Bunyaviridae
Abstract
This chapter describes the properties of bunyaviruses and features of the diseases they cause in animals.
Keywords
Bunyaviridae; Bunyavirus; arthopod vectors; congenital defects
The family Bunyaviridae is the largest virus family, with more than 350 member viruses included in 5 genera: Orthobunyavirus, Hantavirus, Nairovirus, Phlebovirus, and Tospovirus. The family name is derived from Bunyamwera in western Uganda, where the prototype bunyavirus of the same name was isolated. The common features of the bunyaviruses pertain both to the nature of the virions and to their biological properties. Viruses in three genera (Orthobunyavirus, Nairovirus, and Phlebovirus) are maintained in arthropod–vertebrate–arthropod cycles (ie, arboviruses), which have specificity in regard to both arthropod vectors and vertebrate reservoir hosts. This specificity is the basis for the usually narrow geographic and ecologic niches occupied by each virus. Similarly, viruses in the genus Tospovirus can be transmitted between plants by thrips, and replicate in both thrips and plants. Viruses in the genus Hantavirus are an exception, in that they are maintained in vertebrate–vertebrate cycles without arthropod vectors; nevertheless, the hantaviruses also exhibit great specificity in vertebrate reservoir hosts, and therefore also have distinct geographic and ecologic niches (Table 22.1).
Table 22.1
Family Bunyaviridae: Major Pathogens of Animals and Humans
Genus | Virus | Geographic Distribution | Arthropod Vector | Target Host Species or Amplifier Host | Disease in Animals | Disease in Humans |
Phlebovirus | Rift Valley fever virus | Africa | Mosquitoes | Sheep, cattle, buffalo, humans | Systemic disease, hepatitis, abortion | Flu-like illness, hepatitis, hemorrhagic fever, retinitis |
Nairovirus | Nairobi sheep disease virus | Eastern Africa | Ticks | Sheep, goats | Hemorrhagic enteritis | Mild febrile illness |
Crimean-Congo hemorrhagic fever virus | Africa, Asia, Europe | Ticks | Sheep, cattle, goats, humans | Mild if any | Hemorrhagic fever, hepatitis | |
Bunyavirus | Akabane virus | Australia, Japan, Israel, Korea, Africa | Mosquitoes, Culicoides spp. | Cattle, sheep, goats | Arthrogryposis, hydranencephaly | None |
Schmallenberg virus | Europe (including United Kingdom, Turkey) | Culicoides spp. | Cattle, sheep, goats, wild ruminants, camelids | Arthrogryposis, hydranencephaly | None | |
Cache Valley virus | United States | Mosquitoes | Cattle, sheep, goats, deer, horses | Arthrogryposis, hydranencephaly rarely | Sporadic encephalitis and rare congenital infection | |
La Crosse and other California encephalitis group viruses | North America | Mosquitoes | Small mammals, humans | None | Encephalitis | |
Hantavirus | Hantaan virus | China, Russia, Korea | None | Apodemus agrarius (striped field mouse) | None documented | Hemorrhagic fever with renal syndrome |
Puumala virus | Scandinavia, Europe, Russia | None | Clethrionomys glareolus (bank vole) | None documented | Hemorrhagic fever with renal syndrome | |
Seoul virus | Worldwide | None | Rattus norvegicus (Norway rat) | None documented | Hemorrhagic fever with renal syndrome | |
Sin Nombre virus and other New World hantaviruses | The Americas | None | Peromyscus maniculatus (deer mouse) and other reservoir rodent species | None documented | Hantavirus pulmonary syndrome |
Arthropod-borne bunyaviruses are transmitted by specific mosquitoes, ticks, midges, or biting flies, whereas the individual hantaviruses are disseminated by specific rodents. Bunyaviruses cause transient infection in their vertebrate hosts, whether mammal or bird, and life-long persistent infection in their arthropod vectors, whereas hantaviruses cause persistent infection in their rodent reservoir hosts. Most bunyaviruses never infect domestic animals or humans, but those that do can cause important diseases that vary from congenital fetal malformation to systemic “hemorrhagic fever” disease syndromes.
Properties of BUNYAVIRUSES
Classification
The very large number and diversity of the bunyaviruses offer a considerable taxonomic challenge, and current nomenclature is confusing. Genomic features are used to define genera, particularly the organization of each RNA genome segment and the sequences of conserved nucleotides at the termini of each segment. Classical serologic methods are used to classify these viruses further. In general, antigenic determinants on the nucleocapsid protein are relatively conserved, and so serve to define broad groupings among the viruses, whereas shared epitopes on the envelope glycoproteins, which are the targets in neutralization and hemagglutination-inhibition assays, define narrow groupings (serogroups). Unique epitopes on envelope glycoproteins, also determined by neutralization assays, define individual virus species. With few exceptions, viruses within a given genus are related antigenically to each other, but not to viruses in other genera. The lack of adequate biochemical characterization of many named bunyaviruses confuses their precise classification.
Genetic reassortment occurs when mammalian hosts, insect vectors, or cultured cells are coinfected with closely related bunyaviruses, and this probably has been important in the natural evolution of these viruses. Within its particular ecologic niche, each bunyavirus evolves by genetic drift and selection; for example, isolates of La Crosse virus from different regions in the United States differ considerably, as a result of cumulative point mutations and nucleotide deletions and duplications. The evolution of La Crosse virus has also involved genome segment reassortment, and reassortant viruses have been isolated from mosquitoes in the field.
The bunyaviruses are assigned to five genera, four of which include viruses that infect animals and a fifth (the genus Tospovirus) that contains only plant viruses. A very substantial number of bunyaviruses have not yet been assigned to a genus or serogroup.
The genus Orthobunyavirus contains a large number of viruses that share common genetic features and are serologically unrelated to viruses in other genera of the Bunyaviridae. Most of these viruses are mosquito-borne, but some are transmitted by sandflies or midges (Culicoides spp.). The genus includes a number of pathogens of domestic animals and humans, including Akabane and La Crosse viruses and their relatives.
The genus Phlebovirus contains over 50 viruses, all of which are transmitted by sandflies or mosquitoes. The genus contains important pathogens, including Rift Valley fever virus and the sandfly fever viruses.
The genus Nairovirus contains a large number of viruses, most of which are tick-borne, including the pathogens Nairobi sheep disease and Crimean–Congo hemorrhagic fever viruses.
The genus Hantavirus also includes a substantial number of viruses, many of them relatively recently discovered. All are transmitted by persistently infected reservoir rodent hosts via urine, feces, and saliva; the same transmission pattern has occurred among rats in laboratory colonies. In humans, several of these viruses from Asia cause hemorrhagic fever with renal syndrome, whereas those from Europe are typically associated with a different and less severe disease syndrome. Some of the hantaviruses from the Americas cause a severe acute respiratory distress syndrome referred to as “hantavirus pulmonary syndrome.”
Virion Properties
Morphological properties vary among viruses in the various genera, but bunyavirus virions are spherical, approximately 80–120 nm in diameter, and are composed of a lipid envelope with glycoprotein spikes, inside which are three circular ribonucleoprotein (RNP) complexes comprised of individual genome RNA segments associated with the viral nucleoprotein (Table 22.2; Fig. 22.1). These RNP complexes are stabilized by a panhandle structure generated by noncovalent bonds between inverted palindromic sequences on the 3′ and 5′ ends of each RNA genome segment. The terminal sequences are identical for all three RNA segments within each virus species, and are critical for recognition by the viral polymerase for virus genome replication and initiation of virus mRNA transcription.
Table 22.2
Four genera infect vertebrates: Bunyavirus, Phlebovirus, and Nairovirus, all arthropod-borne; Hantavirus, nonarthropod-borne
Virions are spherical, enveloped, 80–120 nm in diameter
Virions have glycoprotein spikes but no matrix protein in their envelope
Segmented negative-sense, single-stranded RNA genome; three segments—L (large), M (medium), and S (small)—total 11–19 kb in size
The S segment of the genomic RNA of the member viruses of the genus Phlebovirus has an ambisense coding strategy
Cytoplasmic replication; budding into Golgi vesicles
Generally cytocidal for vertebrate cells, but noncytocidal persistent infection in invertebrate cells
Genetic reassortment occurs between closely related viruses
The genome of bunyaviruses is 11–19 kb and consists of three segments of negative-sense (or ambisense), single-stranded RNA, designated large (L), medium (M), and small (S). The RNA segments differ in size among the genera: the L RNA segment ranges in size from 6.3 to 12 kb, the M RNA segment from 3.5 to 6 kb, and the S RNA segment from 1 to 2.2 kb. The L RNA encodes a single large protein (L), the RNA-dependent RNA polymerase. The M RNA encodes a polyprotein that is processed to form two glycoproteins (Gn and Gc) and, in some cases, a nonstructural protein (NSm). The S RNA encodes the nucleoprotein (N) and, for members of the Orthobunyavirus and Phlebovirus genera, a nonstructural (NSs) protein (Fig. 22.2). The N and NSs proteins of viruses in the genus Phlebovirus are each translated from a separate subgenomic mRNA. The N protein is encoded in the 3′-half of the S RNA, and its messenger RNA (mRNA) is transcribed using genomic RNA as template. However, the NSs protein, occupying the 5′-half of the same S RNA molecule, is encoded in the reverse complementary sense, with the NSs mRNA being transcribed only after the synthesis of full-length viral genome RNA intermediates; thus the S segment RNA exhibits an ambisense coding strategy.
All bunyaviruses have at least four virion proteins (Fig. 22.1), including two external glycoproteins (Gn, Gc), the L protein (transcriptase), and the N protein (nucleoprotein). Virions also contain lipids, with their composition reflecting the composition of host-cell membranes (principally derived from the Golgi membrane, but also cell-surface membrane) and carbohydrates as side chains on the glycoproteins. The Gn glycoprotein (formerly known as G2) is responsible for receptor binding of California serogroups bunyaviruses. The nonstructural NSs protein of Rift Valley fever virus interferes with the innate host-cell antiviral response at multiple levels, leading to global suppression of the type I interferon response (see Chapter 4: Antiviral Immunity and Virus Vaccines).
The viruses are quite sensitive to heat and acid conditions, and are inactivated readily by detergents, lipid solvents, and common disinfectants.
Virus Replication
Most bunyaviruses replicate well in many kinds of cells, including Vero (African green monkey) cells, BHK-21 (baby hamster kidney) cells, and, other than hantaviruses, C6/36 mosquito (Aedes albopictus) cells. With the exception of hantaviruses and some nairoviruses, these viruses are cytolytic for mammalian cells, but are noncytolytic for invertebrate cells. Most of the viruses also replicate to high titer in suckling mouse brain.
Viral entry into its host cell is by receptor-mediated endocytosis; all subsequent steps take place in the cytoplasm. Cell receptors are not described for many bunyaviruses, but those that contribute to binding of the hantaviruses include αβ integrins and other cell receptor proteins such as gC1qR/p32, which is expressed on endothelial cells, dendritic cells, lymphocytes, and platelets. Because the genome of the single-stranded, negative-sense RNA viruses cannot be translated directly, the first step after penetration of the host cell and uncoating is the activation of the virion RNA polymerase (transcriptase) and its transcription of viral mRNAs from each of the three virion RNAs. The exception, as noted earlier, is that in the genus Phlebovirus the 5′-half of the S RNA is not transcribed directly; instead, the mRNA for the NSs protein is transcribed after synthesis of full-length complementary RNA. The RNA polymerase also has endonuclease activity, cleaving 5′-methylated caps from host mRNAs and adding these to viral mRNAs to prime transcription (so-called cap snatching). After primary viral mRNA transcription and translation, replication of the virion RNA occurs and a second round of transcription begins, with preferential amplification of the genes that encode structural proteins necessary for virion synthesis.
Virions mature by budding through intracytoplasmic vesicles associated with the Golgi complex and are released by the transport of vesicles through the cytoplasm and subsequent exocytosis from the apical and/or basolateral plasma membranes (Table 22.2).
Members of the Genus Hantavirus
The more than 20 member viruses of the genus Hantavirus comprise the only viruses in the family Bunyaviridae that are not arthropod-borne. They are transmitted among rodents by long-term shedding in saliva, urine, and feces. Several of the viruses are zoonotic and the cause of severe human disease. Although there is overlap in the respective disease syndromes, four Old World (Asia and Europe) hantaviruses cause multisystem disease centered on the kidneys (hemorrhagic fever with renal syndrome), whereas several New World (the Americas) hantaviruses cause multisystem disease centered on the lungs (hantavirus pulmonary syndrome). The pathogenicity of some of the newly discovered viruses has not yet been determined. Some viruses also infect other mammalian species, such as horses, but this is uncommon and does not contribute to the life cycle of the viruses or to the risk of human disease.
One important aspect of the hantaviruses has been the level of difficulty surrounding their discovery. For example, during the Korean war of 1950–1952, thousands of United Nations’ troops developed a disease marked by fever, headache, hemorrhagic manifestations, and acute renal failure, with shock and substantial mortality of 5–10%. Despite intense research, the etiologic agent of this disease remained a mystery for 28 years until the prototype hantavirus, Hantaan virus (the name is derived from the Hantaan River in Korea), was isolated from the striped field mouse, Apodemus agrarius. Hantaviruses discovered since then have also been so difficult to isolate in cell culture or experimental animals that RT-PCR assays, and now next generation sequencing strategies (metagenomics) have become key tools for detection and characterization of these viruses in clinical specimens and rodent tissues. More than 200,000 cases of hemorrhagic fever with renal syndrome are reported each year throughout the world, with more than half in China. Russia and Korea report hundreds to thousands of cases; fewer are reported from Japan, Finland, Sweden, Bulgaria, Greece, Hungary, France, and the Balkan countries.
In 1993, a previously unrecognized zoonotic hantavirus disease was recognized in the southwestern region of the United States. The disease was manifest, not as hemorrhagic fever with renal syndrome, but rather as an acute respiratory distress syndrome. The causative virus is now called Sin Nombre virus, and at least eight other hantaviruses have since been identified as causes of the pulmonary syndrome, including Bayou, Black Creek Canal, Andes, and Laguna Negra viruses. Cases of hantavirus pulmonary syndrome have been reported across the United States, and from Canada to Argentina. Other New World hantaviruses have been discovered recently, but their pathogenicity in humans has not yet been determined.
HEMORRHAGIC FEVER WITH RENAL SYNDROME (OLD WORLD) HANTAVIRUSES
The hallmark of hantavirus infection in rodent reservoir hosts is persistent, usually lifelong, inapparent infection with virus shedding in saliva, urine, and feces. Human disease involves contact with contaminated rodent excreta, usually in winter when there is maximal human–rodent contact. In a landmark pathogenesis study, H.W. Lee inoculated the reservoir rodent, A. agrarius, with Hantaan virus and followed the course of infection by virus titration of organs, serology, and immunofluorescence. Viremia was transient and disappeared as neutralizing antibodies appeared. However, virus persisted in several organs, including lungs and kidneys. Virus titers in urine and throat swabs were about 100–1000 times higher during the first weeks after inoculation than subsequently, and animals were much more infectious for cage mates or nearby mice during this period.
Four essential features define the global context of the disease hemorrhagic fever with renal syndrome (Table 22.1): (1) the viruses, per se; (2) their reservoir rodent hosts; (3) the locale of human cases; (4) the severity of human cases. At least four viruses are involved: Hantaan, Dobrava-Belgrade, Seoul, and Puumala viruses. Typically, the Hantaan and Dobrava-Belgrade viruses cause severe disease, with mortality rates of 5–15%. Seoul virus causes less severe disease, and Puumala virus causes the least severe form of the disease (mortality rate less than 1%), which is known in Scandinavia as “nephropathia epidemica.” There are three disease locale patterns: rural, urban, and laboratory acquired. Rural disease is caused by Hantaan virus, which is widespread in China, Asian Russia, and Korea, and Dobrava-Belgrade virus in the Balkans and Greece. Rural disease is also caused by Puumala virus in northern Europe, especially in Scandinavia and Russia. Urban disease is caused by Seoul virus; it occurs in Japan, Korea, China, and South and North America. Each virus has a specific reservoir rodent host. For Hantaan virus this is the striped field mouse, Apodemus agrarius; for Dobrava-Belgrade virus it is the yellow-neck mouse, Apodemus flavicollis; for Seoul virus it is the Norway rat, Rattus norvegicus; and for Puumala virus it is the bank vole, Clethrionomys glareolus.
There is no evidence that there is any clinical disease in animals other than humans. The clinical course of severe hemorrhagic fever with renal syndrome in humans involves five overlapping stages—febrile, hypotensive, oliguric, diuretic, and convalescent—not all of which are seen in every case. The onset of the disease is sudden, with intense headache, backache, fever, and chills. Hemorrhage, if it occurs, is manifested during the febrile stage as a flushing of the face and injection of the conjunctiva and mucous membranes. A petechial rash may also appear. Sudden and extreme hypotension from vascular leakage can precipitate fatal hypovolemic shock, and patients who survive and progress to the diuretic stage show improved renal function but may still die of shock or pulmonary complications. The convalescent stage can last for weeks to months before recovery is complete. The disease in affected individuals is believed to have an immunopathologic basis, as virus-specific antibodies are present, usually from the first day that the patient presents for medical care.
Diagnosis is made by the detection of antigen in tissues (usually by immunofluorescence) or serologically by IgG and IgM capture ELISA. The IgM capture ELISA is used as a primary diagnostic tool, but RT-PCR assays are now available for virus detection. Virus isolation is difficult: the viruses must be blind-passaged in cell culture (most commonly Vero E6 cells) and detected by immunologic or molecular means. Shipping of diagnostic specimens from sites of disease is often less than satisfactory, and all laboratory work on Hantaan and other highly pathogenic hantaviruses must be done under strict biocontainment conditions.
Several inactivated Hantaan virus vaccines have been developed and used in Asia. The key to preventing hantavirus infections is rodent control, including making homes and food stores rodent proof and removing dead rodents and rodent droppings from human habitations. However, control of the wide-ranging rodent reservoirs of Hantaan, Seoul, and Puumala viruses is not possible in most settings in which disease occurs; nevertheless, in some situations their entry into dwellings can be minimized.
There have been several instances in which wild rodents brought into laboratories, established colonies of laboratory rats, and even cell cultures derived from rats have carried or been contaminated with hantaviruses, and have led to virus transmission to animal caretakers and research personnel. Prevention of introduction of virus into laboratory rat colonies requires quarantined entry of new stock (or entry only of known virus-free stock), prevention of access by wild rodents, and regular serologic testing.
HANTAVIRUS PULMONARY SYNDROME (NEW WORLD) HANTAVIRUSES
Sin Nombre virus and other New World hantaviruses probably have been present for eons in extensive portions of the Americas inhabited by Peromyscus maniculatus and other reservoir rodent species; these viruses were recognized in 1993 only because of the number and clustering of human cases of a very distinctive disease syndrome in the western United States. A great increase in rodent numbers after two especially wet winters and a consequent increase in pinyon seeds and other rodent food contributed to the number of human cases. The temporal distribution of human disease reflects a spring–summer seasonality (although cases have occurred throughout the year), again matching the behavior patterns of rodent reservoir hosts. Just as with Old World hantaviruses, each New World virus has a specific reservoir rodent host: Sin Nombre virus—the deer mouse, Peromyscus maniculatus; New York virus—the white-footed mouse, Peromyscus leucopus; Black Creek Canal virus—the cotton rat, Sigmodon hispidus; Bayou virus—the rice rat, Oryzomys palustris; and Andes virus—the long-tailed pygmy rice rat, Oligoryzomys longicaudatus.
Like the hantaviruses that cause hemorrhagic fever with renal syndrome, those that induce hantavirus pulmonary syndrome do not cause disease in their rodent reservoir hosts, but can induce severe disease in infected humans. The virus is shed in saliva, urine, and feces of rodents for at least many weeks, and probably the lifetime of the animal. Transmission from rodent to rodent occurs by close contact and from bite or scratch wounds. Transmission and human infection probably also occur by the inhalation of aerosols or dust containing infected dried rodent saliva or excreta.
Hantavirus pulmonary syndrome in humans typically starts with fever, myalgia, headache, nausea, vomiting, nonproductive cough, and shortness of breath. As disease progresses, there is pulmonary edema, pleural effusion, and rapid disease progression, with death often following in hours to days. Recovery can be as rapid as the development of life-threatening clinical signs. Lesions are those of the acute respiratory distress syndrome, with pulmonary congestion and edema and interstitial pneumonia. Person-to-person transmission appears to be rare, but has been confirmed in cases of Andes virus infection, and strict barrier nursing techniques are now recommended for management of suspected cases.
Functional impairment of vascular endothelial integrity with increased capillary permeability, especially of the pulmonary vasculature, and subsequent hypovolemia, is central to the pathogenesis of hantavirus-induced pulmonary syndrome. However, although there is widespread hantavirus infection of endothelial cells in affected individuals, hantaviruses do not lyse infected cells. It is uncertain what causes this severe, often fatal increase in capillary permeability that results ultimately in hypovolemic shock, but virus-induced cytokine mediators probably contribute (so-called cytokine storm).
The clinical and hematologic findings of hantavirus pulmonary syndrome are characteristic, but the specific diagnosis is usually made by demonstrating antibodies to the infecting virus or one of its close relatives (typically using IgM capture ELISA). Isolation of the virus from patients is very difficult, but viral nucleic acid is readily detected by RT-PCR. Serological testing is usually performed to evaluate infection in reservoir rodent hosts, because of the biohazard associated with virus isolation.
Extensive public education programs have been developed to advise people about reducing the risk of infection, mostly by reducing rodent habitats and food supplies in and near homes, and by taking precautions when cleaning rodent-contaminated areas. The latter involves rodent-proofing food and pet food containers, trapping and poisoning rodents in and around dwellings, eliminating rodent habitats near dwellings, use of respirators, and wetting down surfaces with detergent, disinfectant, or hypochlorite solution before cleaning areas that may contain rodent excreta. Recommendations have also been developed for specific equipment and practices to reduce risks when working with wild-caught rodents, especially when this involves obtaining tissue or blood specimens: these include use of live-capture traps, protective clothing and gloves, suitable disinfectants, and safe transport packaging.
Members of the Genus Nairovirus
NAIROBI SHEEP DISEASE VIRUS
Nairobi sheep disease virus, a member of the genus Nairovirus, is highly pathogenic for sheep and goats. The virus is enzootic in eastern Africa, and closely related viruses occur in Nigeria (Dugbe virus in cattle), and in India and Sri Lanka (Ganjam virus in sheep and goats). The virus is not contagious among mammals; rather, it is transmitted by all stages of the brown ear tick, Rhipicephalus appendiculatus, in which there is transovarial and trans-stadial infection and very long-term carriage in adult ticks (up to 2 years). The vertebrate reservoir host of the virus remains unknown; the virus has not been found in wild ruminants or other animals in enzootic areas.
In Kenya, sheep and goats acquire the infection when they are transported from northern districts to the Nairobi area. After a short incubation period, there is high fever, hemorrhagic enteritis, and prostration. Affected animals may die within a few days, and pregnant ewes abort. Mortality in sheep is up to 90%. Subclinical infections also occur, and recovered animals are immune. Diagnosis is made clinically and by gross pathologic examination; it may be confirmed by virus isolation and identification of isolates immunologically, or by simple immunodiffusion tests on tissue extracts utilizing hyperimmune virus-specific antisera. Control depends primarily on acaricidal treatments to control the vector tick, which is also the vector of the economically important protozoan disease, East Coast fever. Both live-attenuated and inactivated vaccines are effective in preventing the disease in sheep.
The virus is not considered to be a significant human pathogen, although it was isolated from a person with a mild febrile disease.
CRIMEAN-CONGO HEMORRHAGIC FEVER VIRUS
Crimean-Congo hemorrhagic fever virus, a member of the genus Nairovirus, is the cause of an important zoonotic disease (Crimean-Congo hemorrhagic fever) that has been recognized for many years in central Asia and Eastern Europe. There is no evidence of clinical disease in animals other than humans, but the infection in domestic animals is the basis for the overall importance of this disease. This virus is now known to be enzootic from western China, through Central Asia to India, Pakistan, Afghanistan, Iran, Iraq, Turkey, Greece, and other countries of the Middle East, Eastern Europe, and most of Saharan and sub-Saharan Africa. In recent years, there have been repeated outbreaks of the disease in Turkey and the countries of the Persian Gulf, especially in connection with traditional sheep slaughtering and butchering practices. Crimean-Congo hemorrhagic fever is an emerging problem, with increasingly more cases being reported each year from many parts of the world, and increasing detection of antibody in animal populations. For example, in serosurveys, more than 8% of cattle in several regions of Africa have been shown to be seropositive.
The virus is maintained by a cycle involving transovarial and trans-stadial transmission in Hyalomma spp. and many related ticks. Larval and nymphal ticks become infected when feeding on small mammals and ground-dwelling birds, and adult ticks when feeding on wild and domestic ruminants (sheep, goats, and cattle). Infection in wild and domestic ruminants results in high-titer viremia that is sufficient to infect feeding ticks.
The disease in humans is a severe hemorrhagic fever. The incubation period is 3–7 days; onset is abrupt, with fever, severe headache, myalgia, back and abdominal pain, nausea and vomiting, and marked prostration. It is one of the most dramatic of all human hemorrhagic fevers, characterized by substantial hemorrhage within the subcutis and at mucosal surfaces lining the gastrointestinal and genitourinary tracts. There is also marked liver necrosis, and injury to both the myocardium and central nervous system; the case-fatality rate is commonly 15–40%. The disease affects primarily farmers, veterinarians, slaughterhouse workers, butchers, and others coming in contact with livestock, forest-workers, and others coming in contact with infected ticks. The virus is also transmitted by direct contact with subclinically infected viremic animals—for example, during sheep docking, shearing, anthelminthic administration, and veterinary procedures. The virus is also contagious, and can be transmitted from human to human, especially in hospitals.
Diagnosis is made by the detection of antigen in tissues (usually by immunofluorescence) or IgM antibody (by capture ELISA). Real-time RT-PCR assays are now standard for Crimean-Congo hemorrhagic fever virus detection. Virus isolation has proven difficult: the virus is very labile, shipping of diagnostic specimens from usual sites of disease is often less than satisfactory, and all laboratory work must be performed under maximum containment conditions.
Inactivated vaccines have been developed and used in small-scale trials, but are generally not available. Prevention based on vector control is difficult because of the large areas of wooded and brushy tick habitat involved. One important prevention approach would be the enforcement, in endemic areas of Asia, the Middle East, and Africa, of occupational safety standards on farms and in livestock markets, abattoirs, and other workplaces where there is routine contact with sheep, goats, and cattle. Individual risk can be minimized in people living in endemic zones by avoiding tick bites through inspection of clothing and application of repellents.
Members of the Genus Orthobunyavirus
AKABANE VIRUS
Akabane virus is best known for its teratogenic effects in ruminants, with seasonal epizootics of reproductive loss (embryonic/fetal mortality, abortion) and congenital arthrogryposis and hydranencephaly being well described in cattle in Australia, Japan, and Israel. The virus can cause similar reproductive losses and developmental defects in sheep and goats. Akabane virus and/or closely related viruses occur throughout much of Africa and Asia, in addition to Australia.
Clinical Features and Epidemiology
Akabane virus infection of nonpregnant ruminants typically is subclinical, but some strains of Akabane virus in Japan and Korea may cause acute encephalitis in cattle of any age. Infection of pregnant cattle or sheep can lead to one of two outcomes: death of the fetus and abortion, or birth, sometimes premature, of progeny with congenital defects. Affected fetuses characteristically have extensive cavitary defects of the central nervous system (hydranencephaly) and severe musculoskeletal abnormalities (arthrogryposis), thus abortion or birth is often accompanied by dystocia. Fetuses born with hydranencephaly usually are unable to stand after birth; those less severely affected may manifest marked incoordination and a variety of other neurologic deficits (“dummy calves”).
Although the vectors of Akabane virus remain to be conclusively proven, it is believed that the virus is transmitted in Japan by Aedes spp. and Culex spp. mosquitoes, and in Australia by the hematophagous midge, Culicoides brevitarsis. As Akabane virus is an arthropod-borne virus infection, its transmission is seasonal. The type and severity of clinical signs reflect the stage of gestation at which fetal infection took place, and in herds practicing year-round calving, the entire spectrum of lesions and outcomes can be observed.
Pathogenesis and Pathology
After the bite of an infected insect, the virus infects the pregnant ruminant (cow, goat, or sheep) usually without producing clinical signs, and reaches the fetus from the maternal circulation. Fetal infection results in both encephalomyelitis and polymyositis, and virus replication within the developing central nervous system leads to destruction of the developing brain and subsequent hydranencephaly. In general, the earlier the virus reaches the developing cerebrum, the worse the teratogenic defect; in severe cases there is complete absence of the cerebral hemispheres, which are replaced by fluid-filled sacs. The most severe fetal lesions in cattle result from infection at 3–4 months of gestation. Arthrogryposis, the other highly characteristic manifestation of fetal infection with Akabane virus, is characterized by muscular atrophy and the abnormal fixation of several limbs, usually in flexion. In cattle, arthrogryposis is the outcome of infection between 4 and 6 months of gestation. Severely affected fetuses usually die and are aborted, whereas those alive at birth often must be euthanized. In sheep and goats, due to the shorter period of gestation, the sequential occurrence of hydranencephaly and arthrogryposis as observed in cattle does not occur. Affected lambs or kids are frequently born with multiple severe lesions in the brain and musculature, and may have hypoplasia of the lungs or other organs.
The histological lesions observed also vary with the time of gestation at which infection occurs. Calves infected late in gestation have a nonsuppurative polioencephalomyelitis, often associated with a flaccid paralysis at birth. Animals affected with congenital arthrogryposis have few gross abnormalities, other than limited or no movement of their joints. Microscopically, there are severe degenerative changes in the motor horns of the spinal cord and there may be degenerative changes in the skeletal muscle.
Lesions associated with hydranencephaly can vary from small cystic lesions to the virtual absence of the cerebral hemispheres and replacement with fluid filled meningeal sacs. Histologic evaluation of the tissues of animals with severe hydranencephaly-arthrogryposis is often unrewarding as the cavitary lesions in affected brain are typically surrounded by parenchyma with relatively normal architecture. In cattle, the cerebellum is rarely, if ever, affected, a useful differential feature to distinguish from other congenital infections such as bovine viral diarrhea virus, however cerebellar hypoplasia/atrophy does occur in some calves congenitally infected with other orthobunyaviruses such as Aino and Schmallenberg viruses.
Diagnosis
In enzootic areas, diagnosis of Akabane virus infection may be suggested by clinical, pathologic, and epidemiologic observations (seasonal occurrence), but most often by gross pathologic examination. Diagnosis is confirmed by the detection of specific antibodies in serum or fluids (pleural, pericardial) collected from aborted fetuses or from newborn calves, kids, or lambs before ingestion of colostrum. Alternatively, virus specific RT-PCR may be used to detect viral RNA in samples from malformed fetuses, especially from the central nervous system or in the serum of acutely infected animals, although viremia only lasts for a few days. Virus is difficult or impossible to isolate after congenitally infected calves, kids, or lambs are born. However, virus can be recovered from the placenta, brain or muscle of aborted fetuses, or from tissue samples taken from fetuses removed by cesarean section before normal parturition or after slaughter of the dam. Virus isolation is carried out in cell cultures or by intracerebral inoculation of suckling mice. Residual viral RNA may be detected from a range of organs by RT-PCR.
Immunity, Prevention, and Control
Infection with Akabane virus induces lasting immunity, and outbreaks typically are seen on the margins of endemic regions as the distribution of vector insects tends to fluctuate under the influence of climatic variation. Naïve animals imported into endemic regions are also at risk. Both inactivated and live-attenuated vaccines are available for protective immunization of livestock against Akabane virus infection. Inactivated virus vaccines produced in cell culture have proven safe and efficacious, and are also used as combination vaccines against other viral infections (eg, Aino virus).
SCHMALLENBERG VIRUS
Schmallenberg virus, the first European member of the Simbu serogroup of orthobunyaviruses, was discovered in late 2011 near the German/Dutch border. Quickly thereafter, the virus spread throughout much of the continent and the British Isles (see https://flutrackers.com/forum/forum/animal-diseases-of-concern-excludes-h5n1/schmallenberg-virus/124383-schmallenberg-virus-in-europe-map-information-by-country-january-2013). The virus infects domestic and wild ruminants, but there is no evidence that humans are susceptible. Like Akabane virus, Schmallenberg virus is vector-borne, being transmitted principally by Culicoides biting midges (C. obsoletus sensu stricto, C. scoticus, C. chiopterus, C. dewulfi, C. nubeculosus). Infected adult ruminants show either no signs (subclinical infection) or only mild, nonspecific clinical signs such as fever, diarrhea, or reduced milk production for a few days. Of considerably greater significance is the capacity of the virus to cross the placenta to cause premature birth, stillbirth, or the birth of severely malformed offspring after naïve dams are infected during a vulnerable period of gestation.
The outcome of congenital infection of ruminants with Schmallenberg virus mirrors that described for Akabane virus. Congenitally infected lambs or calves may show varying degrees of arthrogryposis, hydranencephaly, torticollis, kyphosis, lordosis, scoliosis, ankylosis, and brachygnathia, the severity of which reflects the gestational age of the fetus at infection (Fig. 22.3). The adverse impact of Schmallenberg virus infection is greater in sheep than in cattle.
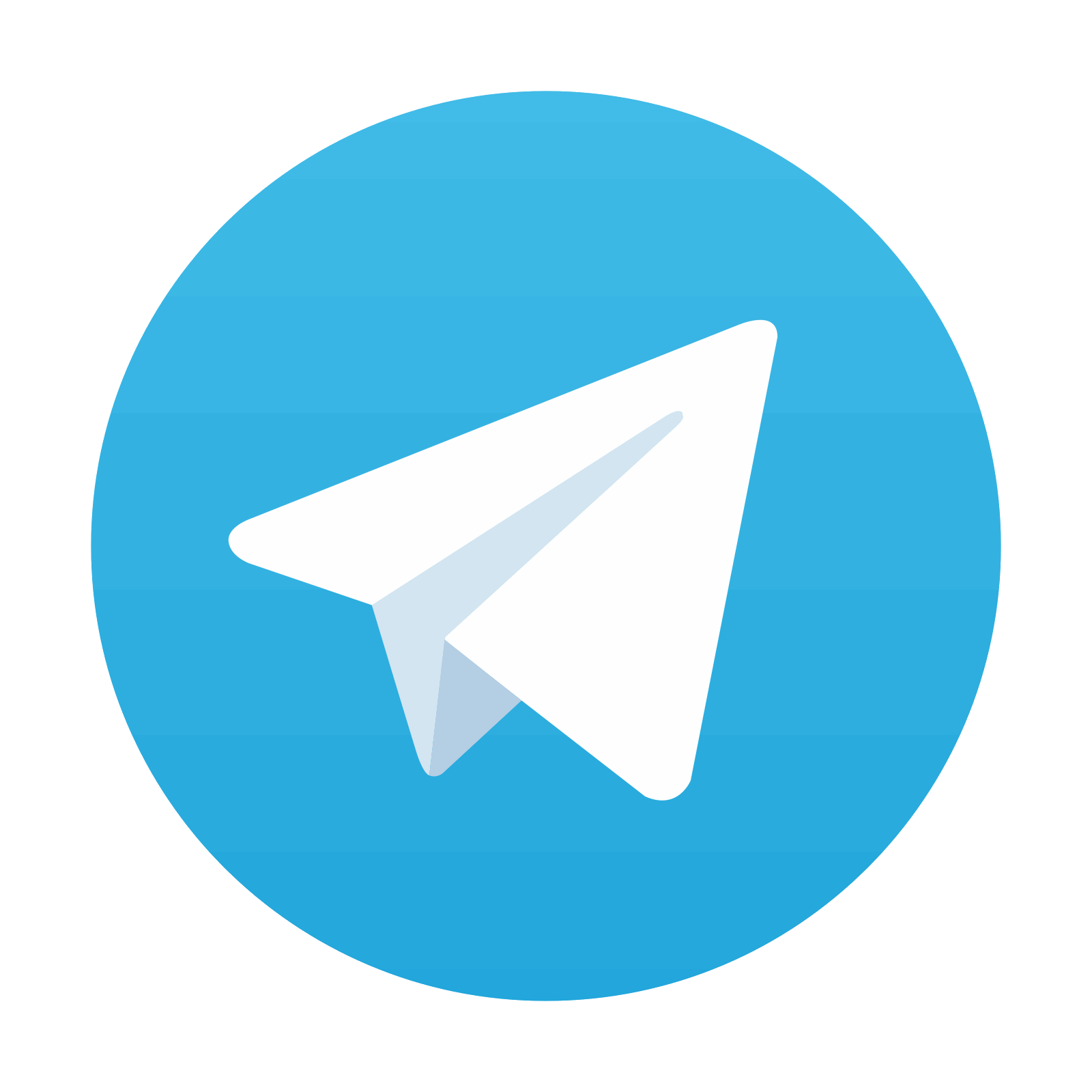
Stay updated, free articles. Join our Telegram channel

Full access? Get Clinical Tree
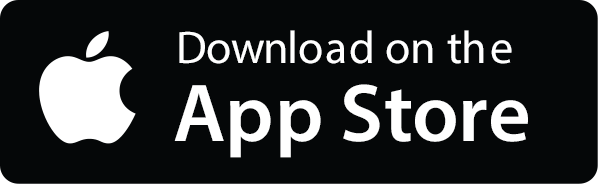
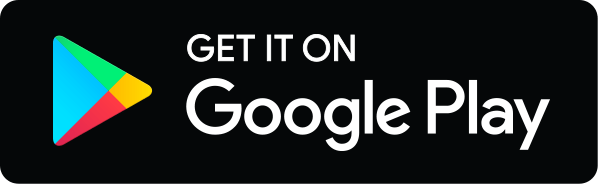