of balance, and a head tilt either toward or away from (paradoxical) the side of the lesion. Lesions of the rostral cerebellar peduncles and deep cerebellar nuclei produce the most severe clinical signs. An abnormality in the region of the fastigial nucleus in cats may produce contralateral pupillary dilation. The affected pupil will not be completely responsive to light (miosis), and there will be partial protrusion of the ipsilateral third eyelid. With lesions of the interposital nucleus, the same pupillary light abnormalities will occur as in a fastigial nucleus lesion; however, the affected pupil is ipsilateral to the lesion. Paradoxical vestibular syndrome and decerebellate rigidity are two classic signs of cerebellar disease. Occasionally, animals will present with a head tilt and paresis on the opposite side of the head tilt, and they may or may not have other signs of cerebellar dysfunction (e.g. nystagmus or tremor). Lesions of the flocculonodular lobe or caudal cerebellar peduncles will produce these signs. In these cases the head tilt is paradoxical in that it is away from the side of the lesion. The lesion can be localized to the same side as the most severe conscious proprioceptive deficits. This paradoxical vestibular syndrome is likely due to an abnormality in the vestibulocerebellum, which contains many crossed and uncrossed pathways and, depending on the location and severity of the lesion, may result in the contralateral head tilt (see Chapter 11). Decerebellate rigidity is seen with severe cerebellar abnormalities. It is characterized by a rigid extension of the forelimbs, alternating flexed or extended hind limbs, and opisthotonus. If damage is confined to the cerebellum, consciousness is maintained. The classic differentiation therefore, between an animal with decerebrate posturing and decerebellate posturing is the animal’s level of consciousness. A decerebrate animal will be stuporous or comatose. Occasionally, animals with cerebellar disease will have absent or decreased menace responses ipsilateral to the lesion. The menace deficit is observed despite normal visual pathways and facial nerve function. The cerebellum is known to play a role in the inhibition of micturition, and diseases of the cerebellum may therefore play a part in disorders of micturition (see Chapter 16). Cerebellar cortical abiotrophy This group of diseases specifically refers to the degeneration of normal neuronal cell populations within the cerebellar cortex after birth. Additionally, deep cerebellar nuclei and the terminal fields of cerebellar projections may be affected. Many of these diseases are genetically transmitted; most are suspected to be autosomal recessive traits. The etiology for this group of disorders is unknown. Lack of a metabolic component necessary for cellular survival may be involved. Some of these abiotrophies may represent inappropriate programmed cell death of cerebellar neurons (apoptosis). A genetically transmitted autoimmune process was suspected in a group of young (8-wk-old) Coton de Tuléar dogs with granule cell degeneration. An apoptotic process was suspected in a separate, younger (2-wk-old) group of Coton de Tuléar dogs with granular cell loss. Purkinje neurons are the most commonly affected cell population in cerebellar abiotrophy cases (Fig. 12.9 and Fig. 12.10). However, granule cells, medullary nuclear cells (e.g. cuneate, gracile, olivary nuclei), and motor neurons in the spinal cord have also been affected. A condition of concurrent cerebellar (Purkinje cell) and hepatocellular degeneration was reported in several litters of Bernese Mountain dog puppies. Cerebellar cortical abiotrophy has been primarily reported in dogs, with only sporadic feline reports. The onset and rate of the progression of clinical signs varies with the breed affected (Table 12.1). Most breeds show an onset of clinical signs when the animals begin to ambulate and slightly later (3–12 wks). The course of the disease can be rapid (several weeks) or slowly progressive (several years). In certain cases, the clinical signs will plateau, and the animal will remain stable. Figure 12.9 Normal section of cerebellum. Note molecular layer (M), Purkinje cell layer (P), and granular layer (G). Hematoxylin and eosin stain. Bar 5, 200 mm. (Kevin Lahmers, Washington State University, Pullman, WA, USA, 2014. Reproduced with permission from Kevin Lahmers.) Figure 12.10 Cerebellar abiotrophy. Notice the lack of Purkinje cells in the Purkinje cell layer (P) and the thinning of the granular cell layer (G). Hematoxylin and eosin stain. Bar 5, 200 mm. (Kevin Lahmers, Washington State University, Pullman, WA, USA, 2014. Reproduced with permission from Kevin Lahmers.) Table 12.1 Breeds of dogs and cats recognized to have cerebellar abiotrophy. Note: Other isolated cases: Akita, Boxer, Brittany Spaniel, Cairn Terrier, Clumber Spaniel, Cocker Spaniel, English Spaniel, Fox Terrier, German Shepherd, Golden Retriever, Great Dane, Italian Hound, Miniature Schnauzer, Papillion, Mixed Breed, Pit Bull Terrier, Portuguese Podengo, and Scottish Terrier. a Chinese Crested dogs expressing the hairless phenotype (canine ectodermal dysplasia [CED] mutation) are affected through an autosomal dominant transmission. Homozygotes for CED are not viable. b There are two forms of abiotrophy reported in this breed; one appears to be progressive and the other nonprogressive. In some breeds, clinical manifestations of cerebellar dysfunction occur near or during adulthood. In the cerebellar abiotrophy of Gordon Setters, clinical signs usually begin at about 6–10 mos of age and progress steadily over 9–18 mos. There is a late-onset cerebellar degeneration in Brittany Spaniels. These dogs are typically affected between 7 and 13 yrs of age. A slowly progressive late–onset form of cerebellar cortical degeneration has been characterized in American Staffordshire Terriers. Sixty-three dogs were examined. Onset of clinical signs began between 18 mos and 9 yrs. An autosomal recessive mode of transmission is most consistent with the inheritance. The slow progression of clinical signs to the development of an inability to walk and falling repeatedly was observed. In one report of eight Brittany Spaniels, the time from onset of cerebellar dysfunction to euthanasia varied from 6 mos to 4 yrs. A late-onset cerebellar abiotrophy disorder has been described in Old English Sheepdogs. These dogs exhibited primarily gait abnormalities (beginning between 6 and 40 mos of age) that were mild and slowly progressive. A hereditary cerebellar degeneration has also been described in Scottish Terriers, characterized histopathologically by a loss of Purkinje cells and an accumulation of polyglucosan bodies primarily in the molecular layer. The onset of clinical signs is similar to that of the Gordon Setter and Old English Sheepdog. Clinical signs are typically observed between 6 and 40 mos and are slowly progressive. This condition may have been present for some time although not recognized due to possible confusion with the hereditary disorders known as Scotty cramp and central axonopathy of Scottish Terriers. The episodic nature of Scotty cramp and the early onset with rapid progression of clinical signs associated with central axonopathy of Scottish Terriers would be differentiating signs between the conditions. An autosomal recessive mode of inheritance has been confirmed. A late-onset (more than 1 yr of age) cerebellar abiotrophy has also been reported in a number of cats, including two feral cats, a Persian cat, a Siamese cat, and a domestic shorthaired cat. The latter cat also exhibited retinal degeneration. Clinical signs of cerebellar abiotrophy represent the cerebellar syndrome and may include ataxia, intention tremors, nystagmus, poor menace responses with normal vision, opisthotonus, behavioral abnormalities, and depression. Many cases of cerebellar degeneration/abiotrophy are also noted to have contralateral or ipsilateral conscious proprioceptive abnormalities that are believed not to occur with pure cerebellar disease. In a described case of slowly progressive disease in a Papillon, clinical signs developed at 5 mos and progressed slowly over 2 yrs. The dog initially presented with a cerebellar syndrome. Clinical signs progressed to generalized paresis by 1.5 yrs and the inability to stand by 2 yrs of age. The dog was observed to have difficulty swallowing and unilateral facial nerve paralysis followed by death due to aspiration pneumonia by 2 yrs and 9 mos of age. Immunohistochemistry of the cerebellum identified activation of the apoptotic pathway via identification of caspase-3 positive cells without inflammation in the granule and Purkinje cell layers. Neurodiagnostics have generally not been beneficial in the antemortem diagnosis of this condition; however, in several breeds there are gross structural abnormalities of the cerebellum (hypoplasia and focal signal changes) that may be detectable with magnetic resonance imaging (MRI; Fig. 12.11). Recently, computer-assisted MR image measurements have been shown to be very sensitive and specific in diagnosing cerebellar abiotrophy in the American Staffordshire Terrier breed. In addition, brain-stem auditory evoked response (BAER) testing (see Chapter 5) has been shown to be a useful diagnostic tool in American Staffordshire Terriers with cerebellar abiotrophy. There are no effective treatments for this group of disorders. Figure 12.11 T2-weighted, midsagittal MR brain images of a normal dog (A) and an American Staffordshire Terrier with abiotrophy (B). Note the increased amount of cerebrospinal fluid within and between the folia and surrounding the cerebellum compared with the control dog’s image. (Olby et al., 2004. Reproduced with permission from Wiley.)151 Neuroaxonal dystrophy This disease has been reported in dogs and cats and is characterized histologically by swellings at the terminal ends of axons referred to as spheroids. In the physiologic form, it is considered a normal part of aging. The primary form is associated with the development of neuroaxonal swelling as the main pathological feature associated with the disease in young animals. This is also the name of a condition secondary to the accumulation of metabolic by-products in storage diseases, where the disease results in a neuroaxonal dystrophy. There is one report of neuroaxonal dystrophy in English Cocker Spaniels associated with primary metabolic vitamin E deficiency. Most cases of neuroaxonal dystrophy, however, are thought to represent primary, inherited disorders of axonal transport mechanisms. Axons in the cerebellum and its related pathways are affected, and cerebellar dysfunction often predominates early in the disease course. It is thought that a defect in axonal transport leads to the accumulation of transportable products in the distal ends of the affected axons. The disease is genetically transmitted in cats and is thought to be hereditary in dogs as well. An autosomal recessive mode of inheritance is suspected. The onset of clinical signs relating to the cerebellar syndrome is typically within the first few months of life in the Chihuahua, Collie, Papillon, Jack Russell Terrier, as well as the Siamese and domestic shorthaired cat. Boxers may be affected between 1 and 7 mos of age. The Rottweiler is affected within 1 to 2 yrs, and the German Shepherd may be affected at around 15 mos. In the case of the Rottweiler, there may be severe degeneration of the dorsal columns of the cervical spinal cord. These dogs often have conscious proprioceptive deficits, as well as cerebellar dysfunction. In a report of four Papillon puppies diagnosed with neuroaxonal dystrophy, histopathological evidence of disease was present in nearly all examined areas of the brain, affecting gray and white matter (cerebrum, cerebellum, pons, caudate, basal ganglia, etc.). Lesions were also present within the gray matter and all funiculi of the cervical, thoracic, and lumbar spinal cord. Peripheral nerves may also be affected but less commonly than the brain and spine. Wallerian degeneration was a prominent finding as well. The presence of LMN signs (such as reduced to absent patella reflexes) may result in a multifocal localization. Therefore, the diffuse distribution of the pathology warrants consideration of this disease in patients where cerebellar signs are not the only clinical presenting complaint. Neuroaxonal dystrophy may progress rapidly or slowly in the primary forms and is generally a slow progression in its physiologic (age-related degeneration) form, ultimately leading to multifocal CNS dysfunction. Early in the course of the disease, MRI has been reported as normal. However, if allowed to progress, MRI findings of diffuse brain atrophy have been reported with subsequent imaging studies. The value of high-field MRI examination has not yet been determined in the earlier stages of the disease. There is no treatment. Cerebellar malformation It is thought that a genetically transmitted condition of cerebellar hypoplasia exists. This condition has been reported in Chow Chows, Boston Terriers, Airedale Terriers, Irish Setters, Wire-haired Fox Terriers, and Bull Terriers. The reported malformations vary from complete absence of the cerebellum or parts of the cerebellum (agenesis), abnormal development of the cerebellum with no differentiation of tissue (aplasia), to abnormal development of the cerebellum with some differentiation of tissue (hypoplasia). The condition is present at birth and likely represents the failure of normal cerebellar development. The condition is not progressive, and clinical signs may improve as the animal matures. Dandy–Walker syndrome Dandy–Walker syndrome is a rare congenital malformation that shares similarities with the human form of the disease. It consists of a triad of congenital anomalies, including cerebellar vermis hypoplasia (Fig. 12.12), a communicating hydrocephalus, and the presence of a fluid-filled cyst (syrinx) within the posterior fossa. In humans, Dandy–Walker syndrome is one of several congenital syndromes typified by cerebellar vermis hypoplasia. It has been reported in several dogs with no breed or sex predilection and in a kitten. The clinical signs are typical of cerebellar disease (ataxia, hypermetria, absent menace, and tremors). Some dogs have also displayed a head tilt and circling. It is unknown whether the vestibular signs are due to the cerebellar disease or manifestations of the other developmental abnormalities. The clinical signs are typically not progressive, therefore surgical intervention may not be rewarding. Affected animals usually present early in life, at around 3 mos of age. There is no treatment. Figure 12.12 Sagittal T2-weighted MR image from a dog with cerebellar vermis hypoplasia, a characteristic feature of Dandy–Walker syndrome. (Dr. Gena Silver, 2014. Reproduced with permission from Dr. Gena Silver.) Foramen magnum-associated malformations Malformation of the caudal aspect of the skull, similar to Chiari type I disorder of people, has been described in small-breed dogs. These “Chiari-like” malformations (CLM), also termed caudal occipital malformation syndrome (see Chapter 7), are best appreciated on sagittal MRIs and usually include rostral displacement of the caudal aspect of the occipital bone, with caudal displacement of the caudoventral aspect of the cerebellum into or through the foramen magnum. Additionally, small-breed dogs may be affected by atlanto-occipital overlapping (AOO), either alone or in conjunction with Chiari-like malformation; AOO can result in cerebellar compression due to displacement of the C1 dorsal arch. In humans, the presence of this basilar invagination in conjunction with other aspects of cranial–cervical junction abnormalities results in treatment modification for the most successful outcome. There may be meningeal fibrosis at the cervicomedullary junction in dogs with these malformations. Overcrowding of the caudal fossa region is believed to lead to syringomyelia, especially in the cervical spinal cord region. Concurrent hydrocephalus may also be present. In the Cavalier King Charles Spaniel there is an association between increased cerebellar volume and syringomyelia. The malformation is estimated to be present in 70% of nonclinical Cavalier King Charles Spaniels by the age of 6 yrs, whereas if evaluated at 1 yr of age, only 25% of Cavalier King Charles Spaniels have MRI evidence of the malformation. Some patients with Chiari-like malformations may display evidence of cerebellovestibular dysfunction. Medical therapy (e.g. low doses of prednisone, gabapentin, diuretics) may be successful in some cases of Chiari-like malformations. Foramen magnum decompression (FMD) is usually successful in people with the disorder, and the author has had some success in dogs treated similarly. The presence of atlanto-occipital overriding and other aspects of the malformation is thought to result in a more guarded prognosis in dogs with this condition. Occipital dysplasia is a controversial anatomical abnormality of the occipital bone. The controversy centers primarily on whether occipital dysplasia simply represents anatomic skull variation or is an actual disease state. This condition also primarily affects small-breed dogs. In cases of occipital dysplasia, the region of the occipital bone comprising the dorsal boundary of the foramen magnum (supraoccipital bone) is malformed and replaced by a membranous band of tissue. This abnormality can vary from a small notch leading to a keyhole-shaped foramen magnum to a large midline defect dorsal to the foramen magnum. Often, the caudal aspect of the cerebellum and the dorsal aspect of the cranial cervical spinal cord–caudal brain stem are exposed. It has been proposed that fluid-filled cavities in the brain stem and cranial cervical spinal cord seen on MR examination of dogs with this condition are secondary to abnormal pulsation of cerebrospinal fluid (CSF) through the foramen magnum. However, concurrent Chiari-like malformation and occipital dysplasia have been documented in dogs. In addition to the possibility that occipital dysplasia, by itself, does not represent a disease entity, it has been suggested that the decompressive effect of this defect may actually delay the development of clinical signs related to CLM in dogs that have both abnormalities present. The author has surgically removed this band of tissue in several dogs and has seen an improvement in clinical signs relating to ataxia and cerebellovestibular disease. Episodic ataxia A single case report of a 4-yr-old, male neutered Bichon Frise dog with presumed episodic cerebellar ataxia has been reported. Clinical signs manifested when the dog was 4 mos old. The episode were described as often being preceded by vomiting and ptyalism and lasting 6–24 hrs up to 2–4 times a week at their most frequent and intense point. During the episodes, the dog would exhibit stiffness in all four limbs, tetraparesis, reluctance to move, a wide-based stance, exaggerated jerky movements, frequent falling, and the development of a head bob. The dog would be mentally appropriate during the episodes. Diagnostic testing including complete blood count, biochemical profile, urinalysis, thyroid profile, bile acids, profile, thoracic radiographs, abdominal ultrasound, MRI, CSF analysis, and urine organic acid profile were considered normal. The dog responded to treatment with 4-aminopyridine, a potassium channel blocker that is used to treat a similar condition in humans. The clinical signs would usually resolve within an hour of taking the medication, and by 24 mos post treatment, the dog was normal with only occasional episodes occurring 2–3 times a year and being associated with vigorous exercise or long car rides. 4-aminopyridine is a voltage-gated, fast potassium channel blocker that has been shown to improve axonal conduction of demyelinated fibers. The cause of episodic ataxia in humans is unknown; however, it is suspected to be a disorder of irregular firing Purkinje cells. 4-aminopyridine has its greatest affinity for the Kv1.5 potassium channel and it is suspected that its mechanism of action is in restoring the normal pacemaking activity of Purkinje cells. It is used with caution in people due to its potential epileptogenic properties. A more specific blocker of the Kv1.5 potassium channel is hypothesized to be a more effective treatment with fewer potential side effects. Spongy degeneration A presumably autosomal recessive disorder affecting purebred male and female Malinois puppies, resulting in spongy degeneration of the cerebellar nuclei, has been described (13 puppies from five different litters). Clinical signs of cerebellar ataxia arise by 4–7 wks and affected multiple pups in five litters. Most dogs presented with varying degrees of cerebellar ataxia, wide-based stance, hypermetria, stumbling, or falling. MRI and computed tomography (CT) examination did not reveal any gross abnormalities of the brain in the patients that were scanned. The primary histopathological abnormality was severe bilaterally symmetrical vacuolization of the neuropil of the cerebellar nuclei and, to a lesser extent, similar vacuolization of the cerebellar granular cell layer and white matter of the cerebellar folia. Some puppies also demonstrated additional vacuoles in the brain-stem nuclei and the white matter of the brain stem and spinal cord. Because all puppies were euthanized by the age of 13 wks or before, it is difficult to say whether the clinical signs would have been progressive or stabilized. Primary brain tumors Numerous primary neoplasms can affect the cerebellum. Typically, adjacent structures such as the brain stem and associated cranial nerves are affected as well. Not all neoplastic processes may be histologically considered invasive or malignant; however, because of the limited space for expansion, they are not considered benign, from a clinical point of view. Meningiomas are the most common brain tumor of dogs and cats. Unlike the meningiomas of humans and cats, dog meningiomas tend to be invasive to surrounding tissue and therefore difficult to remove entirely surgically. They usually form at the cerebellopontine or cerebellomedullary junction and grow along the path of least resistance, which is typically into the fourth ventricle. For this reason, they can be mistaken for choroid plexus tumors, which arise from the ventricles. They may also arise from the dural covering adjacent to the tentorium cerebelli. Advanced imaging techniques such as CT or MR imaging are necessary to aid in accurate diagnosis. The CT and MRI features of meningiomas have been described (see Chapter 5 and Chapter 6). Briefly, they are strongly contrast-enhancing, well-circumscribed masses that arise from the covering of the brain and, therefore, have a broad-based appearance (Fig. 12.13). There can often be a thickening of the bone adjacent to the tumor (hyperostosis). Choroid plexus tumors are also strongly contrast enhancing; however, these tumors lack the broad-based appearance of meningiomas. Cystic meningiomas have been reported in the dog. A broad-based, contrast-enhancing rim of tissue, filled with fluid, characterizes these tumors (Fig. 12.14). Feline intracranial meningiomas are most often benign growths in that they do not invade surrounding tissue. They are usually well encapsulated and therefore are good candidates for surgical resection (Fig. 12.15). When meningiomas extend into the fourth ventricle, they can be more difficult to remove. Figure 12.13 Transverse MRI of a dog’s brain demonstrating a large, contrast-enhancing cerebellomedullary mass, consistent with a diagnosis of meningioma. Figure 12.14 Dorsal T1-weighted MR image (with contrast), demonstrating a cystic meningioma in a dog’s cerebellum. Figure 12.15 Sagittal T1-weighted MRI (with contrast) of a cat’s brain, demonstrating a large cerebellar meningioma, which was successfully removed. Gliomas are neoplasms that originate from the supporting cells of the CNS (astrocytes and oligodendrocytes). Astrocytomas may be benign or malignant histologically; however, their presence in a confined space (the caudal fossa) compresses surrounding brain structures. They are usually slow growing, have poorly defined margins, and have a heterogeneously contrast-enhancing pattern that may also have ring enhancement. Oligodendrogliomas are round-cell tumors that invade surrounding tissue and are highly destructive. Similar to astrocytomas, oligodendrogliomas have nonuniform contrast enhancement. There are no specific features which allow the differentiation between astrocytomas and oligodendrogliomas on MRI examination. However, oligodendrogliomas are more likely to contact the meninges at least in the prosencephalon. In the case of prosencephalon oligodendrogliomas or astrocytomas, the contrast enhancement of tumors is associated with a higher tumor grade. The incidence of gliomas is greatest in brachycephalic dogs. Choroid plexus tumors and ependymal tumors arise within the ventricles. These tissues are related embryologically and function in the production and movement of CSF. Tumors of ependymal origin are rare. The fourth ventricle is a common site for choroid plexus tumors. The choroid plexus is a very vascular tissue and therefore strongly contrast enhances on MR examination. Both of these tumor types can shed cells into the normal CSF pathways, and therefore a multifocal neuroanatomic localization is possible. Metastases of these tumors via CSF pathways are referred to as “drop” metastases (drop mets). Medulloblastomas are highly metastatic brain tumors, typically occurring in the cerebellum of dogs and cats, usually between 3 and 10 yrs of age. These tumors share many characteristics with the same tumor type in people. They are classified as primitive neuroectodermal tumors. They arise from a population of cells thought to be present during the development of the cerebellum. Animals may present early or later in life with signs of cerebellar disease and possibly signs of brain-stem compression. As the tumors grow into the fourth ventricle, they will often cause obstructive hydrocephalus. Tumor cells will invade the adjacent meninges. Metastasis along CSF pathways is also common. Epidermoid and dermoid cysts are structural abnormalities that may result in cerebellar dysfunction. These masses of tissue are not technically classified as neoplasia; however, because of their location, they create similar clinical signs. It is thought that they arise from an embryological invagination of neuroectoderm. During embryological development, portions of ectoderm destined to become skin become entrapped in the closing neural tube. Because the neural canal closes from a caudal to cranial direction, the fourth ventricle and cerebellomedullary junction is a common site for these abnormalities. They are present at birth; however, the onset of clinical signs usually occurs later in life. Because they are invaginations of tissue destined to become skin, they are filled with keratin and desquamated epithelial cells. They cause cerebellar signs due to the slow compression of surrounding structures. More information on primary brain tumors and epidermoid/dermoid cysts may be found in Chapter 7. A cerebellar vascular hamartoma has been reported in a cat. The cat presented at 16 mos old with a history of cerebellar signs and a localization consistent with cerebellar syndrome. MR imaging of the cat identified a cerebellar mass with poor definition between the mass and normal cerebellar parenchyma. The mass was hyperintense on T2-weighted (T2-W) images and possessed both hyperintense and isointense region on T1-weighted (T1-W) precontrast images. On T1-W post contrast images, there was heterogeneous contrast enhancement with peculiar smaller, isointense, ring-enhancing areas within the mass. A histopathological examination of the mass identified multiple, proliferative endothelial-lined cavities containing thrombi and multiple foci of mineralization. Secondary brain tumors The CNS is a common site of metastatic tumors. The cerebellum may be affected through hematogenous spread or spread through the CSF pathways. The extent and nature of cerebellar dysfunction will be referable to the site of the metastatic tumor (i.e. it may involve other surrounding structures). Common metastatic tumors include mammary adenocarcinoma, prostatic adenocarcinoma, pancreatic adenocarcinoma, pulmonary adenocarcinoma, melanoma, hemangiosarcoma, and lymphoma. Tumors of surrounding structures of the CNS may also compress the cerebellum. These include tumors of the skull, such as osteosarcoma, chondrosarcoma, and multilobular osteochondrosarcoma. More information regarding secondary brain tumors may be found in Chapter 7. Paraneoplastic cerebellar degeneration (PCD) Although relatively rare in humans, there are multiple reports of paraneoplastic cerebellar degeneration in people, and this is the most common paraneoplastic disorder of this species that affects the brain. The immunopathogenesis of this phenomenon is complex, but it generally involves aberrant peripheral (i.e. not CNS) tumor expression of neuronal antigens (usually of Purkinje cells), antigens that normally are sequestered from the immune system via the blood–brain barrier. The resultant autoimmune response against these tumor antigens leads to a secondary autoimmune degeneration of cerebellar neurons (primarily Purkinje cells). Cerebellar dysfunction in such cases is typically acute or subacute in onset and precedes detection of an obvious neoplasm in up to two-thirds of cases. A wide variety of tumors have been associated with PCD in people, but the most commonly reported are small cell lung carcinoma, ovarian carcinoma, breast carcinoma, and Hodgkin’s lymphoma. Diagnosis can be very challenging, especially in the absence of a detectable neoplasm. Brain imaging is often normal, though evidence of cerebellar degeneration may be appreciated. CSF analysis may also be normal, though a mild pleocytosis may be evident. Definitive diagnosis depends upon the identification of serum and/or CSF antibodies directed against specific neuronal antigens (e.g. anti-Yo, anti-Hu antibodies). These antibody tests are not available for dogs and cats. Though not yet reported in dogs and cats, the possibility of PCD should be considered in some patients (e.g. older dogs and cats with obvious cerebellar dysfunction and normal or equivocal brain imaging and CSF findings). Feline (panleukopenia) and canine parvovirus One of the most well-recognized disorders of cerebellar development is the in utero infection of feline embryos with the feline panleukopenia virus (FPV). Kittens infected with FPV either in utero or in the perinatal period may develop cerebellar dysfunction secondary to cerebellar hypoplasia. Infections resulting in clinical signs of cerebellar disease cause inflammation of the brain and destruction of cells in the external germinal layer of the cerebellum. This layer is highly active prior to, and in the first few weeks following, birth. This active division leads to the fully functional cerebellum. Disruption of the division of these cells leads to hypoplasia of the granular layer and gross cerebellar hypoplasia. Purkinje cells that are actively growing may also be affected. Kittens typically present with a nonprogressive, symmetric cerebellar ataxia usually noticed at the onset of ambulation. Occasionally, other areas of the CNS will be affected. With time, most cats will compensate for the cerebellar dysfunction and clinical signs may abate. Amplification of parvoviral DNA from the paraffinized brain tissue of two dogs with congenital cerebellar hypoplasia has been performed implicating the in utero infection of canine parvovirus as a possible cause of the malformation. The polymerase chain reaction (PCR) technique has also been successfully performed in cats with congenital cerebellar hypoplasia. Canine herpes virus The predilection of clinical signs related to cerebellar dysfunction secondary to viral infection is likely due to the developmental nature of the tissue affected. The Purkinje neurons in the cerebellum are exquisitely susceptible to all types of injury. This, when considered with the fact that the granular layer is continuing to develop well into the perinatal time period, provides a plausible explanation as to why clinical signs of cerebellar disease would predominate. Puppies exposed to canine herpes virus either in utero, during parturition, or within the first 2 wks of life can develop a herpes virus meningoencephalitis that can preferentially affect the cerebellum. Nearly all puppies with an active infection early in life will succumb to the infection. However, a few will live and have residual lesions in the CNS. Although other organs may be affected (lung, kidney, liver), signs of a cerebellar syndrome in a newborn puppy would indicate that a herpes virus infection is a likely differential diagnosis. Surviving puppies may also be affected with retinal dysplasia, as this tissue is also undergoing active differentiation at the time of the infection. Canine distemper virus (CDV) Canine distemper virus may affect dogs of any age; however, there is a pattern to the destruction of nervous system tissue that is age-dependent. Those dogs affected with the disease early in life suffer from a polioencephalomyelopathy (gray-matter disease), usually have a history of seizures, and rarely survive. MRI findings associated with the acute form of CDV infection are associated with demyelination and include hyperintense signal changes on T2-W images of the brain. The signal changes are secondary to the accumulation of extracellular water in the space formerly occupied by myelin. Contrast enhancement of the lesions may occur but is not common. There may not be a correlation between the location of the lesion and histopathological confirmation of demyelination. This may be due to the fact that in dogs experiencing seizures similar MRI findings have been observed. However, lesions of the cerebellum are more likely to correlate with histopathological confirmation of demyelination in cases of CDV infection. This MRI characteristic is observed in other demyelinating diseases such as spongy degeneration and globoid cell leukodystrophy; therefore, the MRI changes must be considered in the light of the clinical suspicion for CDV infection. Dogs affected by the disease later in life may have brain-stem, cerebellar, and vestibular signs. They typically have a leukoencephalomyelopathy (white-matter disease) or a combination of gray- and white-matter disease, and in most cases the disease is less severe. The cerebellar peduncles are commonly affected; however, distemper virus can affect all segments of the nervous system. A common sequela of an infection with CDV is a rhythmic myoclonus of a single muscle group that may be mistaken for tremors. Feline infectious peritonitis (FIP) The pyogranulomatous (dry) form of feline infectious peritonitis virus can affect the CNS and may result in inflammation of the ependyma, choroid plexus, or meninges that surround the brain stem and junction of the cerebellum and medulla. This disease can be a challenge to diagnose, as definitive antemortem tests to prove a cat has the disease are virtually nonexistent. In one report, it was found that a high CSF IgG titer against feline coronavirus (greater than 1:25) was predictive of FIP as a causative entity. However, in a later report, CSF IgG titers were not found to be predictive of FIP, but rather suspected to be nonspecifically derived from the systemic circulation. Similarly, it is unclear whether PCR testing of CSF for coronavirus in cats is predictive of the existence of CNS-related FIP. It has been reported that over 45% of cats affected with the dry form of the disease will have signs of CNS dysfunction. This results from an inflammatory response to the virus and a lack of a protective cell-mediated immune response. Because the disease occurs as a multifocal pyogranulomatous meningoencephalomyelitis in the CNS, the neuroanatomic localization can be diverse. Various guidelines have been suggested for the clinician to follow in order to make a presumptive diagnosis. They include a history of possible exposure, clinical signs, high CSF coronaviral titers, and concurrent infection with feline leukemia virus. The disease is usually fatal, except in rare cases. Granulomatous meningoencephalomyelitis Granulomatous meningoencephalomyelitis (GME) has been reported as primarily affecting the cerebellum; however, the condition typically affects multiple areas of the CNS. Three forms of the disease have been described: the disseminated (multifocal) form, the focal form, and the ocular form. The disseminated form usually has a much more rapid onset and progression than the focal form. This disease is typically characterized by a massive perivascular inflammatory response consisting of histiocytes, plasma cells, and lymphocytes, occasionally mixed with other leukocytes. It primarily affects the white matter, but it can affect the gray matter as well. The condition most commonly affects young to middle-aged small-breed dogs and has a higher incidence in females than males. Lesions in the region of the cerebellomedullary angle are common. Affected animals will present with clinical signs referable to structural disease of the cerebellum (i.e. ataxia, tremors, nystagmus). The extensive accumulations of inflammatory cells are at times so proliferative that they compress surrounding tissue. An antemortem diagnosis of GME is based primarily on demonstrating characteristic contrast-enhancing brain lesions on CT or MRI (Fig. 12.16) and the presence of a mixed, predominantly mononuclear, pleocytosis on CSF examination. The treatment of GME is covered in detail in Chapter 7. Corticosteroid therapy may relieve clinical signs, but this is often for a short period of time (weeks to months). When the histiocytes are admixed with inflammatory cells, the distinction is one of a nonsuppurative meningoencephalomyelitis. If histiocytes are the predominant monomorphic form, then the classification is more akin to neoplasia. It has been suggested that the nonsuppurative form of the disease may be a form of neoplasia as well. Because the predominant cell types appear to originate from the monocyte–macrophage cell lines, radiation therapy has been proposed and has been used successfully in the treatment of focal GME. Figure 12.16 Dorsal T1-weighted MRI (with contrast) of dog’s brain, demonstrating a contrast-enhancing GME lesion in the cerebellum. Fungal diseases All of the commonly encountered mycotic organisms may infect the CNS; however, the most frequently seen infections are Cryptococcus neoformans, Blastomyces dermatitidis, and Coccidioides immitis. They typically result in a diffuse or multifocal meningoencephalitis. The CNS may be infected through systemic dissemination (i.e. hematogenously), local extension (e.g. nasal, frontal sinuses), migrating foreign bodies (e.g. grass awns), or surgical procedure (iatrogenically). CNS infections may involve the junction of the cerebellum and the medulla, resulting in cerebellar, vestibular, and other brain-stem signs. Additionally, because of the typical multifocal presentation of these infections, signs of cerebral and spinal cord involvement may be apparent along with cerebellar signs. Fluconazole is the treatment of choice. Additional information regarding CNS fungal infections can be found in Chapter 7. Rickettsial disease The vasculitis caused by either Rocky Mountain spotted fever or ehrlichiosis may cause a meningoencephalitis in dogs. Dogs neurologically infected with a rickettsial disease commonly present with vestibular dysfunction, usually after a history of lethargy, depression, and fever. The hematologic abnormalities secondary to a rickettsial infection or vasculitis may pass without the owners being aware that the animal was infected. Cerebellovestibular signs may be appreciated in combination with clinical signs referable to other areas of involvement in the CNS. Rickettsial diseases are rare in cats. Additional information regarding CNS rickettsial infections can be found in Chapter 7. Protozoal disease The nervous system of both dogs and cats may be infected by the coccidian protozoan Toxoplasma gondii. Neospora caninum, a protozoal organism related to Toxoplasma, is known to naturally infect the nervous system of dogs only. These infections typically present as multifocal disease and are supported by systemic illness. They can infect animals of all ages and breeds. Immunocompromised or immunosuppressed animals are more susceptible to infection. The infections cause necrosis, a nonsuppurative encephalomyelitis of both gray and white matter and may cause cerebellar atrophy. Infectious foci can form granulomas, which may result in signs consistent with focal disease. Clinical signs of cerebellovestibular disease would be expected if the lesion caused compression, atrophy, or inflammation of the cerebellum, cerebellar peduncles, or brain stem. Additional information regarding CNS protozoal infections can be found in Chapter 7. Algal disease Prototheca wickerhamii and Prototheca zopfii are species of ubiquitous algae that have been reported to cause CNS disease. The disease is rare, and it is thought that an abnormality of the animal’s immune system must contribute to the pathogenesis of the infection. Animals will present with systemic signs of illness and multifocal neurologic disease, the clinical signs of which will be dependent upon the area of the nervous system affected. The pyogranulomatous lesions seen in the brains of dogs with protothecosis may cause signs of cerebellovestibular dysfunction. In one case, an eosinophilic pleocytosis was reported. The organism has a predilection for the eyes, so ocular changes may accompany signs of multifocal neurologic disease. Lysosomal storage diseases Lysosomal storage diseases of the CNS represent a group of disorders that have in common the accumulation of metabolic by-products within the perikaryon, axon, dendrites, or surrounding neuropil. Storage diseases can primarily affect the cerebellum; however, they usually are very diffuse in their localization, affecting multiple areas of the brain and spinal cord. Animals with lysosomal storage diseases affecting the cerebellum will typically present at a young age, due to the fact that these diseases are congenital. However, there are few reports of animals presenting with signs of cerebellar disease later in life. Numerous storage diseases may have the potential to affect the cerebellum. A few of the more commonly documented diseases are discussed here. More information concerning lysosomal storage diseases is provided in Chapter 7. Lafora bodies are polyglucosan deposits within the CNS. They have been associated with disease of the CNS in various small animals, including a 4.5-yr-old cat that presented for head bobbing and whole-body tremors. The Lafora bodies were most numerous in the granular layer of the cerebellum and in Purkinje neurons. Neuronal ceroid lipofuscinosis has been most commonly reported in the dog. A similar condition affects cats. The disease results from intraneuronal accumulations of ceroid lipofuscin granules, and it can result in gross cerebellar atrophy. It has been related to primary cerebellar disease in dogs. Dogs with this storage disease will usually present at less than 1 yr old; however, they may not show clinical signs until they mature. Niemann–Pick disease type C in cats causes neurologic deterioration and hepatosplenomegaly. This disease is caused by an accumulation of sphingomyelin and other lipids in the CNS and reticuloendothelial tissues (e.g. spleen, liver). Neuroaxonal dystrophy is the primary histologic feature of the disease. Cats affected by this disease will usually show signs of tremor by 8–12 wks. The disease will slowly progress until the cat is unable to stand. Eventually, the menace responses may disappear as well. In people, those children who have the late infantile form (3–5 yrs old) have primarily cerebellar signs. Although these storage diseases are rare, they should be suspected in young animals that present with slowly progressing cerebellar signs. Ischemic/vascular (Video 21) Because of the cerebellum’s sensitivity to ischemic changes secondary to hypoxia, clinical signs of cerebellar disease may be evident before signs related to other areas of the brain following ischemic events. A definitive antemortem diagnosis of cerebellar infarct is usually not possible. With advanced imaging techniques, such as MRI (Fig. 12.17), however, a presumptive diagnosis can usually be made by combining imaging results with history, signalment, and results of ancillary diagnostic tests. Clinical signs of cerebellar disease will typically be ipsilateral to the area damaged in the cerebellum. If the damage to the cerebellum is minor, one would expect the animal to have a good prognosis, with typical improvement in clinical status seen within the initial 72 hrs after the ischemic episode. Causes of thromboembolic disease such as hyperadrenocorticism, hypothyroidism (atherosclerosis), bacterial endocarditis, heartworm disease, hyperlipidemia, hypertension, and neoplasia must be ruled out as potential causes. Sporadic cases of postanesthetic cerebellar dysfunction have been observed. Clinically normal animals undergoing routine anesthetic procedures have been affected with a cerebellar syndrome. Brachycephalic breeds (e.g. Boston Terriers, Persian cats) may be at a higher risk of developing this anesthetic-related complication. In a retrospective study involving 11 Persian-cross cats affected with cerebellar signs following routine anesthetic procedures, ketamine was used in each case. Although there is no direct implication of ketamine, it is hypothesized that a genetic susceptibility may play a role in the process. Ischemic/vascular encephalopathy is also discussed in some detail in Chapter 7. Figure 12.17 T2-weighted sagittal (A) and transverse (B) MR brain images from a dog with a cerebellar infarct. Intracranial arachnoid cysts (IAC), quadrigeminal arachnoid cysts, ependymal cysts Intracranial arachnoid cysts, sometimes referred to as quadrigeminal cysts, are described in detail in Chapter 7. The development of these anomalous conditions is poorly understood and they may be considered an incidental finding when occurring concurrently with other disorders of the brain. They are most commonly observed in toy breeds; however, they have been reported sporadically in the veterinary literature affecting a wide variety of breeds of dogs and more uncommonly in cats. While they typically arise from the quadrigeminal cistern, they may exert pressure on the cerebellum resulting in compression and possible foramen herniation. The author has observed a case of a cerebellar IAC in a 2-yr-old Rottweiler with a slowly progressive cerebellar syndrome. In this particular case, the IAC arose from the caudal aspect of the tentorium cerebelli and not the rostral aspect as is commonly reported (quadrigeminal cistern). An ependymal cyst has been reported in an 11-wk-old, Staffordshire Bull Terrier. The dog presented with a cerebellar syndrome present since birth. MRI confirmed a fluid-filled cavity in the region of the left cerebellar hemisphere and vermis. Histopathological determination of an ependymal cyst as opposed to an arachnoid cyst was made through demonstration of glial fibrillary acidic-positive glial cells covered multifocally by epithelial cells. A traumatic origin was suspected due to the presence of hemorrhage and tissue destruction. Tremors in dogs and cats can be caused by a variety of conditions affecting the nervous system. The definition of tremor in the human literature is debated based on whether the term “involuntary” should be included. In veterinary medicine, we do not recognize voluntary tremors; therefore the definition for our purposes can be modified from a well-accepted human-related definition of tremor (Box 12.1). A tremor is an involuntary, approximately rhythmic, oscillatory, or roughly sinusoidal movement of a body part. Tremor can be found in both normal and abnormal animals. It can be distinguished from pure myoclonus, which is a sudden contraction of muscles without necessarily a rhythmic, oscillatory, or sinusoidal nature (such as a tic or twitch). However, certain forms of myoclonic clonus can have a rhythmic characterization and therefore be included under the category of tremor. Certainly, in the broadest sense of the definition of a movement disorder, tremor is a distinct subcomponent. For veterinary purposes, the classification of tremor may be based on the phenomenological or the syndromic schemes (Table 12.2). Much of the understanding of the pathophysiology of tremor in humans has been elucidated in the last 20 yrs. Classification schemes are continually being updated, which is a clear indication that no complete understanding of all the possible etiologies of tremor exists. It is important to remember that many of the experiments that have generated the current conclusions regarding the pathophysiology of tremors in people were performed on animals. It is difficult to categorize tremors of dogs and cats in the same groupings as human tremors. Numerous classification schemes have been published in both human and veterinary medical literature. In people, tremors are characterized by their frequency in Hertz (Hz; or cycles per second), the location of the tremor (e.g. fingers, legs, head), and the condition in which the tremor occurs (e.g. at rest, standing, goal-oriented behavior). The origins of tremors are uncertain. The activity of the brain is intensely rhythmic. Oscillators are systems that have the ability to produce rhythmic activity. These are not necessarily discrete anatomic structures. They may likely be neural circuits or connections between subsystems that have an inherent ability to function in this manner. Neurons have the ability to resonate and set up oscillatory firing. The subcortical oscillations of neurons occur through electrical coupling via gap junctions. These neurons resonate at 7–10 Hz, which is the typical range for physiologic tremors. Table 12.2 Classification of tremors in dogs and cats
Cerebellar Diseases and Tremor Syndromes
Cerebellar disorders
Introduction3, 15, 18, 28, 59, 86, 100, 114, 139, 152, 175
Divisions of the cerebellum15, 35, 59, 86, 100, 114, 138, 210
Microscopic anatomy of the cerebellum15, 35, 59, 86, 89, 210, 211
Afferent projections to the cerebellum15, 18, 35, 59, 86, 89, 161, 210, 211
Efferent projections from the cerebellum3, 15, 18, 35, 59, 86, 91
Functions of the cerebellum3, 12, 15, 18, 23, 24, 28, 48, 56, 59, 102, 107, 114, 132, 133, 134, 135, 136, 137, 147, 152, 157, 216
Clinical signs of cerebellar dysfunction3, 15, 18, 59, 86, 100, 119, 155 (Video 9)
Disorders of the cerebellum1–4, 6–8, 10, 13–22, 25, 27–34, 36, 37, 39–43, 45–47, 49–52, 57–62, 64, 66–71, 73–77, 79–82, 84–88, 92, 95–101, 103–106, 108–113, 115–120, 122–128, 130, 140, 141, 143–146, 148–152, 154, 156, 158, 163–170, 172–174, 176–187, 189, 190, 192–199, 202–204, 209, 211, 213, 218–220
Breed
Age of onset
Progressive or stable
Dogs
Airedale Terrier
< 6 mos
Progressive
American Staffordshire
18 mos to 9 yrs
Slowly progressive
Terrier
Australian Kelpie
6–12 yrs
Progressive
Bavarian Mountain dog
3–7 mos
Slowly progressive
Beagle
3 wks
Progressive
Bernese Mountain dog
4–6 wks
Progressive
Border Collie
6–16 wks
Progressive
Brittany Spaniel
7–13 yrs
Slowly progressive
Bull Mastiff
4–28 wks
Progressive
Chinese Cresteda
3–6 mos
Slowly progressive
Coton de Tulearb
8 wks/2 wks
Progressive/Nonprogressive
English Bulldog
8–12 mos
Slowly progressive
Finnish Harrier
< 6 mos
Progressive
Gordon Setter
6–10 mos
Slowly progressive
Irish Setter
3–10 days
Progressive
Jack Russell Terrier
2 wks
Progressive
Kerry Blue Terrier
8–16 wks
Progressive
Labrador Retriever
12 wks
Rapidly progressive
Lagotto Romagnolo
10–15 wks
Rapidly progressive
Miniature Poodle
3–4 wks
Unknown
Rhodesian Ridgeback
Birth
Progressive
Rough Coated Collie
4–8 wks
May stabilize
Samoyed
Birth to 6 mos
Slowly progressive
Cats
Domestic Shorthair
> 1 yr
Slowly progressive
Mixed breed
6–8 wks
Progressive
Persian
7 yrs
Slowly progressive
Siamese
> 1 yr
Slowly progressive
Tremor syndromes5, 9, 11, 26, 38, 44, 53, 55, 63, 65, 72, 78, 83, 90, 93, 94, 121, 129, 131, 142, 153, 159, 162, 171, 188, 191, 200, 201, 205, 208, 212, 214, 215, 217, 221, 222
Pathophysiology and classification
Phenomenologic
Syndromic
A. Resting tremor
B. Action tremor
1. posture tremor
a. position dependent
b. position independent
2. kinetic tremor
a. simple (nontarget directed)
b. target directed (intention)
3. task specific or isometric (not present in nonprimate species
A. Physiologic
B. Enhanced physiologic
C. Essential tremor
1. classic essential tremor
2. intermediate tremor syndrome
3. primary orthostatic tremor
4. position specific tremor
D. Cerebellar tremor syndromes
E. Holmes’ tremor (rest plus intention tremor)
F. Drug induced and toxic tremors
G. Tremors due to peripheral neuropathy
H. Unclassified tremors
1. episodic head tremor syndrome
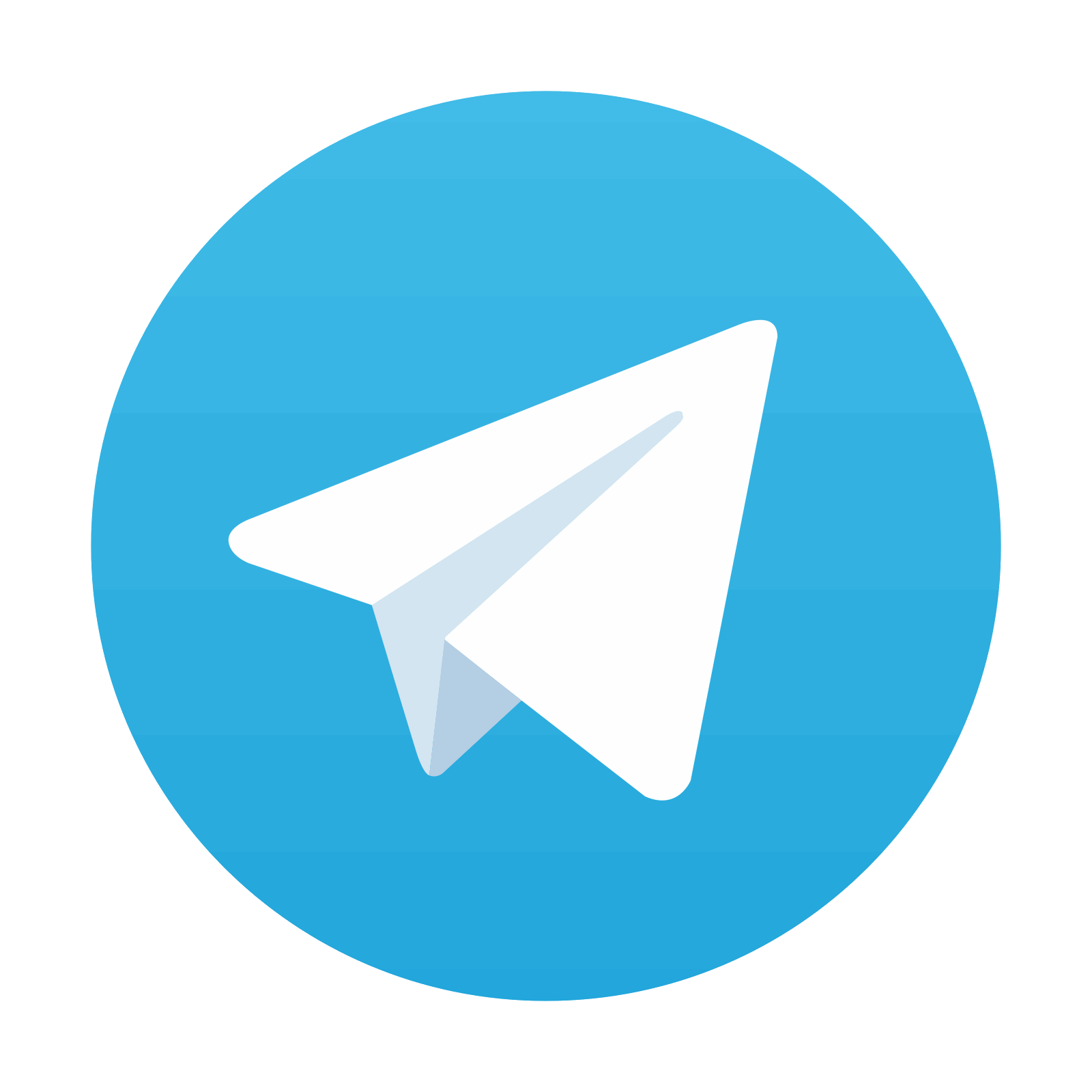
Stay updated, free articles. Join our Telegram channel

Full access? Get Clinical Tree
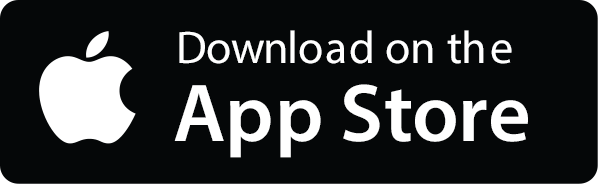
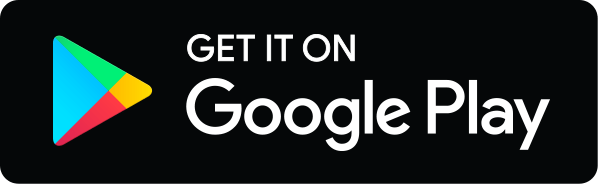