CHAPTER 4 Central Nervous System Infections
Infections of the central nervous system (CNS) of horses, although uncommon, are some of the most devastating and frequently fatal diseases in horses. Diseases such as equine protozoal myeloencephalitis (EPM) and West Nile virus encephalomyelitis (WNE) have had a significant economic impact on the equine industry in recent years and stimulated investigations into preventive, diagnostic, and therapeutic alternatives for CNS infections in horses.
Viral, bacterial, rickettsial, protozoal, parasitic, and fungal pathogens may cause CNS infections in horses (Table 4-1). In small animals and in humans the causes of meningoencephalitis, in order of decreasing frequency, are viral, bacterial, protozoal, rickettsial, parasitic, and fungal, whereas in the horse the most frequently diagnosed CNS infections are probably of viral and protozoal origin.1,2 In an Australian study, 30 of 450 horses with neurologic disease had an infectious or inflammatory disease, and 11 of these 30 had meningitis.3 This study did not reflect the emergence of West Nile virus (WNV) in the United States in 1999 or account for CNS diseases that are present in North America, such as Eastern equine encephalomyelitis (EEE) or EPM.
Table 4-1 Equine Central Nervous System Pathogens
PATHOGEN | SELECT REFERENCES |
---|---|
Viral | |
West Nile virus | 15, 20, 22, 50, 115–119 |
Western equine encephalomyelitis virus | 121–129 |
Eastern equine encephalomyelitis virus | 122, 127, 129, 131–139 |
Venezuelan equine encephalomyelitis virus | 143, 144 |
St. Louis virus | 146 |
Murray Valley virus | 149–151 |
Louping ill virus | 153–157 |
California group/snowshoe hare virus | 159–164 |
Main Drain virus | 170 |
Semliki Forest virus | 171 |
Japanese encephalitis virus | 172 |
Hendra virus | 173 |
Nipah virus | 173 |
Powassan virus | 176 |
Aujeszky’s disease virus | 180 |
Equine herpesvirus type 1 | 41, 42, 181–187 |
Equine infectious anemia virus | 188, 189 |
Rhabdovirus (rabies) | 192 |
Kunjin virus | 151, 194 |
Borna virus | 195 |
Parasitic | |
Setaria | 120 |
Habronema | 130 |
Hypoderma (lineatum, bovis, diana) | 140–142 |
Parastronglyus spp. | 145 |
Strongylus vulgaris | 130, 147, 148 |
Halicephalobus gingivalis | 93–95, 97, 98, 101, 103–109, 113, 114, 152 |
Draschia megastoma | 158 |
Trypanosoma evansi | 165–169 |
Bacterial | |
Escherichia coli | 9, 62 |
Actinobacillus equuli | 62, 79 |
Streptococcus (equi, zooepidemicus, suis) | 7, 27, 62, 70, 174, 175 |
Salmonella | 62, 63, 88 |
Pasteurella caballi | 11, 177–179 |
Pseudomonas aeruginosa | 7 |
Enterococcus | 8 |
Actinomyces | 190, 191 |
Rhodococcus equi* | 25, 79, 81, 82, 86, 89, 193 |
Brucella abortus* | 89 |
Mycobacterium bovis* | 11, 196–198 |
Staphylococcus spp.* | 77 |
Actinobacillus lignieresii* | 80 |
Klebsiella pneumoniae, type 1 | 68 |
Listeria monocytogenes | 36, 64 |
Protozoal | |
Balamuthia mandrillaris | 111 |
Sarcocystis neurona | 18, 48, 199–203 |
Fungal | |
Cryptococcus neoformans | 72–74 |
Aspergillus niger, A. fumigatus* | 75 |
Rickettsial | |
Anaplasma phagocytophilum | 204 |
*Denotes vertebral body osteomyelitis.
Regardless of the type of etiologic agent involved, CNS infections require an accurate and rapid diagnosis and implementation of an appropriate course of treatment by the attending clinician. CNS infection should be suspected in horses with abnormal mentation, seizures, blindness, multiple cranial nerve abnormalities, and general proprioceptive deficits. Infections involving primarily the spinal cord may manifest as limb weakness, incoordination, and stiffness, with or without associated cerebral dysfunction. The reader is referred to chapters on individual diseases for detailed description and discussion of EPM (see Chapter 59), WNV (see Chapter 21), alphavirus encephalitides (see Chapter 20), rabies (see Chapter 19), equine herpesvirus myelopathy (see Chapter 13), Streptococcus equi subsp. equi (see Chapter 28), and Anaplasma phagocytophilum (see Chapter 42). This chapter provides an overview of CNS infection, pathogenesis, diagnosis, and treatment, with discussion of miscellaneous CNS infections not covered elsewhere in this text.
The appropriate term for infection and resultant inflammation of the CNS is determined by the specific area of the nervous system affected. Inflammation of the brain, meninges, spinal cord, and peripheral nerves is termed encephalitis, meningitis, myelitis, and neuritis, respectively. Rhomboencephalitis and cerebellitis refer to localized inflammation of the brain stem and cerebellum, respectively.4,5 Frequently, more than one tissue or anatomic site may be affected. Meningoencephalitis is inflammation of the meninges and brain, and meningoencephalomyelitis is inflammation of the meninges, brain, and spinal cord. Inflammation of the brain and spinal cord, without meningeal involvement, is termed myeloencephalitis.
Infection of the CNS can also result in focal suppuration of the brain parenchyma or spinal cord and formation of abscesses. Localized areas of infection between the outermost meningeal layer (dura mater) and the skull and vertebral column are termed epidural abscesses. Inflammation between the outer two layers of the meninges (dura mater and arachnoid) is termed subdural empyema.1
NEUROANATOMY AND DISEASE
Brain and Meninges
Inside the protective barrier of the skull, the brain is surrounded by three layers of meninges: the outermost dura mater, or pachymeninges, and the leptomeninges, consisting of the inner arachnoid membrane and pia mater (Fig. 4-1). The pia mater is adherent to the external surface of the brain and spinal cord, forming cuffs around penetrating vessels and merging with the ependymal lining of the fourth ventricle. Cerebrospinal fluid (CSF) occupies the subarachnoid space between the pia mater and arachnoid. Acute bacterial infections within the subarachnoid space typically begin with the leptomeninges of the brain and spinal cord and spread inward through the foramina of the fourth ventricle. Infections between the dura mater and arachnoid (subdural empyema) can spread over the entire cerebral hemisphere. The dura mater adheres to the periosteum of the skull and limits the spread of epidural abscesses (between the dura and skull), except where it invaginates into the cranial cavity to form four rigid septa: the falx cerebri, falx cerebelli, tentorium cerebelli, and diaphragma selli.1
The brain rests within the anterior, middle, and posterior cranial fossae, which are associated with the paranasal sinuses. The anterior fossa forms the roof of the frontal and ethmoidal sinuses. The sella turcica is located between the left and right middle fossa and forms the roof of the sphenoid sinuses. Infection in these paranasal sinuses can spread through the respective fossa centrally to the brain, resulting in epidural abscesses and subdural empyema. In the horse, these infections can be either bacterial or fungal.6–10 Middle ear infections (otits media) within the petrous temporal bone may extend into the middle fossa to involve the temporal lobe of the brain or into the posterior fossa to involve the cerebellum or brain stem.1,11
Neuroanatomic localization of brain disease in the horse has been well described.12–14 Briefly, infectious neurologic disease in the horse either is diffuse (e.g., viral or protozoal) or can have a single neuroanantomic signal (e.g., brain abscess). Neuroanatomic localization should be defined in terms of the five major regions of the CNS (and the cranial nerves): disease of the cerebrum, basal nuclei, rostral brain stem, caudal brain stem, and cerebellum.14
Seizure activity and moderate to severe obtundation are the most common signs of cerebral disease in the horse. Although the cortex controls conscious proprioception, this is difficult to localize to the cerebrum in the horse and in the absence of other clinical signs. Blindness occurs secondary to lesions in the visual cortex. Cortical blindness presents as decreased normal reactions to visual cues, with normal pupillary light reflexes. A full ophthalmic examination is paramount to assessing cortical blindness. The most common clinical sign of focal disease of the basal nuclei is the inability to chew and form coordinated eating movements with the tongue, teeth, and oropharynx.12–14 However, diffuse disease in this area involves the caudate nucleus, globus pallidus, putamen, and substantia nigra and should result in loss of coordination of movement. Extension to the reticular formation may result in abnormalities of the wake/sleep cycle.15
Clinical signs referable to lesions of the rostral brain stem can be differentiated on the basis of signs of abnormalities of cranial nerve (CN) II through CN IV. Vision, pupillary responses, and eyeball placement can be affected by disease in these areas. Postganglionic Horner’s syndrome (ipsilateral ptosis, miosis, enophthalmos, and localized ipsilateral sweating) can occur if lesions are rostral to the foramen lacerum involving the sympathetic fibers as they course to the sphincter pupillae muscle (see Chapter 10).12–14
Cranial Nerves
All the cranial nerves exit through the meninges at the base of the brain and are susceptible to injury in horses with meningitis because of direct spread of infection or increased intracranial pressure. The clinical signs of multiple versus single cranial nerve abnormalities are important for ruling out specific etiologies.12–14 For example, a weak horse with primary dysphagia and slow pupillary light responses has evidence of a multifocal or diffuse disease process such as botulism. Unilateral masseter atrophy consistent with CN V paralysis is a common finding in horses with clinical disease caused by Sarcocystis neurona infection.
Spinal Cord
The spinal cord has a central core of gray matter surrounded by the ascending and descending nerve tracts of the white matter. Intramedullary lesions (within the spinal cord) produce neuronal injury at one or more spinal cord segments and then expand laterally to involve motor and sensory nerve tracts. Clinical signs are observed caudal to the site of the spinal cord lesion because of damage to descending motor tracts.12–14 Although clinical signs of spinal cord disease are most often bilateral in horses, severity is frequently asymmetric; a close examination will reveal that most intramedullary lesions, unless extremely focal, will have some degree of abnormality associated with the contralateral limb. On the other hand, extramedullary lesions, or lesions of the peripheral nerves, will involve a single limb. Peripheral or extramedullary lesions can produce signs of nerve root irritation. When a lesion is compressive on the spinal cord, from outward to inward, there is a stepwise loss of proprioception, then weakness. After onset of weakness, further compression results in loss of sensation, followed by loss of deep pain. A typical presentation for a horse with cervical vertebral myelopathy is a young, strong 2-year-old racehorse with spontaneous loss of balance.16,17 Diffuse spinal cord disease is often observed in viral infections such as arbovirus infection, neurologic equine herpesvirus syndrome, rabies, and Halicephalobus infection, whereas multifocal, asymmetric disease is observed in horses with EPM.18,19 West Nile virus can be highly variable, with either diffuse spinal signs or highly asymmetric clinical signs.15,20–22
Spread of infectious agents can be limited by neuroanatomic boundaries. The anatomic arrangements of the spinal meningeal layers (pia mater, arachnoid, and dura mater) are the same as described for the brain, and a plane of infection is possible between the arachnoid and dura. The spinal dura and periosteum diverge at the foramen magnum. At the level of the seventh cervical vertebra (C7), they are separated by a fat-filled epidural space that cannot prevent longitudinal spread of infection, and thus infection may extend over many segments.1 In the horse the spinal cord ends as the cauda equina as the cord tapers into the conus medullaris, with distally coursing spinal nerve roots. Unlike in other species, the meninges end caudally between the second and third sacral vertebrae (S2 and S3) in the horse.12,16 The cauda equina is a site associated with CNS inflammatory diseases and occasional peripheral neuritis.23
Vascularization
The blood supply to the CNS includes an extensive network of intercranial arterial and venous vessels fed by two sources, the basilar and internal carotid arteries, with multiple communications to the external circulatory vessels via the circle of Willis to ensure collateral circulation of the brain. The horse is distinct from other species because the internal carotid artery does not receive any blood from the maxillary artery. The details are beyond the scope of this review; however, some salient features are worth mentioning. The ophthalmic artery is a branch of the internal carotid artery, which is a branch of the main intercranial artery, the basilar artery. Therefore, CNS infection could result in septic emboli to the ophthalmic artery and consequent retinal lesions and loss of some visual fields. The middle cerebral artery has the greatest blood flow volume and is considered the area of greatest risk for septic embolization and mycotic aneurysms in the brain.3 Brain infection most likely arises from infections with Aspergillosis and mucoracious fungi in lungs, uterus, and intestine24 (see Chapters 56 and 57). In descending order of frequency, the internal carotid artery, external carotid artery, and maxillary arteries are the most common equine vessels to be affected with mycotic aneurysm and extracranial (guttural pouch) infection1 (see Chapter 1).
Despite an extensive network of collateral circulation, three areas of the brain are supplied by only one or two vessels. Highly vulnerable to ischemic injury and abscess formation, these areas include (1) the middle and posterior cerebral arteries at the junction of the parietal, occipital, and temporal lobes; (2) the medial surface of the hemispheres of the cerebellum; and (3) cerebral white matter. No valves are present in the venous supply to the CNS, and the direction of flow may change with hemodynamic changes caused by pressure changes in the CSF and conditions such as cerebral edema. The anterior spinal cord is supplied by the cervical and intercostal arteries from the descending aorta and generally has a higher likelihood of infection than other parts of the spinal cord.1,3 Data that support this have not been evaluated in the horse, although osteomyelitis of the cervical and thoracic spinal column may occur in foals.25,26
Blood-Brain Barrier and Cerebrospinal Fluid
The capillary system of the CNS is unique in that it consists of endothelial cells with tight junctions and no fenestrations, creating an effective blood-brain barrier (BBB).1 The BBB is the primary protective barrier of the CNS and acts as a filter preventing access of large proteins, immunoglobulins, antigens, pathogens, and some antimicrobial agents (e.g., gentamicin, amphotericin B) to the brain.1 Injury to the BBB by ischemic insult (e.g., septic emboli), vasculitis induced by inflammation, or increased levels of tumor necrosis factor alpha (TNF-α) can disrupt this protective barrier and predispose to CNS infection. Disruption of the BBB permits the entry of radiodense agents into the CNS for early visualization of abscesses on contrast magnetic resonance imaging (MRI) and computed tomography (CT) studies.7,27 The blood supply to the pituitary gland, choroid plexus, and brain stem does not have tight junctions, and these areas are considered to exist outside the BBB.
Cerebrospinal fluid is an ultrafiltrate of plasma produced by active secretion from the choroid plexus in the lateral, third, and fourth ventricles and by diffusion across the meninges.1,28–30 CSF protects and sustains the CNS.31,32 It circulates outward through the ventricular foramina into the subarachnoid space and is reabsorbed over 3 to 4 hours through cells of the arachnoid villi along the superior sagittal sinuses. Blockage of the villi from inflammation, blood in the subarachnoid space, or occlusion of the superior sagittal or lateral sinuses prevents the reabsorption of CSF, and communicating hydrocephalus develops. Obstructive hydrocephalus results from blockage of CSF circulation at the ventricles caused by inflammation or compression of the ventricles, as might occur with abscess or hemorrhage. Unlike communicating hydrocephalus, redistribution of increased quantities of CSF and cerebral edema into the subarachnoid space is not possible in obstructive hydrocephalus, and there is increased likelihood of brain herniation and death.1,32
PATHOGENESIS
Entry of Pathogens
Most neurotropic viruses gain initial entry to the body through the bite of an infected mosquito or insect (e.g., arboviruses), the respiratory tract (e.g., herpesvirus), or the gastrointestinal (GI) tract. Dendritic cells or phagocytes at the site of initial infection transport virus to local lymph nodes, where it undergoes primary replication with subsequent viremia. Initial infection with bacterial or fungal organisms most often occurs through the respiratory, GI, reproductive, and urinary tracts.2 Septic emboli from vegetative endocarditis are another potential source of bacteria or fungi for hematogenous spread to the CNS. Regardless of the initial route of infection, the majority of CNS pathogens probably enter the nervous system of the host by a hematogenous route.1,2,5
The exact mechanism by which pathogens cross the BBB and enter the CNS is uncertain for most viruses and bacteria, but several mechanisms have been proposed. Bacterial infections of the CNS frequently involve the meninges. A breakdown in the BBB due to ischemia of meningeal vessels secondary to emboli and inflammation may provide a route of access to the brain parenchyma with subsequent abscessation.33–35 The initial systemic immune response to viral infection in the periphery results in release of cytokines, which stimulate increased expression of adhesion molecules on CNS endothelial cells and increased surveillance of the CNS by activated T cells.5 Some viruses enter the CNS using these cells as a “Trojan horse.” Other viruses use endothelial adhesion molecules to gain entry or induce release of TNF-α, with subsequent increased BBB permeability.5 Intracellular pathogens, such as Listeria monocytogenes and rickettsial species, gain entry into the CNS by penetrating endothelial cells of the BBB or by traveling within phagocytes.36
Other than hematogenous spread, pathogens may access the CNS by direct invasion (trauma or iatrogenic introduction), spread from contiguous structures (e.g., paranasal sinuses, otitis media), or retrograde entry along nerve roots.2,5,33,37 Despite the frequency of infections involving the equine head (e.g., sinusitis, tooth root abscesses, guttural pouch empyema), the number of CNS infections resulting from direct spread to the CNS appears to be low in horses.37 Rabies virus gains entry to the CNS by retrograde axonal transport along peripheral nerves. Herpesvirus is thought to infect the peripheral trigeminal nerve during latent phases.2 The potential for entry to the brain through the free nerve endings of the olfactory nerve in the nasal cavity has been proposed for rabies virus and arboviruses, especially Venezuelan equine encephalitis (VEE) virus, but has not been proved clinically.38
Immune Response of Central Nervous System
The response of the CNS to infection plays an important role in the pathogenesis of disease. In bacterial CNS infections, CSF concentrations of complement and immunoglobulin G (IgG) are low compared with concentrations in the peripheral circulation.33 Complement and specific antibody are important for opsonization of bacteria, and a diminution of this function may be a critical factor in the pathogenesis of bacterial infections in the CNS. The presence of bacterial cell wall components in the CSF elicits the release of cytokines (e.g., interleukin-6 [IL-6], TNF-α, macrophage inflammatory protein [MIP-1a, -1b, -2]), which stimulate the entry of neutrophils, increased BBB permeability, and vasculitis; CNS edema; and inflammation of tissues surrounding the meninges.
The peak inflammatory response is observed 72 hours after the start of infection.33 Degenerating leukocytes release toxins that stimulate vasospasm, local ischemia, and further tissue edema. Inflammation of the arachnoid villi where CSF is absorbed could result in communicating hydrocephalus; inflammation of the ependymal lining and ventricles where CSF is circulated may result in obstructive hydrocephalus. Initially there is redistribution of increased CSF and cerebral edema into the subarachnoid space with communicating hydrocephalus, but with severe edema and obstructive hydrocephalus, this redistribution is not possible. Within the confining structures of the skull, the increase in intracranial pressure may result in pressure necrosis of the brain parenchyma or death due to herniation of the cerebellum through the foramen magnum.34 Vasogenic edema of the CNS is now viewed as a potentially fatal consequence of bacterial infection, and treatment of human patients with both antimicrobial and antiinflammatory medications has dramatically decreased the mortality associated with CNS infection.38
When infection occurs, the CNS must mount a controlled adaptive immune response that minimizes damage to brain cells.5 Initially there is an innate immune response, with production of interferon-β (IFN-β), chemokines, and proinflammatory cytokines. These mediators activate microglia to express interleukin-1 (IL-1), TNF-α, and chemokines that stimulate increased expression of endothelial adhesion molecules (e.g., vascular cell adhesion molecule [VCAM-1], intracellular adhesion molecule [ICAM]). By 3 to 4 days after infection, peripheral inflammatory cells that were activated in secondary lymphoid tissues enter the CNS. Unlike in the periphery, nonlytic clearance of viruses and infected cells occurs in the CNS to prevent secondary damage to surrounding neurons and tissues. Viruses that remain latent in neurons are controlled by continued secretion of antibody, IFN-β, and IFN-γ by long-term lymphocytes.
As with bacterial CNS infections, control or elimination of viral infection in the CNS, without inducing unacceptable damage to neural tissue, requires a delicate balance of the CNS immune response. Induction of apoptosis (cell death) of neurons by microglia and stimulation of migration of T cells into the CNS are possible contributing factors to the neurodegeneration observed in degenerative diseases, such as Parkinson’s and Alzheimer’s disease.39 Overexpression of C protein, important in the complement system, causes bystander neurodegeneration and oligodendrocyte damage.40
The immune response to equine herpesvirus type 1 (EHV-1) may be important in the pathogenesis of the neurologic form of this pathogen. Localization of EHV-1 in the CNS endothelium induces vasculitis. Subsequent CNS damage results from ischemia rather than direct neuronal insult; thus the disease is termed a “myeloencephalopathy” rather than “myeloencephalitis.”41 The exact pathogenesis of herpesvirus neurologic disease in horses remains unclear. The disease is sporadic and seems to be more common in horses with a previous history of exposure to this ubiquitous pathogen and in pregnant or lactating mares. Evidence of antigen-antibody complexes between EHV-1 antigen and EHV-4 antibody and decreased levels of complement activation have been observed in experimentally infected ponies.42,43
Other factors also play a role in the pathogenesis of viral infections. Nonsurvivors of Japanese encephalitis virus (JEV), a flavivirus infection, have increased levels of IL-6, interferon-α (IFN-α), and interleukin-8 (IL-8). Host genetic factors influencing production of these cytokines may be a factor.44 Regardless of the immune response, viruses have developed strategies to facilitate evasion of the CNS immune response. For example, WNV may block the signaling pathway for IFN-α.45
Understanding of the host response to viral CNS infections is increasing and may explain the seemingly improper or inadequate immune response that allows the establishment of persistent infection. In human patients, certain types of vaccination or viral infection result in a multifocal inflammatory demyelinating process or acute disseminated encephalomyelitis (ADEM).4 An immune-mediated attack against antigen in brain myelin appears to be the cause of ADEM. The poliomyelitis-like, acute flaccid paralysis observed in some people with West Nile encephalitis may be an example of ADEM.46,47
The old paradigm of the CNS as incapable of mounting an immune response to infection and being “immunologically privileged” has given way to the current recognition of the CNS as a specialized immune organ.39 The innate and adaptive immune responses of the host play an important role in CNS infections. A better understanding of this role is important for ultimately developing novel therapeutic and preventive strategies.
CEREBOSPINAL FLUID CHARACTERISTICS
Collection Techniques
Cerebrospinal fluid may be obtained antemortem from two sites in horses: the atlanto-occipital (cerebellomedullary) space (Fig. 4-2) and the lumbosacral (LS) space (Fig. 4-3). The optimal site for sample collection is determined on the basis of the neuroanatomic localization of the suspected lesion and practical considerations regarding patient systemic health status and restraint options. In general, better diagnostic results are achieved if CSF is obtained from the site closest to the suspected lesion. The atlanto-occipital (AO) space is sampled under general anesthesia and may be preferable in nervous horses, horses undergoing anesthesia for another reason, or in horses with conformation preventing successful LS taps (LS subluxation). Conversely, a LS tap performed standing under sedation may be advantageous in an animal where recovery from general anesthesia is considered a risk because of the severity of neurologic disease.
Collection techniques for both AO and LS tap have been described in detail.11,28,30 Briefly, atlanto-occipital CSF collection is performed with the horse under general anesthesia and lying in lateral recumbency. An area of the poll and neck (15-20 cm caudal to ears and 8-10 cm on either side of mane) is clipped and surgically prepped. The head is flexed so that the median axis of the head is at right angles to the median axis of the cervical vertebrae. A sterile 8.9-cm, 20-gauge spinal needle with stylet is inserted at the intersection of the cranial borders of the atlas and the external occipital protuberance along the dorsal midline. The needle should be parallel to the ground, perpendicular to the skin, and aimed toward the nose of the horse. The needle is gradually advanced until a “popping” sensation is felt with penetration of the AO membrane and cervical dura. The stylet is withdrawn, and the appearance of clear CSF at the hub indicates a successful procedure. If no CSF appears when the stylet is removed, the needle is rotated 90 degrees. If fluid is still not obtained, the stylet is replaced, and the needle is advanced carefully. The approximate depth of needle insertion for entry into the subarachnoid space is 5 to 8 cm. If the needle contacts bone at a depth of 2 to 5 cm, it should be withdrawn and repositioned appropriately. If blood appears at the hub of the needle when the stylet is removed and does not clear with CSF in 15 to 20 seconds, the stylet is replaced and the needle removed; a fresh needle is used for the next attempt. When CSF flows freely from the hub of the needle, the sample is collected by free flow or gentle aspiration into an appropriate tube. After the sample has been collected and the needle is withdrawn, the head of the horse is extended to a normal or slightly extended position to prevent leakage of CSF from the puncture site.30
Lumbosacral CSF collection in the horse is typically performed with the sedated horse standing as squarely as possible.12,30 Landmarks for the LS site are the intersection of imaginary lines joining the caudal borders of the tuber coxae along the dorsal midline or at the highest point of the gluteal region of the horse. In addition to sedation, adequate restraint with a twitch and use of stocks are advisable. In response to penetration of the dura mater, sedated horses may show no reaction, or tail movement and slight flexion of the pelvic limbs, or violent kicking responses that can endanger the patient and the veterinarian. A 10 × 10-cm site is clipped and sterilely prepped. A 20-gauge, 15.2-cm spinal needle with stylet is inserted in a sterile manner and advanced carefully a few millimeters at a time. Care should be taken to keep the needle perpendicular to the dorsum and on midline. A “popping” sensation may be felt with penetration of the LS interarcuate ligament, dorsal dura mater, and arachnoid membrane. The stylet is removed to check for CSF at the hub. Gentle aspiration with a syringe may be necessary to initiate flow of spinal fluid. If no fluid is obtained, the needle (with the stylet replaced) is advanced to the floor of the vertebral canal and then withdrawn with slow rotation of the needle a millimeter at a time. A needle depth of 12 to 14 cm is usually required for successful CSF collection. Large-breed horses or obese horses may require longer needles. Queckenstedt’s maneuver (bilateral occlusion of the jugular veins) may be performed by an assistant to increase intracranial and intraspinal pressure and facilitate CSF flow up the spinal needle. Rotation of the needle 90 degrees to remove occluding meningeal tissue and nerve roots from the needle point may also be helpful. Indirect aspiration with a syringe through an extension set connected to the spinal needle hub is recommended to minimize hemorrhage from excessive suction pressure and resultant occlusion of the needle with meninges. After adequate CSF is obtained, the stylet is replaced in the spinal needle, and the needle is removed. Collection of CSF from the LS space while the horse is in lateral recumbency (under general anesthesia or in a tetraplegic horse) is possible but is considered more difficult than in the standing horse. Attempts may be facilitated by elevating the upper pelvic limb so that the tuber coxae are perpendicular to the floor or by advancing the pelvic limbs cranially to flex the pelvis and LS joint.
Both AO and LS collection techniques are regarded as safe procedures in the horse. A common complication is blood contamination of the sample with puncture of meningeal or spinal cord vessels. Initial blood contamination of CSF frequently clears after a few milliliters during collection; however, even microscopic amounts of blood in the CSF sample may result in false-positive results in testing for EPM in horses.48 In humans, cerebellar herniation through the foramen magnum and herniation of the temporal cortex under the tentorium cerebelli are considered potential complications of CSF collection, especially in patients with increased intracerebral pressure, severe meningitis, or brain abscesses with deteriorating condition.1 This complication has not been reported as a frequent sequela to CSF collection in horses. Evidence of extradural hemorrhage or formation of fibrous adhesions between the LS ligament and dorsal LS dura mater have been observed in experimental subjects postmortem. Penetration of the AO joint is another potential complication. Cellulitis and septic abscesses secondary to CSF collection in horses are rare.
Analysis
Analysis of the CSF may include measurement of CSF pressure and examination of sample cytology, total protein concentration, glucose concentration, biochemical alteration, turbidity, and color.28,30 CSF pressure is measured by attachment of a manometer to the hub of the spinal needle before collection. Normal CSF pressure in the horse is approximately 300 mm H2O (150-500 mm H2O).16,30,49 Increased opening pressure, when CSF is first obtained, may result from obstructive hydrocephalus. In addition to noninfectious congenital abnormalities, potential causes of obstruction include tumor, abscess, hemorrhage, and edema. An increased opening pressure that decreases by 20% to 50% after removal of 1 to 2 mL of CSF is indicative of an intracranial mass or spinal cord lesion cranial to the site of collection. Because CSF flows caudad from the ventricles of the brain, and because jugular compression causes increased blood volume in the cranial cavity with subsequent increases in CSF pressure, failure of the CSF pressure to increase in the LS site with bilateral jugular vein compression may indicate a compressive thoracic or cervical lesion.
Appearance
Normal CSF is clear and colorless and does not clot. Xanthochromia (yellow discoloration) of the CSF after centrifugation is caused by preexisting trauma, vasculitis, increased protein concentration (150 mg/dL), direct bilirubin leakage from high serum concentration, or breakdown of the BBB.16,30,49 Xanthochromia with increased protein concentration is typical of equine encephalomyelopathy caused by vascular inflammation and increased BBB permeability.42,43
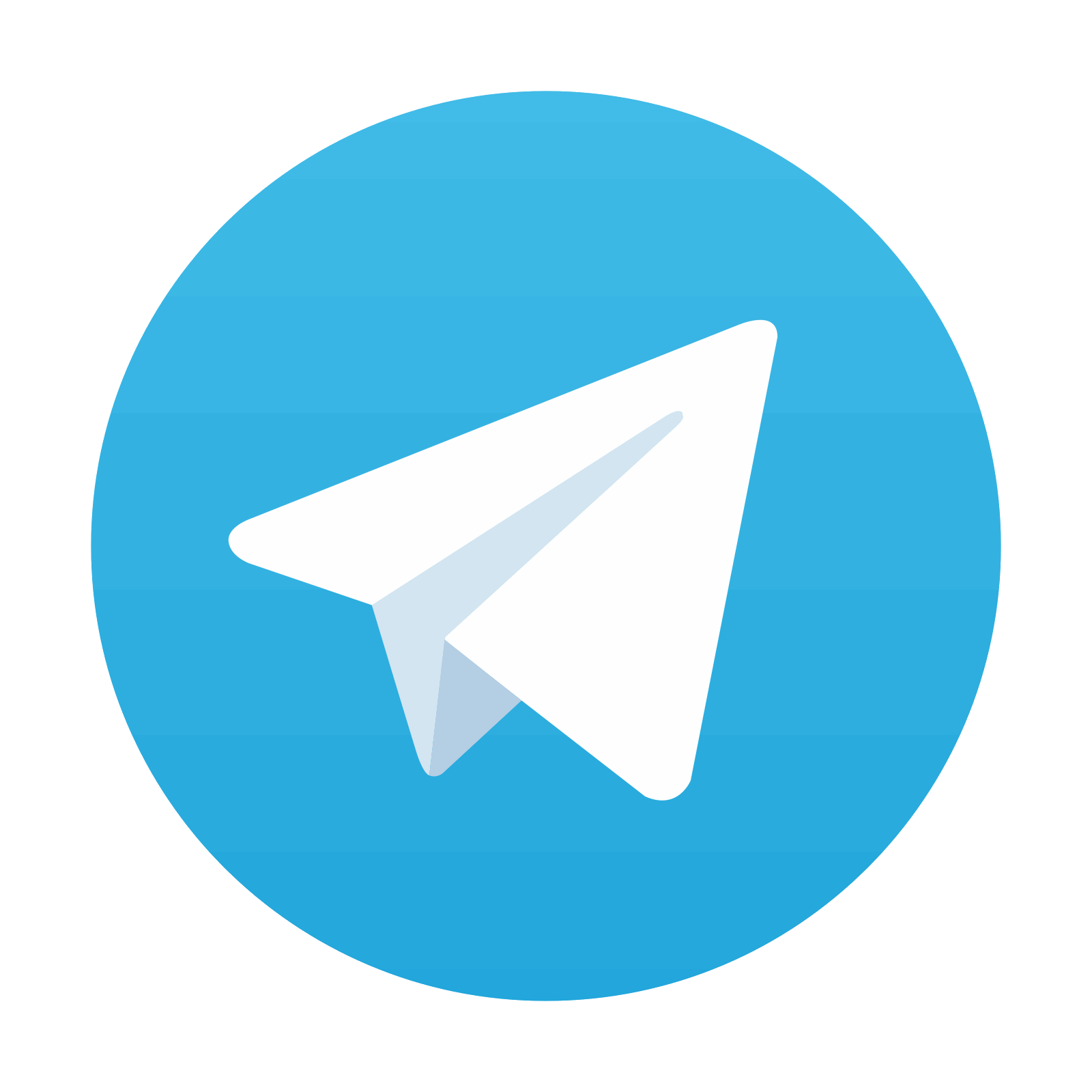
Stay updated, free articles. Join our Telegram channel

Full access? Get Clinical Tree
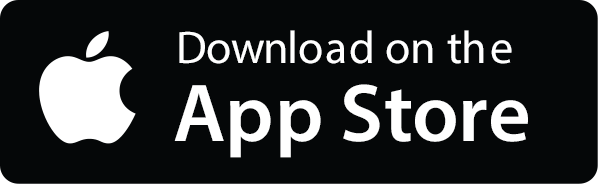
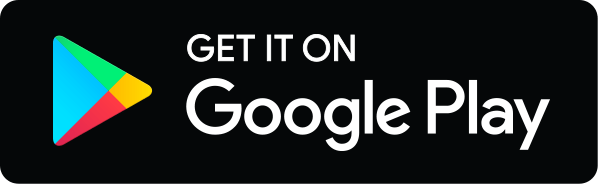