Chapter 11 CANINE DIABETES MELLITUS
The endocrine pancreas is composed of the islets of Langerhans, which are dispersed as “small islands” in a “sea” of exocrine-secreting acinar cells. Four distinct cell types have been identified within these islets on the basis of staining properties and morphology—alpha cells, which secrete glucagon; beta cells, which secrete insulin; delta cells, which secrete somatostatin; and F cells, which secrete pancreatic polypeptide. Dysfunction involving any of these cell lines ultimately results in either an excess or a deficiency of the respective hormone in the circulation. In the dog and cat, the most common disorder of the endocrine pancreas is diabetes mellitus, which results from an absolute or relative insulin deficiency due to deficient insulin secretion by the beta cells. The incidence of diabetes mellitus is similar for the dog and cat, with a reported frequency varying from 1 in 100 to 1 in 500 (Panciera et al, 1990).
CLASSIFICATION AND ETIOLOGY
TYPE 1 DIABETES MELLITUS.
Type 1 diabetes mellitus is characterized by a combination of genetic susceptibility and immunologic destruction of beta cells with progressive and eventually complete insulin insufficiency (Eisenbarth, 1986; Palmer and McCulloch, 1991). Autoantibodies are directed against several islet components, including insulin, beta cell, and glutamic acid decarboxylase (GAD) (Srikanta et al, 1985; Verge et al, 1996; Gorus et al, 1997). Serum anti-insulin, anti-beta cell, and/or anti-GAD autoantibodies are commonly identified at the time diabetes is diagnosed and are also detected early in the development of type 1 diabetes, prior to the onset of hyperglycemia or clinical signs (Verge et al, 1996; Gorus et al, 1997). Screening individuals for serum anti-insulin, anti-beta cell, and anti-GAD autoantibodies is used to identify individuals at risk for development of type 1 diabetes. Identification of anti-GAD autoantibodies or positive results for all three autoantibodies has the highest predictive value for development of type 1 diabetes (Tuomilehto et al, 1994; Schatz et al, 1994; Hagopian et al, 1995).
Immune-mediated destruction of the islets has been conceptually divided into six stages in humans, beginning with genetic susceptibility (Table 11-1) (Eisenbarth, 1986). Stage 2 involves a triggering event that leads to beta-cell autoimmunity. Environmental factors that trigger the development of beta-cell immunity are poorly defined but probably include drugs and infectious agents. One interesting environmental factor that has been proposed in humans involves consumption of cow’s milk during infancy. Some humans with newly diagnosed insulin-dependent diabetes mellitus (IDDM) have antibodies to bovine serum albumin and a 17-amino acid bovine serum albumin peptide. It has been hypothesized that the ingestion of cow’s milk in early life can initiate beta-cell destruction through molecular mimicry between these proteins in cow’s milk and a beta-cell protein (Karjalainen et al, 1992; Savilahti et al, 1993; Gerstein, 1994), although there is skepticism concerning an etiologic role of these antibodies (Atkinson et al, 1993). Stage 3 is the period of active autoimmunity, but normal insulin secretion is maintained. During stage 4, immunologic abnormalities persist, but glucose-stimulated insulin secretion is progressively lost, despite maintenance of euglycemia. Overt (clinical) diabetes develops in stage 5, although some residual insulin secretion remains. Stage 6 is characterized by complete beta-cell destruction. As a result, individuals with type 1 diabetes are dependent on insulin to control glycemia, avoid ketoacidosis, and survive. Based on our current understanding of the disorder, it seems likely that the pathogenesis of diabetes mellitus in dogs progresses through similar stages. Unfortunately, we do not typically identify diabetes in dogs until stage 5 or 6, at which time insulin therapy is mandatory (Fig. 11-1).
TABLE 11-1 STAGES IN THE DEVELOPMENT OF IMMUNE-MEDIATED INSULIN-DEPENDENT DIABETES MELLITUS IN HUMANS
Stage 1 | Genetic susceptibility |
Stage 2 | Triggering event |
Stage 3 | Active autoimmunity and normal insulin secretion |
Stage 4 | Active autoimmunity and impaired insulin secretion |
Stage 5 | Overt diabetes with residual insulin secretion |
Stage 6 | Overt diabetes with complete beta-cell destruction |
TYPE 2 DIABETES MELLITUS.
Type 2 diabetes mellitus is characterized by insulin resistance and “dysfunctional” beta cells (Reaven, 1988; Leahy, 1990), defects that are believed to be genetic in origin, evident for a decade or longer before hyperglycemia and clinical signs of diabetes develop, and with deleterious effects that can be accentuated by environmental factors such as obesity (Warram et al, 1990; Martin et al, 1992). Debate still continues among human diabetologists about which defect—insulin resistance or impaired insulin secretion—initiates the cascade of events leading to overt diabetes mellitus. Hepatic resistance to insulin, potentially induced by increased free fatty acids in the portal circulation, results in excessive basal hepatic glucose production and fasting hyperglycemia (DeFronzo et al, 1989; Groop et al, 1989; Bergman and Mittleman, 1998; Massillon et al, 1997). Muscle insulin resistance impairs the ability of endogenously secreted insulin to augment muscle glucose uptake in response to a meal, resulting in an excessive postprandial increase in the blood glucose concentration (Mitrakou et al, 1990). Defects in insulin receptor function, insulin receptor–signal transduction pathway, glucose transport and phosphorylation, glycogen synthesis, and glucose oxidation contribute to muscle insulin resistance (DeFronzo, 1997). Impaired insulin secretion also plays a major role in the pathogenesis of glucose intolerance in humans with type 2 diabetes (Polonsky, 1995). Total amounts of insulin secreted during glucose tolerance testing may be increased, decreased, or normal compared with the normal fasting animal. However, relative to the severity of insulin resist-ance and prevailing hyperglycemia, even elevated serum insulin concentrations are deficient (Saad et al, 1989). As the fasting blood glucose concentration increases, insulin secretion decreases progressively, and by the time the fasting blood glucose concentration is greater than 180 to 200 mg/dl, the insulin response to glucose tolerance testing is deficient in absolute terms.
Humans with type 2 diabetes are typically not dependent on insulin to control the disease. Control of the diabetic state is usually possible through diet, exercise, and oral hypoglycemic drugs. However, insulin treatment may be necessary in some type 2 diabetics if insulin resistance and beta cell dysfunction are severe. As such, humans with type 2 diabetes can have IDDM or non–insulin-dependent diabetes mellitus (NIDDM). Insulin resistance and impaired insulin secretion have been identified in dogs and cats with obesity (Nelson et al, 1990; Appleton et al, 2001), and obesity is accepted as a contributing factor for the development of diabetes mellitus in dogs and cats (Panciera et al, 1990; Scarlett and Donoghue, 1998). Low insulin sensitivity has also been identified in a group of lean cats subsequently identified to be at greater risk for developing impaired glucose tolerance with obesity than lean cats with higher insulin sensitivity that subsequently developed obesity (Appleton et al, 2001). The authors speculated that these cats may be more at risk for progressing to overt diabetes mellitus. The role of genetics, if any, for variations in insulin sensitivity and risk for developing diabetes in cats remains to be determined.
INSULIN-DEPENDENT AND NON–INSULIN-DEPENDENT DIABETES MELLITUS.
The classification of human diabetics as type 1 or type 2 is based on familial history, clinical presentation, and results of immunologic (e.g., identification of serum anti–beta cell and anti-insulin autoantibodies) and insulin secretagogue tests (Unger and Foster, 1998). In contrast, classifying diabetes as IDDM and NIDDM is based on the need for insulin therapy to control glycemia, avoid ketoacidosis, and survive. Individuals with IDDM must receive insulin to avoid ketoacidosis, whereas control of glycemia and avoidance of ketoacidosis can be accomplished through diet, exercise, and oral hypoglycemic drugs in individuals with NIDDM. Approximately 10% of diabetic humans in the United States have type 1 diabetes, which is insulin-dependent. Approximately 90% of diabetic humans have type 2 diabetes, which may be insulin-dependent or non–insulin-dependent, depending on the severity of insulin resistance and beta cell dysfunction.
It is more clinically relevant and perhaps more accurate to classify diabetes in dogs and cats as IDDM or NIDDM rather than as type 1 or type 2. Familial history is rarely available in diabetic dogs and cats; the clinical presentation is usually not helpful in differentiating type 1 and type 2 diabetes, especially in cats (Nelson et al, 1993); insulin secretagogue tests are not routinely performed, and their results may be misleading (Kirk et al, 1993); and autoantibody tests for type 1 diabetes are not readily available. Therefore, as clinicians, we usually classify diabetic dogs and cats as either IDDM or NIDDM based on their need for insulin treatment. This can be confusing because some diabetics, especially cats, can initially appear to have NIDDM progressing to IDDM, or flip back and forth between IDDM and NIDDM as severity of insulin resistance and impairment of beta-cell function waxes and wanes. Apparent changes in the diabetic state (i.e., IDDM and NIDDM) are understandable when one realizes that islet pathology may be mild to severe and progressive or static; that the ability of the pancreas to secrete insulin depends on the severity of islet pathology and can decrease with time; that responsiveness of tissues to insulin varies, often in conjunction with the presence or absence of concurrent inflammatory, infectious, neoplastic, or hormonal disorders; and that all these variables affect the animal’s need for insulin, insulin dosage, and ease of diabetic regulation. Use of serum insulin concentrations to differentiate between IDDM and NIDDM is discussed on page 495.
INSULIN-DEPENDENT DIABETES IN DOGS.
In our experience, virtually all dogs have IDDM at the time diabetes mellitus is diagnosed. IDDM is characterized by hypoinsulinemia, essentially no increase in endogenous serum insulin concentration following administration of an insulin secretagogue (e.g., glucose or glucagon) at any time following diagnosis of the disease, failure to establish glycemic control with diet and/or oral hypoglycemic drugs, and an absolute necessity for exogenous insulin to maintain glycemic control. The cause of IDDM has been poorly characterized in dogs but is undoubtedly multifactorial (Table 11-2). Genetic predispositions have been suggested by familial associations in dogs and by pedigree analysis of Keeshonds (see Table 11-3) (Guptill et al, 1999; Hess et al, 2000a). The most common pathologic lesions in dogs with diabetes mellitus are a reduction in the number and size of pancreatic islets, a decrease in the number of beta cells within islets, and hydropic ballooning degeneration of beta cells (see Fig. 12-1, page 541). In some dogs, an extreme form of the disease may occur, represented by a congenital absolute deficiency of beta cells and pancreatic islet hypoplasia or aplasia (Alejandro et al, 1988; Atkins et al, 1988). Less severe changes of pancreatic islets and beta cells may predispose the adult dog to diabetes mellitus after it has been exposed to environmental factors, such as infectious agents, insulin-antagonistic diseases and drugs, obesity, and pancreatitis. Environmental factors may induce beta cell degeneration secondary to chronic insulin resistance or may cause release of beta cell proteins that induce immune-mediated destruction of the islets (Nerup, 1994). Studies evaluating for anti-beta cell autoantibodies in diabetic dogs have been conflicting, having been identified in newly diagnosed diabetic dogs with IDDM in one study (Hoenig and Dawe, 1992) but not in another (Haines, 1986). Immune-mediated insulitis has also been described in diabetic dogs (Alejandro et al, 1988). Seemingly, autoimmune mechanisms, in conjunction with environmental factors, may play a role in the initiation and progression of diabetes in dogs.
TABLE 11-3 BREED RISKS FOR DEVELOPING DIABETES MELLITUS DERIVED FROM ANALYSIS OF THE VETERINARY MEDICAL DATABASE (VMDB) FROM 1970 TO 1993*†
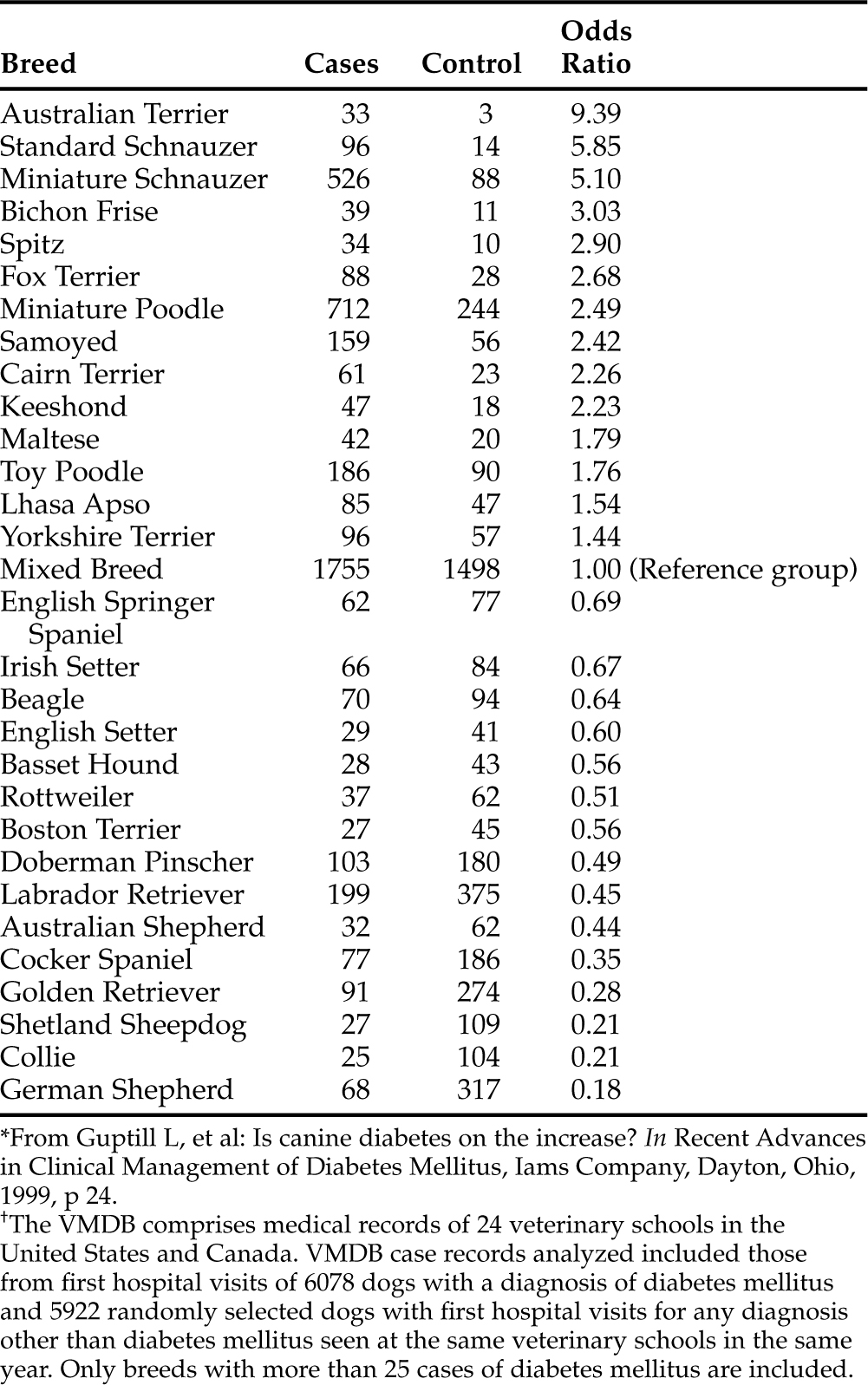
NON–INSULIN-DEPENDENT DIABETES IN DOGS.
Obesity-induced carbohydrate intolerance has been documented in dogs (Mattheeuws et al, 1984), and minute amounts of amyloid have been identified in the islets of some dogs with diabetes mellitus. Despite these findings, clinical recognition of NIDDM is very uncommon in the dog. A juvenile form of canine diabetes mellitus that closely resembles human maturity-onset diabetes of the young, a subclassification of NIDDM, has been described. Measurement of plasma C-peptide concentrations during insulin response testing also suggests the presence of some continuing beta-cell function in a small percentage of diabetic dogs (see Fig. 11-1). C-peptide is the connecting peptide found in the proinsulin molecule and is secreted into the circulation in equimolar concentrations as insulin. Increased plasma C-peptide concentrations in these latter dogs suggest either a severe form of type 2 diabetes mellitus or residual beta-cell function in dogs with type 1 diabetes mellitus. Unfortunately, clinical characteristics of juvenile canine NIDDM and the presence of some C-peptide secretory capabilities resemble IDDM, in that dogs with these conditions are treated with insulin to manage hyperglycemia.
TRANSIENT DIABETES IN DOGS.
Transient or reversible diabetes is extremely uncommon in dogs and usually occurs in dogs with subclinical diabetes treated with insulin antagonistic drugs (e.g., glucocorticoids) or in the very early stages of an insulin-antagonistic disorder (e.g., diestrus in the bitch, hyperadrenocorticism). Such dogs have a reduced but adequate mass of functional beta cells to maintain carbohydrate tolerance when insulin resistance is not present, but they are unable to secrete an adequate amount of insulin to maintain euglycemia in the presence of insulin antagonism. Early recognition and correction of the insulin antagonism may reestablish euglycemia without the long-term need for insulin therapy. Failure to quickly correct the insulin antagonism will result in progressive loss of beta cells, eventual development of IDDM, and the life-long requirement for insulin treatment to control the hyperglycemia. Additional causes of transient or reversible IDDM include NIDDM and the “honeymoon period” of IDDM. A transient increase in insulin secretion and reduced insulin dosage requirements may occur during the initial weeks to months after the diagnosis of IDDM in humans; this is called the honeymoon period (Rossetti et al, 1990). A syndrome similar to the honeymoon period occurs in some newly diagnosed diabetic dogs and is characterized by excellent glycemic control using dosages of insulin considerably less than what would be expected (i.e., <0.2 U/kg per injection) (Fig. 11-2). Presumably, the existence of residual beta-cell function when diabetes is diagnosed (see Fig. 11-1) and correction of glucose toxicity (see page 544) after initiation of insulin therapy account for the initial ease of treating the diabetic state. Continuing progressive destruction of residual functioning beta cells results in worsening loss of endogenous insulin secretory capacity and a greater need for exogenous insulin to control the diabetes. As a result, glycemic control becomes more difficult to maintain, and insulin dosages increase to more commonly required amounts (0.5 to 1.0 U/kg per injection). This increase in insulin requirements usually occurs within the first 6 months of treatment.
SECONDARY DIABETES IN DOGS.
Secondary diabetes mellitus is carbohydrate intolerance secondary to concurrent insulin antagonistic disease or medications. Examples include the bitch in diestrus and the cat treated with megestrol acetate (progesterone). Hyperinsulinemia may be initially present in these animals. However, with persistence of the insulin antagonistic disorder, beta-cell function becomes impaired, and permanent diabetes mellitus, typically IDDM, may develop. If the insulin antagonistic disorder resolves while some beta-cell function is still present, permanent overt diabetes mellitus may not develop. However, animals that become euglycemic after treatment of the insulin antagonistic disorder or discontinuation of the insulin antagonistic drug remain candidates to become “subclinical” diabetics, and an attempt should be made to avoid insulin antagonistic drugs and disorders to prevent overt diabetes mellitus from developing.
Older bitches occasionally develop diabetes during diestrus or pregnancy, presumably as a result of the insulin antagonistic actions of progesterone (see page 526). The diabetic state may resolve once the progesterone concentration declines to anestrual levels or may persist and require life-long insulin therapy. Development of diabetes during diestrus or pregnancy resembles gestational diabetes in humans. In humans, gestational diabetes is restricted to pregnant women in whom the onset or recognition of diabetes or impaired glucose tolerance occurs during pregnancy (Unger and Foster, 1998). After pregnancy termination, the woman may remain clinically diabetic, may revert to a subclinical diabetic state with impaired glucose tolerance, or may revert to a normal glucose-tolerant state. For the latter groups, the risk for developing overt diabetes later in life ranges from 10% to 40%, depending on severity of obesity. Bitches with transient diabetes caused by diestrus have a high likelihood of developing permanent IDDM during the next estrus. For this reason, all bitches with “gestational” diabetes should be spayed as soon as possible after diabetes is diagnosed.
Once the diagnosis of diabetes is established, dogs should be considered to have IDDM, and treatment with insulin should be initiated, even if secondary diabetes mellitus is suspected. Measurement of baseline serum insulin concentration or evaluation of serum insulin concentrations following administration of an insulin secretagogue (e.g., glucose, glucagon; see Table 12-1, page 547) are generally not recommended in newly diagnosed diabetic dogs because of the high prevalence of IDDM. The vast majority of newly diagnosed diabetic dogs will have either undetectable serum insulin concentrations or serum insulin concentrations in the lower half of the normal reference range (i.e., less than 12 μU/ml); findings consistent with IDDM and hypoinsulinemia. Measurement of serum insulin concentration may provide prognostic information in newly diagnosed diabetic dogs in which there is a strong suspicion of secondary diabetes mellitus (e.g., intact bitch in diestrus; severe acute pancreatitis). Serum insulin concentrations greater than one SD above the reference mean (>18 μU/ml in our laboratory) suggest the existence of functional beta cells and the possibility for secondary diabetes mellitus and the potential for reversion to a non-insulin-requiring state if the underlying cause of the insulin antagonism can be identified and corrected. Insulin treatment is still recommended in these situations to correct hyperglycemia and decrease the “stress” placed on the beta cells while waiting for the insulin antagonism to resolve. In theory, glucose toxicity may interfere with accurate assessment of serum insulin concentrations in diabetic dogs in a manner similar to that in diabetic cats (see page 544) (Nelson et al, 1998a). However, the syndrome of glucose toxicity has not yet been clearly established in diabetic dogs, in part because most dogs are truly hypoinsulinemic at the time diabetes mellitus is diagnosed.
PATHOPHYSIOLOGY
The interaction of the “satiety center” in the ventromedial region of the hypothalamus with the “feeding center” in the lateral region of the hypothalamus is responsible for controlling the amount of food ingested (Ganong, 1991). The feeding center, responsible for evoking eating behavior, is chronically functioning but can be transiently inhibited by the satiety center after food ingestion. The amount of glucose entering the cells in the satiety center directly affects the feeling of hunger; the more glucose that enters these cells, the less the feeling of hunger and vice versa (Ganong, 1991). The ability of glucose to enter the cells in the satiety center is mediated by insulin. In diabetics with a relative or absolute lack of insulin, glucose does not enter satiety center cells, resulting in failure to inhibit the feeding center. Thus these individuals become polyphagic despite hyperglycemia.
The four classic signs of diabetes mellitus are polyuria, polydipsia, polyphagia, and weight loss. The severity of these signs is directly related to the severity of hyperglycemia. As these signs become obvious to the owner, the pet is brought to the veterinarian for care. Unfortunately, some dogs and cats are not identified by their owners as having signs of disease, and these untreated animals may ultimately develop diabetic ketoacidosis (DKA). See Chapter 13 for a detailed discussion of the pathophysiology of DKA.
SIGNALMENT
Most dogs are 4 to 14 years old at the time diabetes mellitus is diagnosed, with a peak prevalence at 7 to 10 years of age. Juvenile-onset diabetes occurs in dogs less than 1 year of age and is uncommon. Female dogs are affected about twice as frequently as male dogs. Genetic predispositions for or against the development of diabetes have been suggested by familial associations in dogs and by pedigree analysis of Keeshonds (see Table 11-3; Guptill et al, 1999; Hess et al, 2000a). Breed popularity can also affect perceptions of predisposition. For example, epidemiologic studies suggest that the Labrador retriever is at decreased risk for development of diabetes mellitus; yet this is one of the most frequently affected breeds in our practice, presumably because of their popularity in our region.
ANAMNESIS
The history in virtually all diabetic dogs includes the classic signs of polydipsia, polyuria, polyphagia, and weight loss. Polyuria and polydipsia do not develop until hyperglycemia results in glycosuria. Occasionally an owner brings in a dog because of sudden blindness caused by cataract formation (Fig. 11-3). The classic signs of diabetes mellitus may have gone unnoticed or been considered irrelevant by the owner. If the clinical signs associated with uncomplicated diabetes are not observed by the owner and impaired vision caused by cataracts does not develop, a diabetic dog is at risk for the development of systemic signs of illness as progressive ketonemia and metabolic acidosis develop (see page 584). The time sequence from the onset of initial clinical signs to the development of DKA is unpredictable, ranging from days to weeks.
PHYSICAL EXAMINATION
Performance of a thorough physical examination is imperative in any dog suspected to have diabetes mellitus, in part because of the high prevalence of concurrent disorders that can affect response to treatment. The physical examination findings in a dog with newly diagnosed diabetes depend on whether DKA is present and its severity, on the duration of diabetes prior to its diagnosis, and on the nature of any other concurrent disorder. The nonketotic diabetic dog has no classic physical examination findings. Many diabetic dogs are obese but are otherwise in good physical condition. Dogs with prolonged untreated diabetes may have lost weight but are rarely emaciated unless concurrent disease (e.g., pancreatic exocrine insufficiency) is present. Lethargy may be evident; the haircoat may be sparse, with dry, brittle, and lusterless hair; and scales from hyperkeratosis may be present. Diabetes-induced hepatic lipidosis may cause hepatomegaly. Lenticular changes consistent with cataract formation are another common clinical finding in diabetic dogs. Additional abnormalities may be identified in the dog with diabetic ketoacidosis (see page 584).
ESTABLISHING THE DIAGNOSIS OF DIABETES MELLITUS
A diagnosis of diabetes mellitus requires the presence of appropriate clinical signs (i.e., polyuria, polydipsia, polyphagia, weight loss) and documentation of persistent fasting hyperglycemia and glycosuria. Measurement of the blood glucose concentration using a portable blood glucose monitoring device (see page 512) and testing for the presence of glycosuria using urine reagent test strips (e.g., KetoDiastix; Ames Division, Miles Laboratories Inc., Elkhart, Ind.) allows the rapid confirmation of diabetes mellitus. The concurrent documentation of ketonuria establishes a diagnosis of diabetic ketosis or ketoacidosis.
It is important to document both persistent hyperglycemia and glycosuria to establish a diagnosis of diabetes mellitus, because hyperglycemia differentiates diabetes mellitus from primary renal glycosuria, whereas glycosuria differentiates diabetes mellitus from other causes of hyperglycemia (Table 11-4). Stress-induced hyperglycemia is a common problem in cats and occasionally occurs in dogs, especially those that are very excited, hyperactive, or aggressive. Refer to page 567 for more information on stress-induced hyperglycemia.
TABLE 11-4 CAUSES OF HYPERGLYCEMIA IN DOGS AND CATS
Mild hyperglycemia (i.e., 130 to 180 mg/dl) is clinically silent and is usually an unexpected and unsuspected finding. If the dog with mild hyperglycemia is examined for polyuria and polydipsia, a disorder other than clinical diabetes mellitus should be sought, most notably hyperadrenocorticism. Mild hyperglycemia can occur up to 2 hours postprandially in some dogs following consumption of soft moist foods (Holste et al, 1989), in “stressed” dogs, in early diabetes mellitus, and with disorders causing insulin resistance (see page 524). A diagnostic evaluation for disorders causing insulin resistance is indicated if mild hyperglycemia persists in the fasted, unstressed dog. Insulin therapy is not indicated in these animals, because clinical diabetes mellitus is not present.
CLINICAL PATHOLOGIC ABNORMALITIES
OVERVIEW OF PATIENT EVALUATION.
A thorough evaluation of the dog’s overall health is recommended once the diagnosis of diabetes mellitus has been established to identify any disease that may be causing or contributing to the carbohydrate intolerance (e.g., hyperadrenocorticism), that may result from the carbohydrate intolerance (e.g., bacterial cystitis), or that may mandate a modification of therapy (e.g., pancreatitis). The minimum laboratory evaluation in any “healthy” nonketotic diabetic dog should include a CBC, serum biochemical panel, and urinalysis with bacterial culture. Serum progesterone concentration should be determined if diabetes mellitus is diagnosed in an intact bitch, regardless of her cycling history. If available, abdominal ultrasound is indicated to assess for pancreatitis, adrenomegaly, pyometritis in an intact bitch, and abnormalities affecting the liver and urinary tract (e.g., changes consistent with pyelonephritis or cystitis). Because of the relatively high prevalence of pancreatitis in diabetic dogs, measurement of serum lipase or serum trypsin–like immunoreactivity (TLI) should be considered if abdominal ultrasound is not available. Measurement of the baseline serum insulin concentration or an insulin response test is not routinely done. Additional tests may be warranted after obtaining the history, performing the physical examination, or identifying ketoacidosis. The laboratory evaluation of dogs with glycosuria and ketonuria is discussed in detail in Chapter 13, page 586. Potential clinical pathologic abnormalities are listed in Table 11-5.
TABLE 11-5 CLINICOPATHOLOGIC ABNORMALITIES COMMONLY FOUND IN DOGS AND CATS WITH UNCOMPLICATED DIABETES MELLITUS
IDDM, Insulin-dependent diabetes mellitus; NIDDM, non-insulin-dependent diabetes mellitus.
SERUM BIOCHEMICAL PANEL.
The prevalence and severity of abnormalities identified in the serum biochemistry panel are dependent on the duration of untreated diabetes and the presence of concurrent disease, most notably pancreatitis. The serum biochemical panel is often unremarkable in “healthy” diabetic dogs without significant concurrent disease, aside from hyperglycemia and hypercholesterolemia. The most common abnormalities are an increase in serum alanine transaminase and alkaline phosphatase activities and hypercholesterolemia. The increase in liver enzyme activities is usually mild (less than 500 IU/L) and a result of hepatic lipidosis. Serum alkaline phosphatase activities in excess of 500 IU/L should raise suspicion for concurrent hyperadrenocorticism, especially if other abnormalities consistent with hyperadrenocorticism are identified in the laboratory data (see Chapter 6). Serum alanine transaminase activities in excess of 500 IU/L should raise suspicion for hepatopathy other than hepatic lipidosis, especially if additional abnormalities in endogenous liver function tests (e.g., low urea nitrogen, hypoalbuminemia, increased serum bile acids) are identified. An increase in the serum total bilirubin concentration should raise suspicion for extrahepatic biliary obstruction caused by concurrent pancreatitis. When appropriate, abdominal ultrasound and histologic evaluation of a liver biopsy specimen may be indicated to establish concurrent liver disease.
The BUN and serum creatinine concentrations are usually normal in the uncomplicated diabetic. An elevation in these parameters may be due to either primary renal failure or prerenal uremia secondary to dehydration. Primary renal failure as a result of glomerulosclerosis, damage specifically related to hyperglycemia, is a well-recognized complication in humans but is uncommon in dogs (see Diabetic Nephropathy, page 532). Evaluation of urine specific gravity should help differentiate primary renal failure from prerenal uremia.
Alterations in serum electrolytes and acid-base parameters are common in pets with DKA and are discussed in the section dealing with therapy for the severe ketoacidotic diabetic (see page 596).
URINALYSIS.
Proteinuria may be the result of urinary tract infection or glomerular damage secondary to disruption of the basement membrane (Struble et al, 1998). Because of the high incidence of infection, the urine sediment should be carefully inspected for changes consistent with infection, including white blood cells, red blood cells, and bacteria. Failure to identify pyuria and hematuria does not rule out urinary tract infection (McGuire et al, 2002). Because of the relatively high prevalence of concurrent urinary tract infections in diabetic dogs, urine obtained by antepubic cystocentesis using aseptic technique should be submitted for bacterial culture and sensitivity testing in all dogs with newly diagnosed diabetes mellitus, regardless of the findings on urinalysis (Hess et al, 2000b).
SERUM CHOLESTEROL AND TRIGLYCERIDE CONCENTRATIONS.
Hyperlipidemia and obvious lipemia are common in the untreated diabetic. Uncontrolled diabetes is accompanied by an increase in the blood concentration of triglycerides, cholesterol, lipoproteins, chylomicrons, and free fatty acids (DeBowes, 1987). Hypertriglyceridemia is responsible for the lipemia, which can be seen in a peripheral blood sample. Hypertriglyceridemia with increases in chylomicrons and very low density lipoprotein (VLDL) triglycerides results from insulin deficiency and the associated curtailment in lipoprotein lipase activity (Eckel, 1989). The enzyme lipoprotein lipase aids in the metabolism of the triglyceride-rich VLDLs and chylomicrons. Increased concentrations of VLDL triglyceride also result from excess hepatic production (induced by increased circulating free fatty acids), obesity, and a high caloric intake (Massillon et al, 1997; Unger and Foster, 1998).
Overall, blood cholesterol concentrations are increased in diabetics, but to a much lesser degree than is hypertriglyceridemia. In humans, several factors contribute to an increase in LDL cholesterol concentrations, including increased LDL synthesis from VLDLs; reduced activity of LDL receptors, impairing LDL clearance; and excess consumption of saturated fatty acids (Howard et al, 1987; Unger and Foster, 1998). In humans, high density lipoprotein (HDL) cholesterol levels are often low, presumably as a result of accelerated catabolism (Witzum et al, 1982). The combination of high LDL and low HDL cholesterol concentrations may play a role in the accelerated development of atherosclerotic vascular disease and coronary heart disease, which is the major long-term complication of diabetes in humans (Garg and Grundy, 1990). Similar vascular complications have been infrequently documented in diabetic dogs and cats (Hess et al, 2002). Fortunately, most lipid derangements can be improved with insulin and dietary therapy.
PANCREATIC ENZYMES.
Blood tests to assess for the presence of pancreatitis should always be considered in the newly diagnosed diabetic dog, especially if abdominal ultrasound is not available. Measurement of serum lipase concentration and serum TLI are most commonly recommended. In theory, dogs with concomitant active pancreatitis should have an increase in serum lipase concentration and serum TLI. Unfortunately, serum lipase concentrations and serum TLI do not always correlate accurately with the presence or absence of pancreatitis (Hess et al, 1998). Pancreatic enzyme concentrations can be increased in dogs with a histologically confirmed normal pancreas and normal in dogs with histologically confirmed inflammation of the pancreas, especially when the inflammatory process is chronic and mild. For these reasons, interpretation of serum lipase and TLI results should always be done in context with the history, physical examination findings, and additional findings on the laboratory tests. In our experience, abdominal ultrasound is the single best diagnostic test for identifying pancreatitis in the dog and should be considered if pancreatitis is suspected after evaluation of the history, physical examination, and laboratory test results. The concomitant presence of pancreatitis may necessitate the instigation of intensive fluid therapy and a highly digestible, low-fat diet, which may otherwise not have been done. Identification of chronic pancreatitis also has important prognostic implications regarding success of establishing and maintaining control of glycemia and long-term survival (see page 528).
Measurement of serum TLI is also used to diagnose exocrine pancreatic insufficiency; an uncommon complication of diabetes mellitus that presumably develops as a sequela of chronic pancreatitis (Wiberg et al, 1999; Wiberg and Westermarck, 2002). Exocrine pancreatic insufficiency should be suspected in diabetic dogs that are difficult to regulate with insulin and are thin or emaciated despite polyphagia (see page 528).
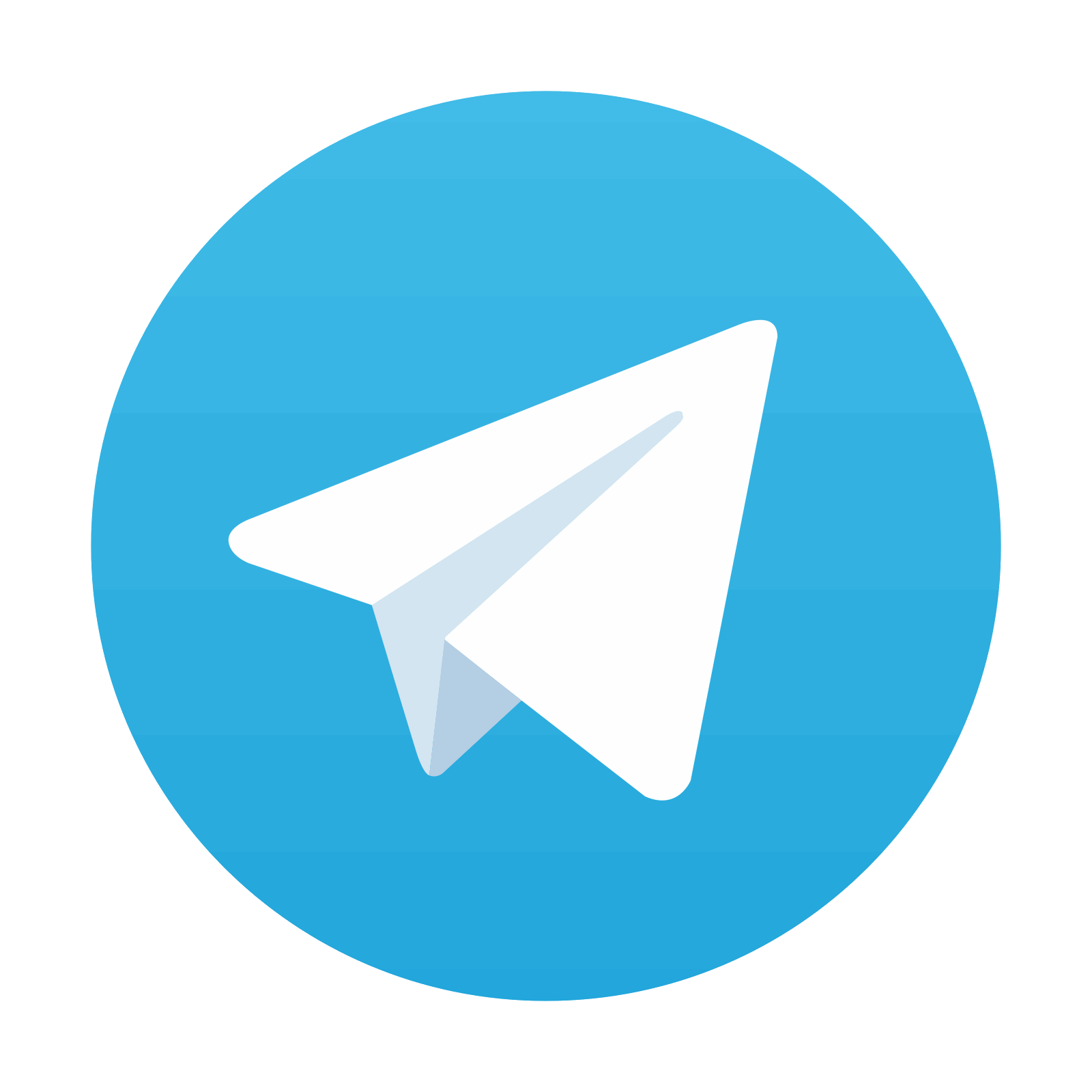
Stay updated, free articles. Join our Telegram channel

Full access? Get Clinical Tree
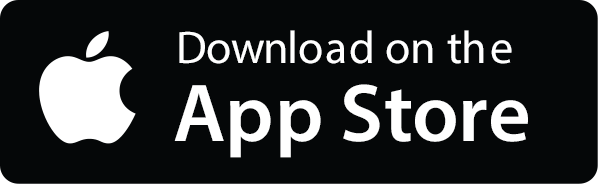
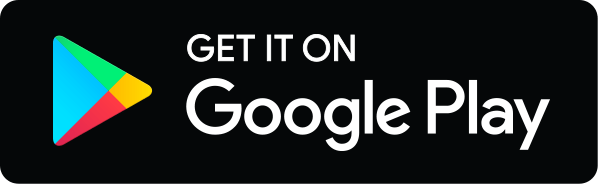