Bornaviridae
Abstract
This chapter describes the properties of bornaviruses and features of the diseases they cause in animals.
Keywords
Bornavirus; Bornaviridae; Mononegavirales; open reading frames; Borna disease virus
Borna disease is named for the town of Borna in Saxony, Germany, where, since at least 1895, devastating epidemics of a naturally occurring, infectious, usually fatal, neurological disease of horses and occasionally sheep have occurred. The viral etiology of the disease was established as early as 1925. Sporadic outbreaks of Borna disease have been described in horses in several European countries, notably Germany, Switzerland, and Austria. There is significant controversy as to whether or not “classical” Borna disease occurs outside Europe. Serological studies suggest that the causative virus, or relatives, has a wide, perhaps global, distribution. In addition to horses and sheep, infection has been reported in other members of the family Equidae, cattle, goats, rabbits, lynx, zoo animals (camelids, sloth, various species of monkeys, hippopotamus) and, rarely, companion animals (dogs, cats). Suggestions that infection of humans with the classical Borna disease virus may be linked to specific neuropsychiatric illnesses have been disproven despite the occurrence of virus-specific antibodies. However, understanding of bornavirus infections has increased considerably during the last decade as the reservoir of the classical Borna disease virus affecting horse and sheep has been identified. Furthermore, a variety of phylogenetically diverse bornaviruses have been detected by molecular methods, including next generation sequencing. Notably, a novel bornavirus has been detected in certain squirrels, and humans who raise these squirrels. Other genetically distinct bornaviruses have been identified in birds, and are incriminated as the cause of an important and fatal disease syndrome designated as proventricular dilatation disease.
Properties of BORNA DISEASE VIRUS
Classification
The member viruses of the family Bornaviridae are included in the order Mononegavirales, along with rhabdoviruses, filoviruses, and paramyxoviruses (see Chapter 17: Paramyxoviridae and Pneumoviridae). The family Bornaviridae contains a single genus Bornavirus, that currently includes at least five distinct virus species: Mammalian 1 bornavirus including classical Borna disease virus and the variant No/98; Psittaciform 1 bornavirus that includes avian/psittacine bornaviruses 1, 2, 3, 4, 7; Passeriform 1 bornavirus including avian/canary bornaviruses C1, C2, C3, and LS; Passeriform 2 bornaviruses including estridild finch bornavirus EF; and Waterbird 1 bornaviruses that include avian bornavirus 062cg. A snake bornavirus, provisionally named as Loveridge’s gartner snake virus 1, is proposed to represent another virus species provisionally named elapid 1 bornavirus; other as yet unclassified viruses in the genus are avian bornaviruses 5, 6, and “MALL,” and an additional reptile virus (Gaboon viper virus, GAVV-1). Some 14 distinct genotypes of avian bornaviruses have been described to date, of which seven have been discovered in nonpsittacine species. Whereas the genome of classical Borna disease virus is highly conserved (nucleotide homology >95%), that of avian bornavirus is less conserved with a sequence identity of approximately 68–85% between genotypes and approximately 60–69% as compared to the classical Borna disease virus. Endogenous bornavirus-like elements similar to the N, M, and L genes have found to be stably integrated into the genome of various vertebrate species including snakes, bats, elephants, fish, lemurs, rodents, squirrels, primates, and humans.
Virion Properties
Borna disease virus virions are spherical, enveloped, approximately 90 nm in diameter and contain a core that is some 50–60 nm in diameter (Fig. 20.1). The genome is a single molecule of negative-sense, single-stranded RNA, approximately 8.9 kb in size that includes at least six open reading frames (Fig. 20.2) with the typical genome organization of viruses in the order Mononegavirales; specifically, 3′-N–P/X–M–G–L-5′. The major structural proteins include the nucleoprotein (N), the phosphoprotein (P), the matrix protein (M), the glycoprotein (G), and the RNA-dependent RNA polymerase (L) protein; a nonstructural small polypeptide (X/p10) that is abundantly expressed in infected cells is absent in virus particles. The virus is sensitive to heat, acid, lipid solvents, and common disinfectants (Table 20.1).
Table 20.1
Properties of Borna Disease Virus
Virions are approximately 90 nm in diameter, enveloped with an inner core, 50–60 nm in diameter
The genome is a single molecule of negative-sense, single-stranded RNA, 8.9 kb in size
The genome has six principal open reading frames encoding at least six proteins, including a nucleoprotein, phosphoprotein, X-protein, matrix protein, glycoprotein, and an RNA-dependent RNA polymerase
Virus transcription and replication take place in the nucleus
Infection in cell culture is characteristically noncytolytic and persistent infection is characteristic of infections of animals and in cell cultures
Virus Replication
Replication of bornaviruses has been characterized principally using the classical Borna disease virus. A broad range of cell types can be infected with this virus, including neurons and glial cells derived from several animal species. The viral G protein mediates attachment, and the virus enters susceptible cells via receptor-mediated endocytosis. The cellular receptor is uncharacterized. The virus uses a noncytolytic replication strategy that results in virus persistence in cultured cells and infected animals. Borna disease virus differs from other members of the order Mononegavirales in that both transcription and replication occur in the cell nucleus. Trimming of the termini of bornavirus RNA appears to mediate persistent infection by avoiding host innate antiviral (interferon) responses that are normally triggered by RIG-I-mediated recognition of triphosphorylated genomic termini (see Chapter 4: Antiviral Immunity and Virus Vaccines).
Borna disease virus is unusual in its transcriptional strategy (Fig. 20.2). The genome of bornaviruses differs from that of other negative-stranded viruses in the configuration of initiation and termination signals, intergenic regions, and overlaps at gene boundaries. The genome is transcribed into six primary transcripts, two of which are modified post-transcriptionally by cellular RNA splicing machinery to yield additional mRNA species. All mRNA species in cells infected with Borna disease virus are polycistronic, except the one encoding the N protein. Several forms of some Borna disease virus proteins are generated during replication, including two isoforms of the N protein, P protein and L protein and three of the G protein, including a full-length N-glycosylated glycoprotein (GP-84/94) and two smaller glycoproteins that represent the N- (GP-N) and C- (GP-C) terminal subunits generated by cleavage by the cellular protease furin. Both GP-C and GP-N are present in virions. The N, P, and L proteins form the polymerase complex. The N, P, and X proteins are all expressed in both the cytoplasm and the nucleus of infected cells. Only low numbers of infected cells express the G protein, in contrast to those expressing N and P. The nonglycosylated M protein mediates virion assembly within the cytoplasm of infected cells.
BORNA DISEASE VIRUS
Clinical Features and Epidemiology
Natural infection with classical Borna disease virus has been described predominantly in horses and sheep in areas of central Europe, but occasional cases have also been reported in other species. Experimentally, the host range is wide, from chickens to primates. For many years, Borna disease was considered sporadically enzootic only in certain areas of central Europe. However, seroepidemiologic investigations have shown that infection in horses is more widespread. Antibodies have been found in approximately 12% of healthy horses in Germany, and a greater prevalence is found in enzootic areas and in stables with affected horses. Although bornavirus-specific antibodies have also been found in horses in Asia, Australia, the Middle East, and the United States, clinically manifest classical Borna disease has not been unambiguously identified outside of central Europe. The great majority of bornavirus infections of mammals are clinically inapparent so that the incidence of disease typically is low, even in enzootic areas. Importantly, however, only genetic sequence data has been described to date for the majority of novel bornaviruses and, with the notable exception of the avian bornaviruses (see below), the clinical impact and epidemiology of these infections remains poorly defined.
The route of natural Borna disease virus infection of horses is uncertain, but oronasal transmission from direct contact has been proposed on the basis of results from experimental infections of laboratory rats. The incubation period is highly variable, ranging from weeks to months, and the course of clinical Borna disease can be acute or protracted. Mortality is high in horses that develop neurological manifestations. Affected horses may initially present with fever and behavioral changes that become increasingly worse, including depression, altered eating behavior, and head pressing. More advanced cases are characterized by profound neurological disturbances, including proprioceptive deficits and abnormal stance, and dysfunction of individual cranial nerves because of involvement of their respective nuclei. Early neurological signs are attributable to dysfunction of the limbic system, whereas, during later stages of the disease, dysfunction of motor systems causing paralysis may predominate. In terminal stages there is anorexia, compulsive circular walking, head tremor and pressing, and convulsions. Ophthalmologic disorders including nystagmus, pupillary reflex dysfunction, and blindness may occur in advanced stages. The course of the disease is variable, 3–20 days approximately, and usually ends in death.
There has been considerable debate, based on the findings of serological and virological investigations, as to whether classical Borna disease virus infection is zoonotic and responsible for human psychiatric disorders. Failure to independently confirm putative instances of zoonotic transmission, coupled with the possibility of cross-contamination, make it exceedingly unlikely that classical Borna disease virus is associated with human disease. Although Borna virus disease-specific antibodies can be present in sera of patients with various psychiatric diseases, they can also been found in clinically healthy people. Recently, however, a novel bornavirus that is genetically distinct from the classical Borna disease virus was detected in variegated squirrels (Sciurus variegatoides) and in humans raising these squirrels who developed fatal encephalitis.
The distinctly seasonal occurrence of Borna disease cases in spring and early summer, as well as its geographically-restricted range, suggest an essential natural reservoir of infection. There is now compelling evidence that small mammals are the natural reservoir host of Borna disease virus as the virus has been detected in the tissues of white-toothed shrews (Crocidura leucodon) in endemic areas of Switzerland and Germany. Moreover, recent data confirm that infected shrews shed infectious virus via various secretions and excretions.
Pathogenesis and Pathology
The laboratory rat has provided the most thoroughly investigated experimental model of Borna disease virus infection. The intranasal route appears most likely to be responsible for natural infections, with virus passing intra-axonally to the olfactory bulbs of the brain from olfactory nerve endings. The virus is then disseminated throughout the central nervous system. In rats, and perhaps other animals, there are substantial differences in pathogenesis, depending on when infection is initiated. Infection of immunotolerant newborn rats results in a persistent infection of the central nervous system, as well as other organs, with minimal tissue injury or neurologic alteration but deficits in learning and memory. In contrast, infection of adult rats of certain strains leads to severe lesions in the central nervous system, and marked behavioral changes. The virus replicates in both neurons and glial cells, and persistent infection is characteristic of Borna disease virus infection.
Borna disease virus infection does not elicit a protective immune response; rather, infection stimulates a T-cell-mediated immunopathologic reaction (a delayed-type hypersensitivity response) that contributes to disease progression. Histologically, this manifests as non suppurative (mononuclear inflammatory cell) meningoencephalitis accompanied by astroglial and microglial activation. Infiltrating cells include CD4+ and CD8+ T cells, macrophages, and B-cells. Experimentally, whereas infection of adult immunocompetent animals regularly results in disease, infection of neonatal or immunocompromised animals leads neither to encephalitis nor to disease, despite the persistence of the virus. Antibodies produced in response to Borna disease virus infection are nonneutralizing, and most likely do not contribute to disease pathogenesis. Adoptive transfer of immunoglobulins from infected animals to immunocompromised recipients does not induce pathological changes or disease whereas adoptive transfer of virus-specific T cells does reproduce the disease. Clinical manifestations of Borna disease likely reflect virus-mediated alterations in cellular signaling pathways and modulation of protein functions, eg, cellular enzymes.
Infection with Borna disease virus in horses and sheep induces a severe encephalomyelitis that principally affects the gray matter (polioencephalomyelitis); histologically, there typically is extensive perivascular cuffing with lymphocytes, macrophages, and plasma cells. Neuronal necrosis is not a feature, but distinctive eosinophilic intranuclear inclusions in neurons, called “Joest-Degen” bodies, are characteristic, even pathognomonic of Borna disease, although they cannot be demonstrated in all cases. Lesions are especially prominent in the gray matter of the olfactory bulb, basal cortex, caudate nucleus, and hippocampus. The retina typically also is involved in experimentally infected laboratory animals.
Diagnosis
Premortem diagnosis of Borna disease is difficult because several diseases can induce similar clinical signs in horses, including rabies, tetanus, equine herpesvirus 1-induced encephalomyelitis, protozoal encephalomyelitis, West Nile and other flaviviral diseases, and the equine alphavirus encephalitides. Diagnosis is usually confirmed by the demonstration of antibodies in the serum and/or, preferably, in the cerebrospinal fluid. This can be done using indirect immunofluorescence staining of persistently infected Madin–Darby canine kidney cells as substrate. Alternative serological tests include western immunoblotting and ELISA. Virus isolation in susceptible cultured cells can be used to confirm bornavirus infection, although RT-PCR and sequence analysis are increasingly used. Immunohistochemical staining of viral antigen is used to confirm postmortem diagnosis of Borna disease in sections of brain with typical histologic lesions. Alternatively, in situ hybridization can be used to detect the presence of bornavirus RNA in tissue sections.
Immunity, Prevention, and Control
Although antiviral therapy has shown some promise in experimental studies, there is currently no specific treatment for animals with classical Borna disease. Similarly, although a number of inactivated, live-attenuated, and recombinant vaccines have been developed and tested for the potential prevention of Borna disease virus infection, there are concerns regarding their safety and efficacy and none are yet licensed or commercially available. Although direct (horse–horse) contact transmission of Borna disease virus has not been clearly demonstrated, the identification and quarantine of carrier animals is potentially important to limit spread of the virus. Given the recent identification of novel bornaviruses in a wide variety of animal species, there is a considerable need for further studies to better characterize the epidemiology of these infections and their potential clinical importance.
Avian BORNAVIRUS
Clinical Features and Epidemiology
Avian bornavirus is the apparent causative agent of proventricular dilation disease, a progressive and devastating neurological and gastrointestinal disease of birds that was first recognized in the 1970s among macaws exported from Bolivia (syn. macaw wasting disease). Although the overwhelming majority of cases of proventricular dilation disease occur in psittacine birds (parrots), the disease has now been identified in approximately 80 species of birds including nonpsittacine species. Affected birds present with signs of progressive neurological and/or gastrointestinal tract dysfunction, including weight loss, dysphagia, regurgitation, ataxia, and proprioceptive deficits. The disease is usually fatal but subclinical infections potentially result in birds that continuously or intermittently shed the virus. Sudden death may also occur. At least 14 different genotypes of avian bornavirus have now been detected in pet and wild birds, including both psittacine and nonpsittacine species such as songbirds [canaries (genera Serinus and Crithagra), finches (family Fringillidae)] and waterfowl, ie, swans (genus Cygnus), geese, and ducks. Avian bornavirus infection apparently occurs in birds worldwide, and particular genotypes of avian bornavirus are more common than others.
The epidemiology of avian bornavirus infections is poorly characterized, including the natural reservoir(s) of infection and routes of virus transmission. The incubation period in experimentally infected birds has ranged from 20 to 200 days after inoculation. Bornavirus RNA can be detected in the feces and cloacal and crop contents of naturally infected birds, suggesting that direct horizontal transmission of the virus might be important. However, co-housing of infected and susceptible birds does not consistently lead to virus transmission and efforts to experimentally infect susceptible birds by oronasal inoculation of virus have been unsuccessful to date. Similarly, the role of vertical transmission of virus is uncertain.
Pathogenesis and Pathology
Experimental avian bornavirus infections of cockatiels and canaries have provided much information on the virus and its pathogenesis, although canaries do not typically develop the proventricular dilation disease syndrome that occurs in cockatiels. To date, most experimental studies have been done using a single virus genotype (avian bornavirus 4). Depending on the route of infection (intracerebral, intramuscular, intravenous), inoculated birds develop virus-specific antibodies between approximately 7 and 60 days after infection, and bornavirus RNA is present within the feces of inoculated birds between 20 and 70 days after infection. Naturally infected birds with high viral loads and with high bornavirus-specific antibody titers are more likely to develop clinical proventricular dilation disease. As occurs in bornavirus-infected mammals, avian bornavirus-specific antibodies are not protective in birds. The correlation of clinical signs, gross and histological lesions, and detection of viral RNA and antigen vary between individuals so that subclinically infected birds can have typical histological lesions and either restricted (eg, central nervous system only) or widespread distribution of bornavirus antigens.
Typical necropsy findings of birds with proventricular dilation disease include emaciation with atrophy of pectoral muscles and dilatation of the crop, proventriculus, ventriculus, and small intestine. Histologic changes include mononuclear (lymphocyte, macrophages and plasma cells) inflammation within both the central and peripheral nervous systems (ie, lymphocytic encephalitis, myelitis, ganglionitis, and neuritis and combinations thereof). Involvement of the gastrointestinal autonomic nervous system is responsible for signs of gastrointestinal dysfunction, including paralytic dilatation of the esophagus and proventriculus. Inflammation also may involve the myocardium and cardiac conduction system, the adrenal glands, and eyes.
Like mammalian bornaviruses, avian bornaviruses are neurotropic but also show affinity to the gastrointestinal tract and peripheral organs. The role of immunopathologic mechanisms of cellular injury in mediating disease expression in bornavirus-infected birds remains to be characterized. Clearly, occurrence of disease varies markedly between bird species and between individuals.
Diagnosis
Antemortem diagnosis of proventricular dilation disease can be difficult as affected birds manifest similar signs to those with other gastrointestinal diseases caused by, amongst others, foreign bodies, intoxications, neoplasia, and various other infectious diseases. Further complicating the diagnosis of avian bornavirus infection is the intermittent presence of viral RNA in the feces of infected birds. The diagnosis of proventricular dilation disease is definitively confirmed by histopathological evaluation and immunohistochemical staining of biopsy species from the upper gastrointestinal tract. The characteristic lesion is lymphocytic ganglioneuritis (lymphocytic inflammation affecting the autonomic ganglia) (Fig. 20.3) with prominent immunohistochemical staining of viral antigen in affected neurons (Fig. 20.4).
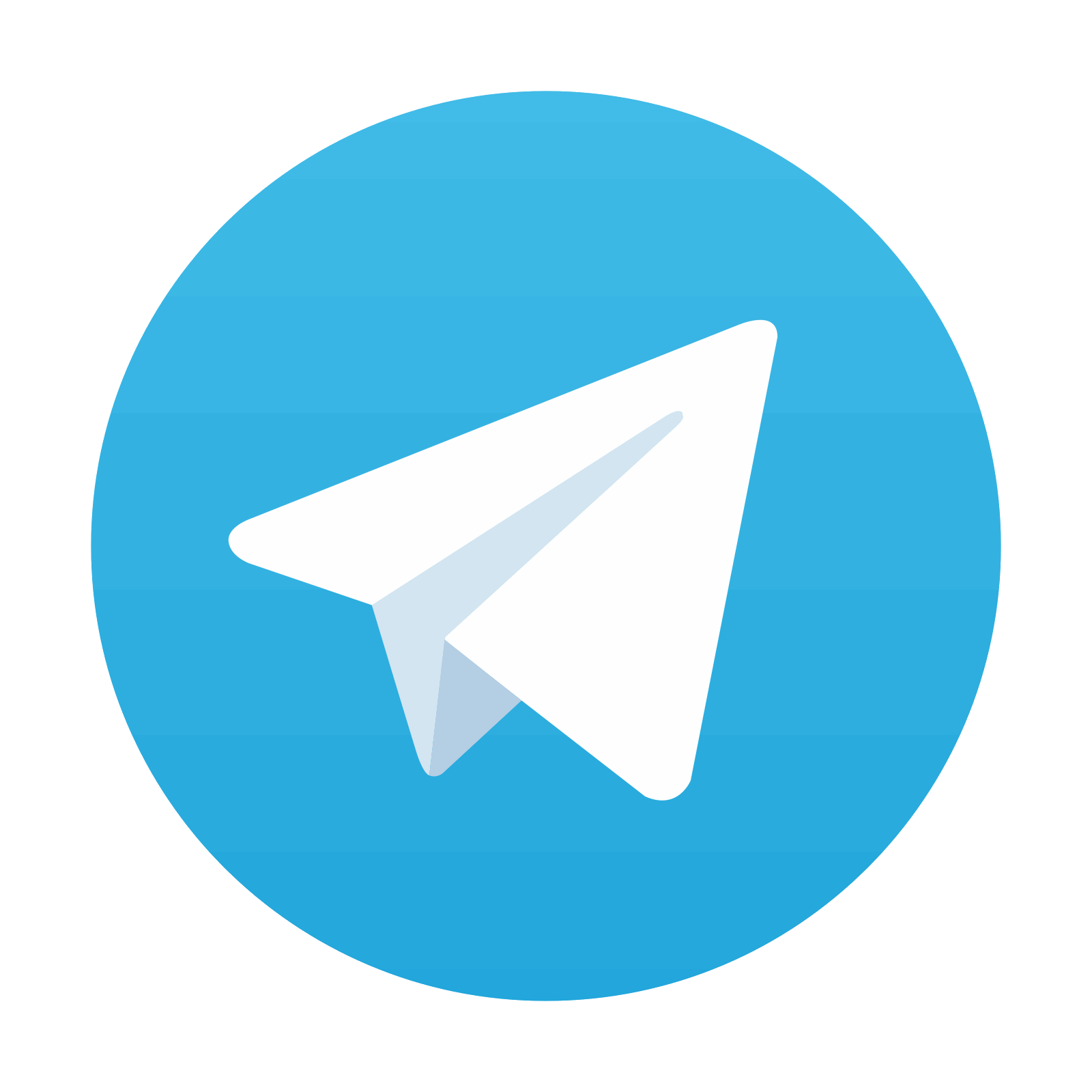
Stay updated, free articles. Join our Telegram channel

Full access? Get Clinical Tree
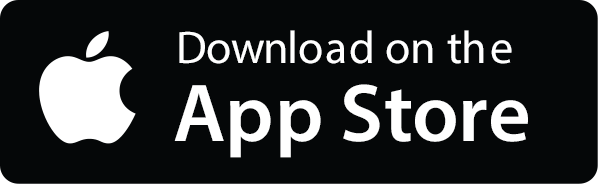
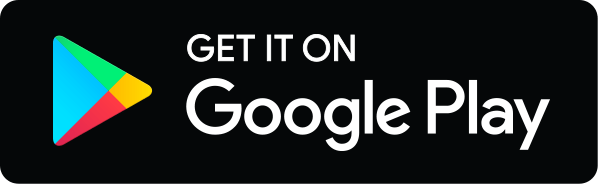