Chapter 14
Canine Blood Collection
Kenichiro Yagi
Adobe Animal Hospital, Los Altos, California, USA
Introduction
Once a canine blood donor has been carefully selected according to certain requirements and screening criteria, the next step in producing viable blood products is blood collection. Blood collection from canine donors for transfusion purposes is very different than diagnostic sampling, since it requires collection of a large volume of blood using blood banking specific supplies and equipment. Blood collection for transfusion can be performed routinely with high frequency at commercial blood bank operations, or infrequently when urgent need for individual transfusions arise at practices experiencing low transfusion caseloads. How donations are scheduled will depend heavily on the practice type, transfusion demand, and donor pool size.
Blood donation in veterinary medicine differs from human blood donations in that canine donors do not voluntarily decide to donate; the owners are giving consent for blood to be collected. Despite the fact that blood is being taken from an animal that cannot give explicit consent, there is a heavy demand for canine blood products. Whereas canine donors, in comparison to feline donors, are much more likely to have a positive donation experience with attention to minimizing stress and using positive reinforcement, the inability for dogs to give consent creates an ethical dilemma. Blood is a limited, yet necessary, biological resource. Since blood donation is not a completely benign process, veterinary professionals have the responsibility to ensure the best possible donation experience by considering the safety and comfort of the donor. This must be balanced with the production of the highest quality blood product in order to make the most of each donation.
Donor selection
Appropriate donor selection is the first step in ensuring the best possible donation experience and quality of the blood product. Many factors are considered, such as health status, medical history, age, body weight, temperament, and physical characteristics. These allow selection of donor dogs that are most unlikely to be harmed by the donation process and able to donate in the most pleasant manner possible. Dogs should be of excellent health with no previous exposure to blood products, between 1 and 8 years of age, at least 50 lb (22.7 kg) of lean body weight, agreeable and stress free during handling and restraint, and have easily accessible jugular veins. Negative screens for bloodborne pathogens, administration of ectoparasite and endoparasite preventatives, up-to-date vaccination status, and being healthy without the need for other medications are all requirements intended to ensure the safety of the blood product derived during the donation. Determining whether to include the dog in the donor program should be performed prior to the need for blood collection arising in order to provide donors urgently should a patient require an emergency transfusion or a blood bank require re-stocking of unit deficits. More details regarding proper canine donor selection can be found in Chapter 13.
Donor eligibility
On the day of donation, an additional set of criteria must be fulfilled by the owner and donor to proceed with the donation. While an extensive set of selection criteria will have been met during the screening process, most of these factors confirm eligibility at the time of entry into the donor program. Therefore, confirmation of this status as unchanged is required prior to blood collection to continue promoting the donor’s health and recipient’s safety. Evaluation of the donor’s eligibility to perform a donation is based on the donor’s recent history, owner’s consent, physical examination findings, and hematologic parameters.
History and consent
After the owner and donor are greeted warmly and shown to the designated waiting area at the hospital or donation location, the intent to donate should be reconfirmed. Informed consent to donate is important as blood donation is not without risk and the possibility of long-term effects is currently unknown. A consent form identifying the owner and donor with confirmation of legal ownership should be signed by the owner. The form should provide details regarding the blood collection procedure such as the volume of blood to be collected, the estimated duration of the procedure, whether sedation will be used, and the amount of time the donor will need to stay after the donation for monitoring. Specific potential risks and common complications of donation (e.g., bruising, venipuncture wounds, hypotension, prolonged procedure, and sedation complications) should be listed as reminders to owners of possible harm.
A questionnaire can be provided, within the consent form or separately, confirming a lack of general signs of illness (i.e., normal appetite and thirst, no coughing, sneezing, vomiting, or diarrhea), and prompting for updates in the medical history, as well as risk factors (e.g., surgery, treatments, medications aside from preventatives, vaccinations, and travel) that could potentially prevent the donor from donating. Reiteration of risks in a direct and accurate manner in layperson terms is especially important to ensure the owners’ comprehension and reaffirm their commitment to the donation. It is equally important to assure the owners that the safety of their dog is the priority of the blood collection team, with standard treatment protocols in place to allow for upholding high standards of care. This will aid in the establishment of trust between owners and the blood collection team, alleviating any potential anxiety regarding donation, as well as promoting understanding when unavoidable complications arise. Completed forms should be kept as a part of the donor’s records.
Frequency of donation
Another important consideration is the previous donation date. While a well-organized record and donor communication system will minimize chances of blood donations being performed too frequently, the records should be reconfirmed prior to each donation to prevent any unintentionally short rest interval between donations.
The ideal rest interval between donations allows enough time for the body to replace the red blood cells (RBCs) lost during donations without any permanent impact on the dog’s health. Earlier studies demonstrate that dogs weighing 23–27 kg are able to donate a unit of blood every 3–4 weeks without the need for any nutritional supplementation (Potkay and Zinn 1969). While these results suggest that donations can be made this frequently, current awareness of animal health and the human–animal bond calls for assurance that the dog and owner’s lifestyle, often involving outdoor activities, will not be disrupted by being a donor. This typically means extending the donation interval to every 3–4 months to avoid donor and owner fatigue or burnout.
One study compared hematologic values and serum concentrations of iron, transferrin, and ferritin in dogs donating 13% total blood volume (TBV) or 11.4 mL/kg every 2 months, 13% TBV every 3 months, or 15% TBV (13.2 mL/kg) every 3 months for a year. Donors within all groups were able to restore depleted RBCs within 10 days after collection and maintained normal iron stores. However, the group of dogs donating 13% TBV every 2 months showed a significant decrease in iron stores. Therefore, monitoring of these values was recommended for programs using 8-week donation intervals (Ferreira et al. 2014).
Another study compared serum iron concentration, unsaturated iron-binding capacity, total iron-binding capacity, and percentage transferrin saturation between experienced donors (mean of 13.6 donations), less frequent donors (mean of 3.8 donations), and a control group (healthy dogs receiving wellness exam or elective surgeries). In the donors that donated 450 mL of blood an average of every 8.5 weeks and as short as every 4.8 weeks, there were no significant differences seen in all values measured. However, dogs that donated on average 14 or more consecutive donations at an average of 8.5 week intervals had decreased transferrin saturation percentage, and increased unsaturated iron-binding capacity and total iron-binding capacity when compared to less frequent donors. This indicated some depletion in iron stores, although the serum iron concentration remained within the normal reference range (Zaldívar-López et al. 2014).
These studies suggest that an 8-week interval for donations is sustainable without negative changes in PCV or clinical signs, although long-term donation this frequently can deplete iron stores. Therefore, waiting 8–12 weeks between donations is less likely to be harmful to dogs if used for long-term donations and iron supplementation could be considered for dogs donating at shorter intervals.
Physical examination
Once the consent form is completed, the veterinarian or veterinary technician should review the questionnaire answers for any changes since the last donation that could increase the risks of donation. The donor age and weight should be confirmed to be within the recommended ranges. Each donor should then receive a thorough physical examination to confirm their health. The physical examination involves a thorough head-to-tail assessment, including auscultation of the heart for abnormal rhythms or murmurs, auscultation of the chest for any abnormal lung sounds, and palpation of the lymph nodes and abdomen for any abnormalities. The heart rate, pulse quality, respiratory rate and effort, and temperature should be recorded. Any signs of illness should lead to forgoing the donation and be addressed appropriately.
Blood tests
Packed cell volume and total protein
In addition to the annual comprehensive blood work and bloodborne pathogen screening, evaluation of packed cell volume (PCV) and total protein (TP) on the day of the donation are required. A complete blood count allows for evaluation of abnormalities within the hematopoietic system, including platelet count, but is not required unless previous concerns have been noted. Canine donors should ideally have a PCV of ≥40% or a hemoglobin concentration of ≥13 g/dL (130 g/L) on the day of the donation to minimize the possibility of anemia from the donation. The minimum PCV acceptable is the low end of the normal range, or 35%. The PCV is influenced by the donor’s hydration and plasma volume status, leading to dilution or concentration when the donor is well hydrated or dehydrated, respectively. Because of this, proper assessment of hydration status during the physical examination, coupled with interpretation of the TP, is necessary to prevent induction of anemia. A total protein between 6.0 and 8.6 g/dL (60 and 86 g/L) is considered normal in an otherwise healthy dog.
When evaluating the donor’s TP, the visual characteristic of the plasma should also be evaluated; any discolorations should be investigated as that could indicate hemoglobinemia (hemolysis), bilirubinemia (icterus), or lipemia. Because hemolysis could be an artifactual finding due to traumatic blood sampling, a sample should be carefully redrawn to confirm the finding. If hemolysis of the sample persists, additional investigation is indicated to rule out an underlying disease.
Lipemia
Lipemia can be transient and simply related to the timing of the donor’s last meal (Figure 14.1). However, it is important to record the finding to confirm that it is not chronic and something that requires further investigation. Documentation of the association between hyperlipidemia and detrimental effects on RBC membranes and life span has been found in many species. These negative effects can be induced by feeding high cholesterol diets and include anemia secondary to acanthocytosis in Beagles (Cooper et al. 1980) and hemolytic anemia in rats induced by increased rigidity and holes in the RBC membrane visible on electron microscopy (Akahane et al. 1986, Sengupta and Ghosh 2011). Another study observed that high fat diet-induced hyperlipidemia resulted in increased production of reactive oxygen species leading to anemia, leukocytosis, thrombocytosis, decreased oxyhemoglobin percentage, increased methemoglobin percentage, and increased RBC osmotic fragility in New Zealand White laboratory rabbits (Abdelhalim and Moussa 2010). While hyperlipidemia does not typically cause clinical anemia in dogs, it is theorized to cause changes in RBC morphology, increase osmotic fragility, and cause compensated subclinical hemolysis (Behling-Kelly and Cillins-Cronkright 2014). Because these effects are detrimental and potentially exacerbated during RBC storage, blood collection should ideally be avoided in donors exhibiting lipemia.

Figure 14.1 Lipemia is visible upon evaluation of hematocrit tubes during pre-donation screening blood work.
Various recommendations exist regarding fasting depending on the donor program. Many programs enforce a 12-hour fasting period prior to donation to limit lipemia and minimize the chances of nausea, vomiting, and aspiration-related complications during sedation. Other programs advocate feeding a healthy meal and ample water prior to donation such that the donors are well hydrated and have appropriate levels of nutrients in the bloodstream prior to blood collection. Feeding non-fat or low-fat meals on the day(s) close to the donation should be sufficient to eliminate transient occurrences of lipidemia in healthy canine donors.
Recommendations for minimizing stress
The donation process should be as positive an experience as possible for the donor. In order to reduce the stress imposed by handling, the number of instances the dog is handled by blood bank personnel should be limited or consolidated as much as possible. The physical examination and sampling of blood for pre-donation testing can be performed during a single interaction, with blood sampling occurring as soon as the physical examination is concluded.
Immediately after the physical examination is also an opportune time to apply topical anesthetic cream to the venipuncture site used for donation, as the creams typically require 30 or more minutes to fully take effect. Two commonly used topical anesthetic creams are eutectic mixture of local anesthetics (EMLA) and liposomal lidocaine. Either of these creams can be applied over the approximate area of the venipuncture site with a gauze square after clipping the fur, and stabilized with a light cling wrap or other bandage material (Figure 14.2). The ideal timing for application is just prior to sampling blood for pre-donation testing, so that the donor is only restrained once for the two procedures. Alternatively, an intradermal injection of a 10:1 mixture of 2% lidocaine and 8.4% bicarbonate at the planned venipuncture site can be used to provide local anesthesia (Figure 14.3). This method can minimize the wait time for onset of anesthesia and is typically performed with a 1 mL syringe and 25-g needle to reduce the pain associated with the injection, but this does add another injection to the procedure. The method of local anesthesia chosen should therefore be considered on a donor to donor basis.

Figure 14.2 Anesthetic cream achieves better contact during application of a light wrap.

Figure 14.3 Intradermal injection of a lidocaine/bicarbonate mixture (10:1 ratio) can be used as a local anesthetic for venipuncture during canine blood collections. (Image courtesy of Samantha Berner, LVT.)
EMLA cream (APP Pharmaceuticals, Schaumburg, IL) is a mixture of 2.5% lidocaine and 2.5% prilocaine, which is solubilized in a form that allows for local absorption without the use of solvents, providing a high concentration of the anesthetic agent to the skin without systemic absorption. Time to onset of anesthesia is 30–60 minutes after application with the use of an occlusive wrap for minor procedures, and its effectiveness in alleviating pain for percutaneous intravenous catheterization in dogs has been demonstrated (Flecknell et al. 1990). However, there are concerns regarding EMLA cream inducing methemoglobinemia in human neonates (Larson et al. 2013) and the potential for the same in dogs and cats. While no studies to date have evaluated this concern in dogs, the occurrence of methemoglobinemia in cats was not observed in a study evaluating adverse effects of EMLA during jugular catheter placement (Wagner et al. 2006). Methemoglobinemia in humans seems to be associated with inappropriate amounts, surfaces (e.g., mucous membranes), surface area, or duration of application of the cream (Gibbon et al. 2003).
Liposomal lidocaine cream (LMX 4, Ferndale Laboratories, Ferndale, MI) utilizes liposomal encapsulation of lidocaine to facilitate faster penetration of skin barriers and provides local anesthesia to the area of application (Figure 14.4). The peak onset time is 20 minutes, which is faster than EMLA cream, and the application does not require an occlusive wrap (Altman and Gildenberg 1999). A study evaluating the ability for topical liposomal lidocaine to cause systemic absorption and signs of toxicosis in cats measured plasma lidocaine concentrations well below toxic concentrations and no clinical signs of adverse effects of lidocaine such as arrhythmias or neurologic signs were seen (Fransson et al. 2002). While there is no literature documenting similar findings in dogs, the effect should be theoretically similar, making liposomal lidocaine safe for use in dogs. The shorter onset time is ideal to minimize the donor’s stay for the blood collection and is likely to be fulfilled during the pre-donation testing and preparation time.

Figure 14.4 Liposomal lidocaine cream can be used to provide topical anesthesia at the venipuncture site.
Pre-donation procedure
Preparing for donation
Gathering every piece of equipment and the supplies required to successfully perform the blood collection prior to the donor entering the room is recommended to ensure preparedness for the procedure. An ideal setup would include a room dedicated to the blood bank and donation process, containing necessary equipment and supplies. Having a designated blood collection area will allow the donor to become better habituated to the process and reduce the amount of time spent gathering items prior to donation.
Preparing materials
The supplies gathered before the donation will depend on the intended purpose of the blood collected. Several scenarios can be considered for blood donations, including small volume and standard volume whole blood collection, as well as standard volume whole blood collection for blood component processing.
Anticoagulants
Regardless of the purpose of collection, an anticoagulant is required to prevent clotting of the blood prior to transfusion of the collected blood. There are several anticoagulants available, although citrate is an anticoagulant that also provides nutrients that prolong the lifespan of RBCs during storage and is used most commonly. The storage time of whole blood and packed red blood cells (PRBCs) varies with the anticoagulant and nutrients used (Table 14.1). It is important to note that recommended storage times are based on in vitro analysis of stored RBCs and do not take into consideration the expanding knowledge of storage lesions.
Table 14.1 Canine red cell storage duration based on anticoagulant and nutrient solutions used
Anticoagulant | Additive nutrient solution | Shelf life (at 4°C) | |
PRBCs | Whole blood | ||
CPD | SAGM | 42 days | – |
CPD | AS-1 or AS-5 | 35 days | – |
CP2D | AS-3 | 35 days | – |
CPDA-1 | – | – | 28 days |
CPD | – | – | 21 daysa |
ACD | – | – | 21 daysa |
Heparin | – | – | Immediate transfusion |
ACD, acid citrate dextrose; CP2D, citrate phosphate double dextrose; CPD, citrate phosphate dextrose; CPDA-1, citrate phosphate dextrose adenine-1; SAGM, sodium adenine glucose mannitol; AS-1, Adsol®; AS-3, Nutricel®; AS-5, Optisol®.
a Based on human data (no canine data available). Note: These storage times are based on in vitro analysis and do not consider the effects of storage lesions.
Heparin
Unfractionated (regular) heparin is readily available in many veterinary practices and can be used as an anticoagulant for blood collected for immediate transfusion. Blood should not be stored using heparin as an anticoagulant since it is not an RBC preservative. Additionally, the multiple-use nature of heparin bottles in veterinary hospitals and the necessity of using an open collection system in which the sterility cannot be maintained limit the storage time when this anticoagulant is used. Each milliliter of blood collected should have 5–12.5 IU of heparin added for proper anticoagulation (Abrams-Ogg 2000).
CPDA-1, CPD, and CP2D
Citrate-phosphate-dextrose-adenine-1 (CPDA-1) is a citrate-based anticoagulant preservative solution. Citrate functions by chelating calcium to limit its availability as a co-factor in the coagulation process. The addition of phosphate alters the pH to promote RBC survival and serves as a substrate for 2,3-diphosphoglycerate (2,3-DPG) production. Dextrose provides glucose molecules to be used for glycolysis and adenosine triphosphate (ATP) production as a source of energy. Adenine serves as a substrate for ATP production. Each of these nutrients is important for RBCs to perform cell maintenance processes, including the maintenance of cell membrane integrity, as well as minimizing impairment of stored hemoglobin as an oxygen carrier.
Citrate-phosphate-dextrose (CPD) and citrate-phosphate-double-dextrose (CP2D) are other citrate-based anticoagulants coupled with additional nutrient solutions that prolong RBC survival after processing into PRBCs. CPDA-1, CPD, and CP2D are used at a ratio of 1 mL for each 7 mL of blood collected. Whole blood can be stored at 4°C with CPDA-1 up to a maximum of 28 days and PRBCs can be stored with CPD and CP2D with additive solution combinations for 35 days (Wardrop et al. 1994; Lucas et al. 2004; Gibson and Abrams-Ogg 2012). Blood collection systems containing CPDA-1, CPD, or CP2D are supplied with appropriate anticoagulant to blood volume ratio for standard collection volumes.
ACD
Acid-citrate-dextrose (ACD) is another citrate-based anticoagulant preservative solution that can be used for blood collected and intended for storage prior to transfusion. While not commonly used in the United States in comparison to CPD and CPDA-1, in other parts of the world ACD is available from suppliers in a large volume (multiple-dose) container, allowing easier use when smaller, non-standard volumes of blood are collected. When ACD is used, 1 mL should be mixed to 7–9 mL of collected blood for sufficient anticoagulation. Evidence regarding the storage duration of canine whole blood when ACD is used is not available, although human data indicates viability for 21 days when stored at 4°C.
Nutrient solutions
Various nutrient additive solutions have been developed in the pursuit of longer RBC shelf lives, such that these solutions are sometimes referred to as “red cell life extenders”. Commonly available nutrient solutions include Adsol® (AS-1) (Fenwal Inc., Lake Zurich, IL), Nutricel® (AS-3) (Haemonetics Corporation, Braintree, MA), Optisol® (AS-5) (Terumo Corporation, Tokyo, Japan), and sodium-adenine-glucose-mannitol (SAGM). The addition of these solutions to PRBCs after plasma separation improves the storage time to 35 days for AS-1, AS-3, and AS-5 (Lucas 2004) and 42 days for SAGM (Ergül Ekiz et al. 2012). These solutions are provided as a part of a closed collection system with pre-filled chambers, ready to be mixed without breaking sterility.
Collection systems
Small volume whole blood collection
Rarely, a small volume transfusion of whole blood might be collected for immediate transfusion to a patient. Such a situation is typically limited to small dogs and neonates that can benefit from a transfusion of fresh whole blood containing RBCs, coagulation factors, and platelets. The collection system required in these cases comprises one or more syringes to collect the total volume of blood desired, anticoagulant at an appropriate ratio to keep the desired volume of blood from clotting, and a butterfly catheter to perform the venipuncture. After successful venipuncture, the syringe is rotated gently to mix the blood with the anticoagulant as it is drawn into the syringe. This is considered an open system that is more prone to bacterial contamination. While there is no veterinary data available for viable storage time of RBCs in plastic syringes, a human study observed no significant changes to blood stored in plastic syringes for 24 hours at 4°C (Podlosky et al. 2008).
Standard volume whole blood collection
Whole blood collection sets consisting of a single plastic bag with tubing connected to a large bore needle are available from commercial suppliers. Bags of 250 mL and 450 mL collection volume are commonly used (Figure 14.5). The bags are pre-filled with anticoagulant at a ratio appropriate for the designated collection volume (63 mL CPDA-1 for 450 mL collection bags). These bags are considered a closed collection system, thus minimizing the chance of bacterial contamination during collection. After blood collection, the tubing is sealed, leaving the bag with ports available for a blood administration set to be inserted.

Figure 14.5 250 mL (left) and 450 mL (right) whole blood collection bags.
Standard volume whole blood collection for component processing
Blood is more commonly collected for component processing utilizing closed collection systems with satellite bags (Figure 14.6). This allows for component therapy and to optimize storage conditions for each component. The primary collection bag contains a citrate-based anticoagulant (63 mL CPD) designed to anticoagulate 450 mL whole blood. The satellite bags are welded onto tubing exiting the primary collection bag, with a temporary tube seal that can be broken upon processing of the blood (see Chapter 17).

Figure 14.6 A multiple-bag blood collection set designed for blood component processing.
Additional equipment and supplies
Supplies required for blood banking aside from the blood collection system are those that involve aseptic collection of blood via venipuncture and intravenous fluid administration. Blood bank specific instruments and equipment such as blood tube strippers, blood tube clamps, a blood tube sealer, and a gram scale are also required. A vacuum chamber and suction machine, and blood collection mixers are optional equipment used during blood collection. A complete list of supplies and equipment required can be found in Box 14.1 and other details regarding specialized equipment are explained throughout the rest of the chapter. Additional information regarding specific items for blood banking can be found in Chapter 17.

Full access? Get Clinical Tree
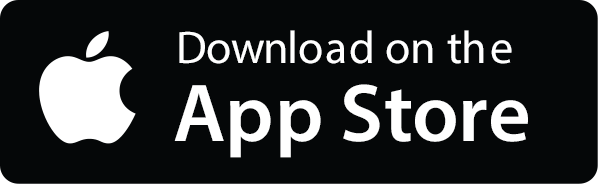
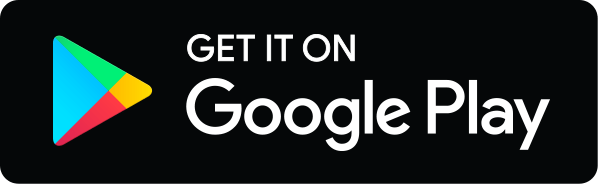